- 1College of Advanced Interdisciplinary Studies, National University of Defense Technology, Changsha, China
- 2Nanhu Laser Laboratory, National University of Defense Technology, Changsha, China
- 3Hunan Provincial Key Laboratory of High Energy Laser Technology, Changsha, China
The spectra of narrow linewidth fiber amplifiers are closely related to both the stimulated Brillouin scattering (SBS) threshold in power scaling process and the combining efficiency in coherent beam combining (CBC) system. In this manuscript, the SBS thresholds of fiber amplifier with different spectral distributions (Gaussian, sinc2 and rectangular) have been compared under the same spectral complex degree of coherence (CDC), which could promise the same combining efficiency in CBC system. A SBS dynamic model is established to analyze the SBS process in fiber amplifier and a comparing experiment is also performed by measuring the SBS thresholds of different spectra that have the same CDC set to be 0.96. The FWHM linewidths of Gaussian, sinc2 and rectangular spectra are adjusted to be 1.1 GHz, 0.5 GHz and 1.06 GHz, respectively. The corresponding SBS thresholds are measured to be 108 W, 77 W, and 135 W. By contrast, the rectangular spectra could have most excellent capacity on improving SBS threshold in fiber amplifier under the same combining efficiency in CBC system. Overall, it could provide a feasible method on spectra designing in high power narrow linewidth fiber amplifiers used in CBC system.
Introduction
High power narrow linewidth fiber amplifiers based on master oscillator power amplifier (MOPA) architecture are usually acted as the laser sources in coherent beam combining (CBC) system to break the power limitation of monolithic fiber amplifier [1–4]. For achieving higher power output, the power capacity of each combinable fiber amplifier and the combining efficiency in CBC system are the two main factors to be considered. Normally, fiber amplifiers with narrower linewidth could enable better combining efficiency and wavefront predistortion in the presence of path length mismatch in CBC system [5–7]. However, narrow linewidth operation would limit the output power of each combinable fiber amplifier due to stimulated Brillouin scattering (SBS) effect [8–12] and transverse mode instability (TMI) effect [13]. Therefore, it should balance this trade-off between the output power and combining efficiency of fiber amplifiers used in CBC system. The key point could be concentrated on the spectra of coherently combined fiber amplifiers.
As we know, in high power narrow linewidth fiber amplifiers with MOPA structure, a single frequency seed laser could be phase-modulated to broaden the spectral linewidth for SBS suppression. To date, several modulating signals have been proposed, including sine-wave signal [14, 15], white noise signal (WNS) [16–18], pseudo-random bit sequence (PRBS) signal [19–22], multi-phase coded signal (MPCS) [23], multi-objective nonlinear optimized signal (MONOS) [24], piecewise parabolic signal (PPS) [25] and other special modulating signals [26–30]. Among of them, WNS and PRBS modulation have been usually applied on high power narrow linewidth fiber amplifiers on experiment. For example, by using WNS modulation, a 960 W polarization-maintained (PM) fiber amplifier with Gaussian spectrum could be obtained and the FWHM linewidth is about 6.5 GHz [18]. PRBS modulation could be used to generate sinc2 spectra and has been applied on fiber amplifiers to obtain output power over 1 kW and FWHM linewidth under 3 GHz [19, 20]. Besides, fiber amplifiers with MONOS or PPS modulation would be equipped with rectangular spectra to achieve high SBS threshold [24, 25]. However, such two modulating techniques have not been realized on experiment according to the public reports.
As we can see, there are so many modulating signals could be selected for SBS suppressing. However, detailed studies comparing the SBS threshold, in the presence of the same combining efficiency in CBC system, have yet to be reported. In the previous research, the beam combining performance of two 150 W fiber amplifiers modulated by PRBS and WNS respectively have been compared by measuring the visibility as a function of path length mismatch [31]. It indicates that the optimized PRBS modulation performs better due to its recoherence effect. However, the SBS thresholds of PRBS and WNS modulation schemes have not been compared under the same visibility in CBC system.
In our previous work, it was found that the spectral complex degree of coherence (CDC) of combinable fiber amplifier was in direct proportion to the combining efficiency in CBC system in the presence of determined path length mismatch (or delay time) [32]. Additionally, the CDC of fiber amplifiers could be calculated from the measured spectra. Due to that the spectral linewidths have different definitions including FWHM linewidth [15, 16], power ration linewidth [25] and RMS linewidth [24], the spectral CDC could act as a unified criterion to evaluate the combining efficiency in CBC system. Therefore, by adjusting the modulating signals to obtain the same spectral CDC, the SBS threshold of fiber amplifiers with different spectral distributions could be compared under the same combining efficiency in CBC system.
In this paper, fiber amplifiers with Gaussian, sinc2 and rectangular spectra were designed to have the same spectral CDC. The SBS thresholds have been calculated firstly based on a SBS dynamical model. Then a high power fiber amplifier system with MOPA structure was established to compare the SBS thresholds of different spectra. By adjusting the modulating parameters to promise identical spectral CDC (and hence combining efficiency), it was found that the rectangular spectra obtained by PPS modulation performed best on suppressing SBS effect in fiber amplifiers.
Theoretical simulation
Firstly, as proved in previous research [32], just considering the influence of spectral characteristics of each combinable fiber amplifier on the combining efficiency in CBC system, the relationship between the combining efficiency (η) and the CDC (γ(τ)) could be described as.
Equation. 1 indicates that the combining efficiency is directly proportional to the real part of CDC. In other words, the higher spectral CDC of coherently combined fiber amplifiers would present more excellent combining performance in CBC system. Besides, the spectral CDC could be described as [32].
Where τ is the delay time between the combined fiber amplifiers in CBC system, ν is the frequency of signal laser and ξ(ν) is the normalized power spectrum. Eq. 2 means that the CDC value of combined fiber amplifiers could be calculated from the spectra when the delay time in CBC system is determined. Then the combining performance of fiber amplifiers can be evaluated from Eq. 1. To make it convenient for analysis and without losing generality, the delay time is set to be 0.1 ns here. The corresponding optical path difference is about 3 cm which is common in CBC system over long-distance propagation [33]. More importantly, when high combining efficiency is needed at this delay time, the FWHM linewidth would be required to be GHz level that have significant application in CBC system [1, 2].
It is worth noting that the same spectral CDC value does not mean the same FWHM linewidth for different spectral distributions. The latter is usually applied to evaluate the spectral coherence of combined fiber amplifiers in CBC system. Figure 1A shows three types of spectra with perfect rectangular, Gaussian and sinc2 shapes. As for different spectral distributions, the FWHM linewidths under different CDC values could be calculated according to Eq. 2. As we can see, when the spectral CDC is required to be identical, the rectangular spectrum would have wider FWHM linewidth. And when the CDC value is above 0.6 at 0.1 ns delay time, the FWHM linewidth of Gaussian spectrum is larger than that of sinc2 spectrum.
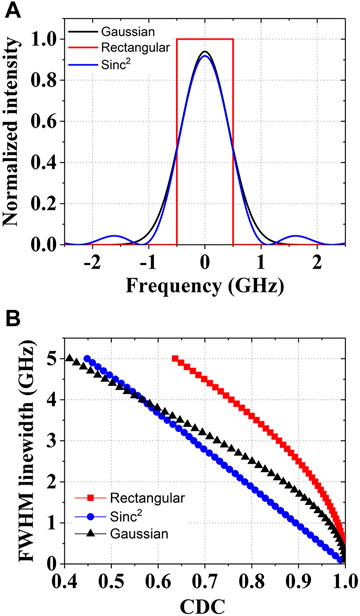
FIGURE 1. (A) theoretical spectra with rectangular, Gaussian and sinc2 shapes, (B) the FWHM linewidths under different CDC values at 0.1 ns delay time.
As a typical application of CDC in CBC system, the SBS thresholds of combinable fiber amplifiers with different spectral distributions could be compared under the same spectral CDC value. Firstly, a theoretical model of simulating the SBS dynamic process in fiber amplifier is introduced. It includes a series of triply coupled partial differential equations that present the three-wave interaction of signal laser field, Stokes field and acoustic phonon field [34, 35]. In this simulation, all fields in fiber amplifier are regarded as time-harmonic monochromatic plane waves and satisfy the following coupling equations [36].
In these equations, AS, AB and Q represent the normalized amplitudes of oscillations of the signal laser, Stokes, and acoustic fields, respectively. νgs and νgB are the group speed of signal laser and Stokes light, νA is the acoustic velocity, κ1S, κ1B and κ2 are coupling coefficients of the signal laser, Stokes, and acoustic fields, respectively. αS and αB are the attenuation of signal laser and Stokes light in active fiber, γs is the nonlinear coefficient of signal laser, gs and gB are the active gain of signal and Stokes light, which can be expressed as
Here the active gain of signal and Stokes light is considered to be identical due to the similar wavelength. σas and σes are the absorption cross-section and emission cross-section of signal laser in active fiber, N and N2 represent the number of doped Yb3+ particles and excited Yb3+ particles at upper-energy state.
In Eq. 5, ΓB is the acoustic damping ratio and Aao is the effective interaction area of the light field and the acoustic field, f is the ignition of the SBS process from a Langevin noise source. It is a Gaussian random variable with an average value of zero and obeys the following equation [37].
Where NQ represent the strength of fluctuations. It can be determined according to thermodynamics principle.
Where k is Boltzmann Constant and ρ0 is the density of fiber, T0 is the temperature and Aeff is the effective mode area in the fiber. Besides, the pumping light of amplifier and the number of Yb3+ particles at upper-energy state can be calculated according to the rate equations in fiber amplifier.
Where PS, PB and PP represent the signal laser power, Stokes power and pump power, ΓS and ΓP are the overlapping factors with doped area of signal light and pump light, respectively. σap and σep are absorption cross-section and emission cross-section of pump light in active fiber. νgs is the group speed of pump light, τ is the average lifetime of Yb3+ in upper-energy state, c is the speed of light in vacuum, h is Planck Constant and Ac is the doped area.
In the main amplifier, a signal laser with power of 10 W is injected into an active 20/400 μm Yb-doped fiber with a length of 10 m. The absorption efficient of active fiber is set to be 1.5 dB/m at 976 nm pumping laser. Besides, considering the practical experiment structure, 3 m delivery passive fiber follows behind the amplifier in the simulation. The other parameters are corresponding to that in [36].
Based on the theoretical model above, we compare the SBS suppression effect of three typical modulation schemes including PRBS modulation, WNS modulation and PPS modulation, which could generate spectra with sinc2, Gaussian and rectangular shapes, respectively. By adjusting the modulating frequency and amplitude, the spectral CDC of spectral distribution is set to be 0.96 at 0.1 ns delay time. The principle of PRBS and WNS has been introduced in [31] and the PPS modulation has been analyzed in detail in [25]. Figures 2A, C, E show the modulating amplitude of WNS, PRBS, and PPS modulation on time domain, respectively. And the corresponding Gaussian, sinc2 and rectangular spectra are shown in Figures 2B, D, F. In this simulation, the PRBS is generated by selecting 27–1 pattern which is proved to have better SBS suppressing ability [31]. The FWHM linewidths are calculated to be 1.45 GHz in rectangular spectrum modulated by PPS, 0.94 GHz in Gaussian spectrum modulated by WNS and 0.5 GHz in sinc2 spectrum modulated by PRBS, respectively.
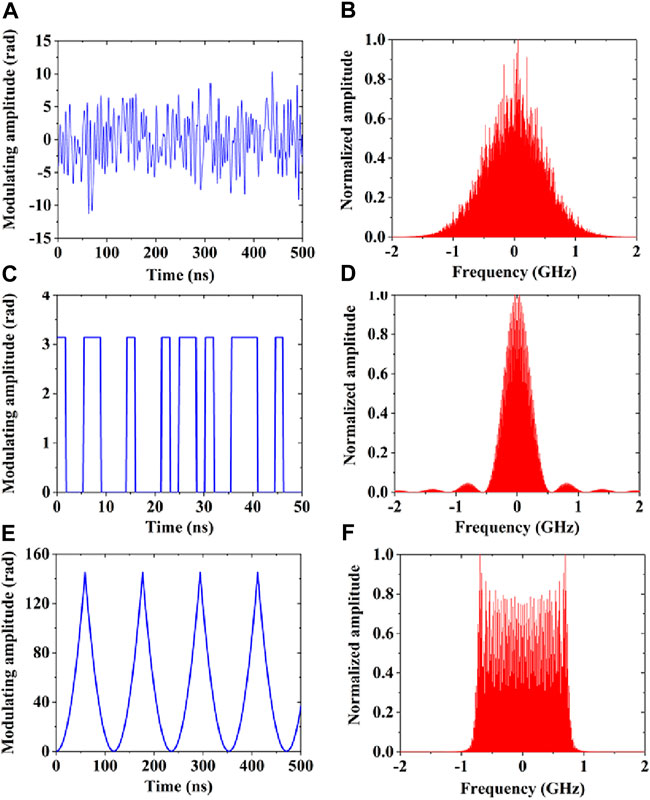
FIGURE 2. (A) WNS, (B) Gaussian spectrum modulated by WNS, (C) PRBS, (D) sinc2 spectrum modulated by PRBS, (E) PPS, (F) rectangular spectrum modulated by PPS.
Figure 3 shows the trend of reflectivity with increasing output power. The reflectivity is defined as the ratio of backward power to output power. When SBS occurs, the reflectivity will increase nonlinearly because the energy would be transferred from the signal light to the backward stokes light [34, 36]. The threshold is marked with a red dotted line where the reflectivity reaches to 0.02% and increases dramatically with the output power. From Figure 3, the calculated SBS threshold of fiber amplifier with rectangular spectrum is about 136 W, which is higher than those of the Gaussian spectrum (108 W) and sinc2 spectrum (59 W). Thus, it could be concluded that the rectangular spectrum has more positive effect on SBS suppression than Gaussian and sinc2 spectrum in the circumstance of identical CDC value.
Further, we calculate the SBS thresholds of such three types of spectra when the CDC varies from 0.82 to 0.96 at 0.1 ns delay time. Each spectrum has a FWHM linewidth below 5 GHz in the varying process according to Figure 1. The dependence of SBS threshold on spectral CDC are shown in Figure 4. When the spectral CDC increases, the FWHM linewidth of spectra would be narrowed. Therefore, the SBS threshold in fiber amplifier would decrease. It is obvious that rectangular spectrum always presents best performance on suppressing SBS at any CDC values from 0.82 to 0.96. Besides, Gaussian spectrum has higher SBS threshold than sinc2 spectrum at the same spectral CDC value. Therefore, the fiber amplifier with rectangular spectrum could present excellent coherent combining capacity in CBC system due to the highest SBS threshold in the case of determined CDC value.
Experimental demonstration
To further investigate the SBS suppressing ability of different spectral distributions, a typical fiber amplifier system with MOPA structure was established (as shown in Figure 5). Specifically, the seed laser was a 1064.4 nm single frequency, linear-polarized fiber oscillator with ultra-short cavity and output power of 40 mW [38]. It was modulated by an electro-optic phase modulator with bandwidth of 10 GHz and half-wave voltage of 5 V, which was driven by a series of modulating voltage signals. Here a white noise source was filtered to generate the WNS and an arbitrary waveform generator (AWG) was programmed to generate PRBS and PPS. The generated modulating signals were amplified by radio-frequency amplifier (RF Amp) and then were applied on the phase modulator. Next, the modulated signal laser was injected to the pre-amplifier and was boosted to about 10 W. A 99.9:0.1 fiber coupler was used for transferring the pre-amplified signal laser to the main amplifier and monitoring the backlight from the main amplifier to judge the SBS threshold. In the main amplifier, a 10 m Yb-doped active fiber with core/cladding diameters of 20/400 μm was employed as the gain fiber. At the end of the main amplifier, a collimator was installed to send the laser beam to free space. All the devices in fiber system were polarization maintained. The output laser beam was split by a high-reflectance (99.9:0.1) mirror (HRM). The main part of output laser was recorded by a power meter and the small part was used for spectrum monitoring.
In this experiment, a Fabry-Perot interferometer (FPI) with a free spectral range (FSR) of 4 GHz and optical spectral resolution of 8 MHz was used to measure the output spectrum at the spectrum monitor port. Then the spectral CDC value could be calculated from the measured spectrum according to Eq. 2 [32]. As we described above, the signal laser modulated by PPS, PRBS and WNS could have rectangular, sinc2 and Gaussian spectra, respectively. By adjusting the output voltage and operating frequency of AWG and controlling the attenuation of WNS source and the filtered frequency, the modulating frequency and amplitude of PPS, PRBS and WNS could be tunable. Then the spectra of signal laser and the corresponding spectral CDC values could be controlled. It was worth mentioning that the modulating amplitude of PPS in simulation was too large to be achieved on experiment. Therefore, the amplitude of PPS was divided by 2π and the remainder was leaved as the new modulating amplitude which would not exceed 2π.
In order to be identical with the simulation, the CDC of each spectrum on experiment was controlled to be 0.96 at 0.1 ns delay time. In this situation, Table 1 showed the experimental parameters of different modulating techniques and the corresponding FWHM linewidths of output laser. The spectra of output laser were also shown in Figure 6. We could see that the FWHM linewidths of output laser were measured to be 1.06 GHz in rectangular spectrum, 1.1 GHz in Gaussian spectrum and 0.5 GHz in sinc2 spectrum, respectively. The experimental results of Gaussian and sinc2 spectra agreed well with those in simulation. The rectangular spectrum on experiment with FWHM linewidth of 1.06 GHz was obviously smaller than that in simulation which was as broad as 1.45 GHz. It was mainly due to that the top of generated rectangular spectrum on experiment were not smooth enough.

TABLE 1. The experimental parameters when the CDC value is controlled to be 0.96 at 0.1 ns delay time.
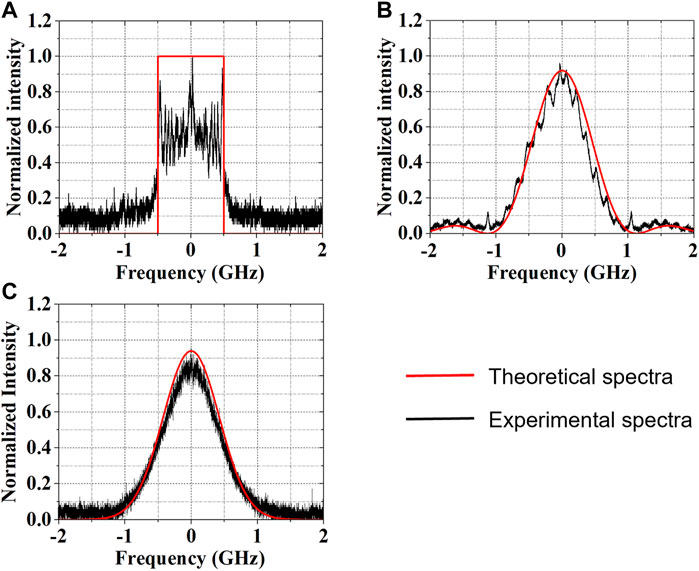
FIGURE 6. The experimental spectra of different modulating techniques (A) PPS with rectangular spectrum, (B) PRBS with sinc2 spectrum, (C) WNS with Gaussian spectrum.
As the output power increased, the varying trend of power reflectivity was also measured and shown in Figure 7. The SBS threshold was defined as the output power where the reflectivity reached up to 0.02% and increased nonlinearly (as the dotted-line indicates in Figure 7), which was also identical with that in simulation. As we can see, the SBS threshold of fiber amplifier was about 135 W with rectangular spectrum, 108 W with Gaussian spectrum and 77 W with sinc2 spectrum, respectively. Thus, it could be concluded that the rectangular spectrum could suppress SBS more effectively than Gaussian and sinc2 spectra at the CDC value of 0.96.
Conclusion
In summary, the SBS process in high power fiber amplifier is simulated when typical phase modulation techniques including PPS, PRBS and WNS are applied. The generated spectra could have rectangular, sinc2 and Gaussian envelopes, respectively. By setting the spectral CDC value to be the same, the SBS threshold of such three types of spectra are calculated and compared. Then a high power fiber amplifier system with MOPA structure is established to measure the SBS threshold of these spectra for experimental demonstration. Both the theoretical and experimental results indicate that the rectangular spectrum has highest SBS threshold under the same spectral CDC. Due to that the spectral CDC is in direct proportion with the combining efficiency in CBC system, it could provide an effective method to select the modulating techniques and design the corresponding spectra of high power narrow linewidth fiber amplifier applied in CBC system.
Data availability statement
The original contributions presented in the study are included in the article/supplementary material, further inquiries can be directed to the corresponding author.
Author contributions
All authors listed have made a substantial, direct, and intellectual contribution to the study and approved it for publication.
Funding
This work was supported by the National Natural Science Foundation of China (61705264, 62035015), Hunan Provincial Innovation Construct Project (2019RS3017, 2019RS2018), the Natural Science Foundation of Hunan province, China (2019JJ10005).
Conflict of interest
The authors declare that the research was conducted in the absence of any commercial or financial relationships that could be construed as a potential conflict of interest.
Publisher’s note
All claims expressed in this article are solely those of the authors and do not necessarily represent those of their affiliated organizations, or those of the publisher, the editors and the reviewers. Any product that may be evaluated in this article, or claim that may be made by its manufacturer, is not guaranteed or endorsed by the publisher.
References
1. Fan TY. Laser beam combining for high-power, high-radiance sources. IEEE J Sel Top Quan Electron (2005) 11:567–77. doi:10.1109/JSTQE.2005.850241
2. Liu Z, Ma P, Su R, Tao R, Ma Y, Wang X, et al. High-power coherent beam polarization combination of fiber lasers: Progress and prospect [invited]. J Opt Soc Am B (2017) 34:A7–A14. doi:10.1364/JOSAB.34.0000A7
3. Flores A, Dajani I, Holten R, Ehrenreich T, Anderson B. Multi-kilowatt diffractive coherent combining of pseudorandom modulated fiber amplifiers. Opt Eng (2016) 55:096101. doi:10.1117/1.OE.55.9.096101
4. Ma P, Chang H, Ma Y, Su R, Qi Y, Wu J, et al. 7.1 kW coherent beam combining system based on a seven-channel fiber amplifier array. Opt Laser Technol (2021) 140:107016. doi:10.1016/j.optlastec.2021.107016
5. Goodno GD, Shih C-C, Rothenberg JE. Perturbative analysis of coherent combining efficiency with mismatched lasers. Opt Express (2010) 18:25403–14. doi:10.1364/OE.18.025403
6. Ma P, Wang X, Ma Y, Zhou P, Liu Z. Analysis of multi-wavelength active coherent polarization beam combining system. Opt Express (2014) 22:16538–51. doi:10.1364/OE.22.016538
7. Leshchenko VE. Coherent combining efficiency in tiled and filled aperture approaches. Opt Express (2015) 23:15944–70. doi:10.1364/OE.23.015944
8. Dawson JW, Messerly MJ, Beach RJ, Shverdin MY, Stappaerts EA, Sridharan AK, et al. Analysis of the scalability of diffraction-limited fiber lasers and amplifiers to high average power. Opt Express (2008) 16:13240–66. doi:10.1364/OE.16.013240
9. Otto H, Jauregui C, Limpert J, et al. Average power limit of Ytterbium-doped fiber-laser systems with nearly diffraction-limited beam quality. Proc SPIE (2015) 97280E. doi:10.1117/12.2208670
10. Ma P, Xiao H, Liu W, Zhang H, Wang X, Leng J, et al. All-fiberized and narrow-linewidth 5 kW power-level fiber amplifier based on a bidirectional pumping configuration. High Pow Laser Sci Eng (2021) 9:e45. doi:10.1017/hpl.2021.32
11. Liu W, Song J, Ma P, Xiao H, Zhou P. Effects of background spectral noise in the phase-modulated single-frequency seed laser on high-power narrow-linewidth fiber amplifiers. Photon Res (2021) 9:424–31. doi:10.1364/PRJ.414223
12. Liu A, Chen X, Li M, Wang J, Walton DT, Zenteno LA. Comprehensive modeling of single frequency fiber amplifiers for mitigating stimulated Brillouin scattering. J Lightwave Technol (2009) 27:2189–98. doi:10.1109/JLT.2008.2005427
13. Ren S, Lai W, Wang G, Li W, Song J, Chen Y, et al. Experimental study on the impact of signal bandwidth on the transverse mode instability threshold of fiber amplifiers. Opt Express (2022) 30:7845–53. doi:10.1364/OE.454189
14. Engin D, Lu W, Akbulut M, McIntosh B, Verdun H, Gupta S. 1 kW cw Yb-fiber-amplifier with <0.5 GHz linewidth and near-diffraction limited beam quality, for coherent combining application. SPIE Proc (2011) 791407. doi:10.1117/12.875701
15. Ma P, Tao R, Su R, Wang X, Zhou P, Liu Z. 1.89 kW all-fiberized and polarization-maintained amplifiers with narrow linewidth and near-diffraction-limited beam quality. Opt Express (2016) 24:4187–95. doi:10.1364/OE.24.004187
16. Chu Q, Shu Q, Liu Y, Tao R, Yan D, Lin H, et al. 3 kW high OSNR 1030 nm single-mode monolithic fiber amplifier with a 180 pm linewidth. Opt Lett (2021) 45:6502–5. doi:10.1364/OL.405386
17. Chang Z, Wang Y, Sun Y, Peng W, Ke W, Ma Y, et al. 1.5 kW polarization-maintained Yb-doped amplifier with 13 GHz linewidth by suppressing the self-pulsing and stimulated Brillouin scattering. Appl Opt (2019) 58:6419–25. doi:10.1364/AO.58.006419
18. Wang Y, Feng Y, Wang X, Yan H, Peng J, Peng W, et al. 6.5 GHz linearly polarized kilowatt fiber amplifier based on active polarization control. Appl Opt (2017) 56:2760–5. doi:10.1364/AO.56.002760
19. Flores A, Robin C, Lanari A, Dajani I. Pseudo-random binary sequence phase modulation for narrow linewidth, kilowatt, monolithic fiber amplifiers. Opt Express (2014) 22:17735–44. doi:10.1364/OE.22.017735
20. Liu M, Yang Y, Shen H, Zhang J, Zou X, Wang H, et al. 1.27 kW, 2.2 GHz pseudo-random binary sequence phase modulated fiber amplifier with Brillouin gain-spectrum overlap. Sci Rep (2020) 10:629. doi:10.1038/s41598-019-57408-5
21. Jun C, Jung M, Shin W, Yu BA, Yoon YS, Park Y, et al. 818 W Yb-doped amplifier with <7 GHz linewidth based on pseudo-random phase modulation in polarization-maintained all-fiber configuration. Laser Phys Lett (2019) 16:015102. doi:10.1088/1612-202X/aaee11
22. Lim WYW, Seah KW, Seah CP, Tan BS. Wavelength flexible, kW-level narrow linewidth fibre laser based on 7 GHz PRBS phase modulation. Proc SPIE (2020) 1126006. doi:10.1117/12.2547482
23. Lai W, Ma P, Liu W, Li C, Su R, Ma Y, et al. Seeding high brightness fiber amplifiers with multi-phase coded signal modulation for SBS effect management. IEEE Access (2020) 8:127682–9. doi:10.1109/ACCESS.2020.3007413
24. Harish AV, Nilsson J. Optimization of phase modulation formats for suppression of stimulated Brillouin scattering in optical fibers. IEEE J Sel Top Quan Electron (2018) 24:1–10. doi:10.1109/JSTQE.2017.2753041
25. White JO, Young JT, Wei C, Hu J, Menyuk CR. Seeding fiber amplifiers with piecewise parabolic phase modulation for high SBS thresholds and compact spectra. Opt Express (2019) 27:2962–74. doi:10.1364/OE.27.002962
26. Panbiharwala Y, Harish AV, Feng Y, Venkitesh D, Nilsson J, Srinivasan B. Stimulated Brillouin scattering mitigation using optimized phase modulation waveforms in high power narrow linewidth Yb-doped fiber amplifiers. Opt Express (2021) 29:17183–200. doi:10.1364/OE.425159
27. Prakash R, Vikram BS, Supradeepa VR. Enhancing the efficacy of noise modulation for SBS suppression in high power, narrow linewidth fiber lasers by the incorporation of sinusoidal modulation. IEEE Photon J (2021) 13:1–6. doi:10.1109/JPHOT.2021.3111207
28. Yang Y, Li B, Liu M, Huang X, Feng Y, Cheng D, et al. Optimization and visualization of phase modulation with filtered and amplified maximal-length sequence for SBS suppression in a short fiber system: A theoretical treatment. Opt Express (2021) 29:16781–803. doi:10.1364/OE.426070
29. Goodno GD, Rothenberg JE. Suppression of stimulated Brillouin scattering in high power fibers using nonlinear phase demodulation. Opt Express (2019) 27:13129–41. doi:10.1364/OE.27.013129
30. Harish AV, Nilsson J. Suppression of stimulated Brillouin scattering in single-frequency fiber Raman amplifier through pump modulation. J Lightwave Technol (2019) 37:3280–9. doi:10.1109/JLT.2019.2914081
31. Anderson B, Flores A, Holten R, Dajani I. Comparison of phase modulation schemes for coherently combined fiber amplifiers. Opt Express (2015) 23:27046–60. doi:10.1364/OE.23.027046
32. Lai W, Ma P, Liu W, Su R, Ma Y, Li C, et al. Evaluating spectral characteristics of narrow-linewidth fiber lasers in coherent beam combining system via using CDC metric. Opt Lasers Eng (2022) 149:106826. doi:10.1016/j.optlaseng.2021.106826
33. Vorontsov MA, Weyrauch T. High-power lasers for directed-energy applications: Comment. Appl Opt (2016) 55:9950–3. doi:10.1364/AO.55.009950
34. Zeringue C, Dajani I, Naderi S, Moore GT, Robin C. A theoretical study of transient stimulated Brillouin scattering in optical fibers seeded with phase-modulated light. Opt Express (2012) 20:21196–213. doi:10.1364/OE.20.021196
35. Jenkins RB, Sova RM, Joseph RI. Steady-state noise analysis of spontaneous and stimulated Brillouin scattering in optical fibers. J Lightwave Technol (2007) 25:763–70. doi:10.1109/JLT.2006.889673
36. Ran Y, Tao R, Ma P, Wang X, Su R, Zhou P, et al. 560 W all fiber and polarization-maintaining amplifier with narrow linewidth and near-diffraction-limited beam quality. Appl Opt (2015) 54:7258–63. doi:10.1364/AO.54.007258
37. Boyd RW, Rzaewski K, Narum P. Noise initiation of stimulated Brillouin scattering. Phys Rev A (Coll Park) (1990) 42:5514–21. doi:10.1103/PhysRevA.42.5514
Keywords: fiber amplifier, narrow linewidth, high power, stimulated Brillouin scattering, spectral optimization
Citation: Lai W, Ma P, Song J, Ren S, Liu W and Zhou P (2022) Optimizing the spectrum of high power narrow linewidth fiber amplifier through the same complex degree of coherence. Front. Phys. 10:1062533. doi: 10.3389/fphy.2022.1062533
Received: 06 October 2022; Accepted: 19 October 2022;
Published: 03 November 2022.
Edited by:
Xinzhong Li, Henan University of Science and Technology, ChinaReviewed by:
Haiyong Zhu, Wenzhou University, ChinaJingjing Zheng, Beijing Jiaotong University, China
Copyright © 2022 Lai, Ma, Song, Ren, Liu and Zhou. This is an open-access article distributed under the terms of the Creative Commons Attribution License (CC BY). The use, distribution or reproduction in other forums is permitted, provided the original author(s) and the copyright owner(s) are credited and that the original publication in this journal is cited, in accordance with accepted academic practice. No use, distribution or reproduction is permitted which does not comply with these terms.
*Correspondence: Pengfei Ma, c2hhbmRhcGVuZ2ZlaUAxMjYuY29t; Pu Zhou, emhvdXB1MjAzQDE2My5jb20=