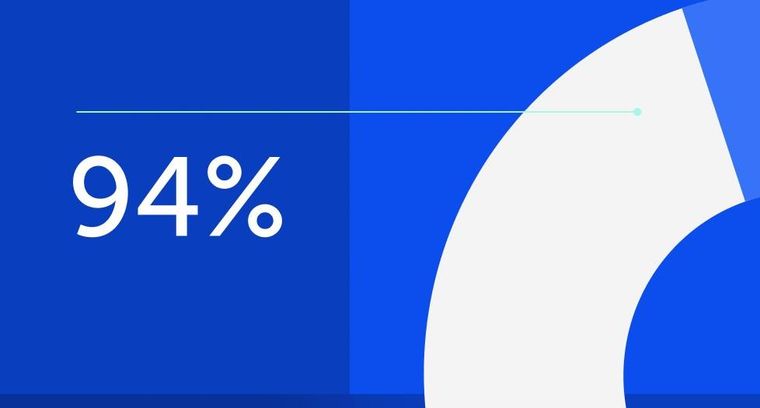
94% of researchers rate our articles as excellent or good
Learn more about the work of our research integrity team to safeguard the quality of each article we publish.
Find out more
ORIGINAL RESEARCH article
Front. Phys., 02 December 2022
Sec. Biophysics
Volume 10 - 2022 | https://doi.org/10.3389/fphy.2022.1058615
This article is part of the Research TopicBiomechanical Performance and Relevant Mechanism of Physical Medicine and Rehabilitation for Neuromusculoskeletal DisordersView all 32 articles
Flatfoot is a common foot deformity, and the collapse of the arch structure affects the foot cushioning during walking. A growing number of scholars have found that the analysis of the impact force should be combined with both the magnitude and the frequency of the impact force. Therefore, the aim of this study is to investigate the plantar impact characteristics of flatfoot patients and normal foot people at different load-bearing buffer stage from the time and frequency domains. Sixteen males with flatfoot and sixteen males with normal foot were recruited to walk on the plantar pressure test system at the same step speed, and the vertical ground reaction force data were collected from the heel contact stage and the arch support stage. The differences in the frequency domain of the ground reaction force between the flatfoot and the normal foot in the two stages were analyzed according to the basic mechanical characteristics and the continuous wavelet transform. Independent sample t-test was used to compare the baseline data of subjects, and the differences in foot impact force characteristics at different stages of foot type and weight-bearing cushion phase were compared by two-factor repeated measures Analysis of Variance. 1) In terms of basic mechanical characteristics, In both groups for flatfoot patients and normal foot people, the peak ground reaction force was higher in the arch support stage compared to the heel contact stage (Pflatfoot<0.001, Pnormal foot<0.001), and the load rate of force change was smaller in this stage (Pflatfoot<0.001, Pnormal foot<0.001). However, no differences in peak ground reaction force and time of occurrence were found between flatfoot and normal foot in the two stages (p > 0.05), in the arch support stage, the force change load rate of patients with flatfoot was lower than that of normal foot people (p = 0.021). 2) The results of time and frequency domain characteristics showed that during the heel contact and the arch support stage, no significant differences in the maximum signal power as well as the corresponding time and the frequency of the main impact force between the normal foot and the flatfoot were found. In both flatfoot and normal foot types, compared with the foot heel contact stage, the maximum signal power in the arch support stage was higher (Pflatfoot < 0.001, Pnormal foot<0.001), and the corresponding impact frequency was smaller (Pflatfoot = 0.002, Pnormal foot = 0.004). Once the step speed was controlled, the flatfoot patients only showed a smaller impact force load rate in the arch support stage, which may be related to their lower arch rigidity. The characteristics of the impact force in different stages of walking support period were different in time and frequency domain, suggesting that there may be differences in the function of various parts of the foot.
Flatfoot is a foot deformity caused by the collapse of the arch [1,2]. As an important structure of the foot, the arch performs an elastic cushioning function through changes in height during movement, therefore, the collapse of the arch is considered to be an important factor that increases the risk of lower limb injury [1,2,3]. In childhood, the foot structure tends to have a flattened appearance, mostly due to incomplete foot development [4], while in elderly, the decline in foot function due to aging makes the foot muscles and ligaments unable to maintain the shape of the arch, which results in flatfoot [5]. It is believed that abnormal foot structure can affect the walking stability of the elderly and become a potential risk for falls [6]. During standing, patients with flatfoot have a higher magnitude and speed of plantar center-of-pressure trajectory sway than normal foot people and exhibit poorer static postural control [7,8], and Harrison’s team showed that as the severity of flatfoot deformity increases, static postural stability decreases [7]. When walking, patients with flatfoot had higher impulse in the heel and arch regions compared to normal foot people [2], and flatfoot exhibited excessive pronation of the foot with lower center of pressure excursion index (CPEI) when pedaling and extending [9]. While running, patients with flatfoot exhibited greater range of motion in the hindfoot, midfoot, and forefoot than normal foot people, suggesting a greater risk of sports injury [10].
Plantar mechanics is an important reference for analyzing the biomechanical characteristics of flatfoot patients. In a majority of movements, the foot is the only motor organ in contact with the ground. During this time, the human body obtains external information in the form of mechanics through plantar proprioception [11,12]. With the gradual study of impact force, researchers found that the study of impact force cannot be limited to the basic characteristics of force. Nigg’s team proposed that the response mechanism of human motion system to impact force should include two angles: time domain and frequency domain. Larger impact loads, impact frequencies, or soft tissue resonances may increase the risk of lower extremity injury [13,14], meanwhile, the basic mechanical indicators used to quantify the impact characteristics may not truly characterize the signals generated by foot-ground collisions [15]. Therefore, impact forces should be studied as input signals with amplitude, time and frequency domains. In the process of walking, the heel and foot arch perform passive cushioning to absorb and transfer the load [16], due to arch collapse and heel exostosis, the cause of sports injuries in flatfoot is mostly thought to be excessive local impact loading of the foot during the load-bearing buffer phase. However, it is still unknown whether changes in foot structure affect the frequency domain characteristics of plantar impact forces in patients with flatfoot and whether this change is responsible for the lower extremity injury in flatfoot. Moreover, structural variations in different parts of the foot lead to their functional differentiation, which may affect the frequency of impact forces corresponding to each part of the foot, therefore, the existence of time-frequency domain variation in different touchdown phases of the foot during the walking support period may also be a new direction to explore the dynamic function of the foot. In summary, combining basic mechanical methods and time-frequency domain analysis to investigate the plantar impact characteristics during walking not only can investigate the possible injury mechanisms in patients with flat feet, but also provides a new research method to assess dynamic foot function.
Continuous Wavelet Transform (CWT) is a time-frequency domain analysis of signals by specific wavelet functions according to different scaling scales. Compared with the Fourier Transform (FT), the CWT enhances the frequency domain analysis of signals at different times. It was found that CWT had better sensitivity compared to traditional median frequency (MF) and mean power frequency (MPF) when assessing muscle fatigue status [17]. After CWT analysis, the research revealed that for visual, proprioceptive interference and foot type differences all affected the frequency domain characteristics of the standing plantar center of pressure trajectory [7,18]. The CWT analysis of impact force also showed that rearfoot pattern runners had higher impact force frequencies during the running support period compared to non-rearfoot pattern runners [15]. The above researches all indicated that CWT has beneficial applications in biomechanical fields such as surface myoelectric signal processing and nonlinear analysis of mechanical signals. In this paper, we combine basic mechanical characteristics and CWT method to compare the load magnitude and time-frequency domain characteristics of vertical ground reaction forces during buffering phase of the walking support period between normal foot and flatfoot. The aims of the paper is to investigate the effects of the weight-bearing cushion phase and foot type differences on plantar impact characteristics during the walking support period. The hypotheses of this study are as follows: 1) In the buffering stage of walking, the plantar impact load of patients with flatfoot is larger than that of normal foot, and the frequency of main impact force may be different from that of normal foot; 2) The weight-bearing cushion dominated by different parts of the foot during the heel touch phase and arch support phase may lead to differences in the time-domain and frequency-domain characteristics of plantar forces.
Thirty-two male subjects were recruited. PASS (Version 15, NCSS, United States) was used for sample size estimation, the minimum effect size was set at 0.90, and the significance level was set at 0.05. When the correlation between different buffer stages within a group was 0.5 and the difference in foot type between groups was 50%, the minimum sample size determined was 22 (Tests for Two Groups of Pre-Post Scores). Combining individual differences in subjects and potential sample attrition, the total sample size was finally determined to be 32.
The foot type was distinguished by foot arch index in 3D foot scanner (3DPODOMED, Beijing Haidemi Investment Co., Ltd. accuracy ± 1.0 mm). Among them, 16 were normal foot (arch index 0.21-0.26) [19] and 16 were flatfoot (arch index >0.30) [19]. The subjects were required to have normal exercise ability (Move freely without pain or discomfort.), no lower limb injury in recent 3 months and no history of lower limb surgery. All the recruited subjects were familiar with this experimental procedure, and volunteered to participate in the experimental test. This study was endorsed by the University Ethics Committee of Hebei Normal University (No. 2022LLSC026).
Each subject walked barefoot on a 2 m high frequency plantar pressure plate (RSscan International Company of Belgium, collecting frequency 120 Hz, sensor density 4/cm2) for 5 times to control the walking speed and record the plantar pressure data of the dominant lateral foot closest to 1.30 m/s. The walking speed was calculated by dividing the stride length by the stride time, while the stride length and stride time were the distance and time interval between the heel touching the pressure plate twice, respectively (Figure 1). Dominant lateral foot was defined as the preferred leg for a ball kicking [20].
The time of the walking support period of each subject was normalized from 0% to 100%, and the ground reaction force during the walking support period was normalized as a percentage of the gravitational force applied to each subject, the ground reaction force diagram during the walking support period was drawn (Figure 2). In this study, we mainly analyzed the characteristics of ground impact force during the weight-bearing cushion phase (red line in Figure 2) so that we could investigate whether heel exostosis and arch collapse in patients with flatfoot would affect the foot cushioning function, and the mechanical characteristics of different parts of the foot during cushioning. As shown in Figure 1, point A is the moment of forefoot touchdown [21,22], and from the hindfoot touchdown to point A is the heel contact stage; point B is the first peak of ground reaction force [21,23], and from point A to point B is the arch support stage.
The peak value of ground reaction force, the time of peak occurrence and the load rate of force were calculated for different foot types during the heel touch phase and arch support phase, respectively, where the load rate of force was calculated by the average slope of the curve, as follows [24,25]:
Where k is the average slope of the curve and
The continuous wavelet transform calculates the inner product between the wavelet basis function and the original signal at different scaling scales through time translation of the wavelet basis function, and then adjusts the time resolution and frequency resolution. Among them, the integral of the wavelet basis function of the selected mother wavelet is close to 0 in its function interval, and its waveform should be similar to that of the original signal as far as possible to ensure the accuracy of the analytical results. Different scaling scales will change the center frequency of the mother wavelet. A larger scale means a lower central frequency of the mother wavelet and a narrower bandwidth in the frequency domain, so the resolution of the frequency is higher. Similarly, a smaller scale means a wider bandwidth in the frequency domain, resulting in a higher temporal resolution.
According to previous studies [15], the morlet wavelet function was selected to perform a continuous wavelet transform (Matlab 2017a, Mathwork, United States) with a scale of 1–200 (resolution frequency of 0.6-120 Hz) and a step size of 0.5 on the ground reaction force normalized to the heel touch stage and the arch support stage respectively, and the time-frequency domain analysis cloud of the ground reaction force is shown as Figure 3. The indicators of this study include maximum signal power, the time of maximum signal power and the frequency of maximum signal power to reflect the time-frequency domain characteristics of the impact force.
FIGURE 3. Analysis diagram of ground reaction in time and frequency domain. (Note: The color bar represents the signal power. As shown in Figure 2, the main frequency of the analytical signal is between 5–25 Hz, and the period of occurrence is in the first 5%–9% of the support period).
The mean and standard deviation for each variable were calculated. Normal distribution check of variables by Shapiro–Wilk test. Independent samples t-test was used to compare the baseline information of normal and flatfoot, and two-factor repeated measures ANOVA (Analysis of Variance) was used to compare the plantar impact characteristics of two foot types at different stages of walking support period, the Bonferroni method was used for simple effect comparisons (SPSS 25.0, IBM, United States), with the significance level α was set as 0.05.
As Table 1 shows, there was no significant difference in age, height and weight between the flatfoot group and the normal foot group (p > 0.05), while no difference was found in the walking speed between the two groups (p > 0.05). The subjects were similar in basic condition and the experimental conditions were well controlled.
For the peak ground reaction force, only different buffer stages had main effect (Table 2), compared with the heel contact stage, the peak ground reaction force of both flatfoot and normal foot in the arch support stage was higher (Pflatfoot<0.001, Pnormal foot<0.001). However, there was no significant difference in the peak ground reaction force between the flatfoot and the normal foot in the two stages (Pheel contact = 0.793, Parch support = 0.453).
The buffer stages main effects also appeared in the time of the peak force and load rate of force change. However, no significant differences were found between foot types in the above two indicators (Table 2). Specifically, the load rate of force change was lower in both flatfoot and normal foot during the arch support stage than the heel contact stage (Pflatfoot<0.001, Pnormal foot<0.001). In addition, in the arch support stage, the force change load rate of patients with flatfoot was lower than that of normal foot people (p = 0.021).
The main effect for stage was observed in both maximum signal power and the time of maximum signal power, no significant differences were found in the above indicators for foot type (Table 3). Moreover, for the maximum signal power, compared with the heel contact stage, the maximum signal power of the flatfoot and normal foot of foot arch support stage was higher (Pflatfoot<0.001, Pnormal foot<0.001).
There was a main effect for stage in the frequency of maximum signal power, neither foot type main effect nor interaction effect were found in this indicator (Table 3). For both of the flatfoot and normal foot, the frequency of maximum signal power in arch support stage was lower than that of the heel contact stage (Pflatfoot = 0.002, Pnormal foot = 0.004).
Walking is one of the basic movements of the human body, the foot, as the end link in direct contact with the ground during walking, has a complex structure that allows the human body to accomplish weight-bearing cushioning and stretching functions. Due to the slower speed during walking, all parts of the foot can fully contact the ground; [12,26,27]. In the load-bearing buffering stage, the heel first touches the ground, at which time the strike between the heel and the ground produces a peak force, as shown at point A in Figure 1, which is known as the “heel strike transient” [21,23], and then the foot rolls forward around the heel axis, the midfoot gradually touches the ground, when the arch plays a dominant role in cushioning [23]. At this time, the human body adjusts the foot posture according to the characteristics of the ground reaction force, while viscoelastic structures such as foot fascia stores elastic potential energy [12,28]. The results of this study showed that the slope of the ground reaction of the two types of foot in the arch support stage was lower than that in the heel contact stage (p < 0.05). In the heel contact stage, although the human body is in the double-support phase while the contralateral limb bears part of the impact load, the load rate of change in the vertical ground reaction force is still higher than that in the arch support phase, which indicates the arch structure of the foot effectively reduces the impact of ground reaction force, reflecting the elastic cushioning function of the foot.
In the arch support stage, our study found that the force change load rate of flatfoot patients was lower than that of normal foot people (p < 0.05), suggesting that the arch of the normal foot may exhibit greater rigidity in response to impact loading, which is consistent with the previous findings of our group [29]. A finite-element analysis of flatfoot [30]. found that lower plantar fascia rigidity and inadequate strength of the peroneus longus affects the ability to maintain the arch of the foot, the result also explains the findings of our study. From these results, it can be concluded that for patients with flatfoot, the lower arch rigidity requires the selection of orthotic insoles with better foot support for functional foot assistance. However, no significant differences were found between normal and flatfoot in the peak ground reaction force and the time of peak ground reaction during both heel contact and arch support stage. A study of cadaveric foot [31] showed that sustained impact loading increased the flatness of flatfoot, this finding suggests that flatfoot is less adaptive and prolonged exercise may further amplify the differences in foot function between flatfoot patients and normal foot people. Since the physical differences between the subjects in this study were small and the step speed was controlled during the test, the foot type differences have less effect on the peak plantar impact force, therefore, it can be speculated that the main factors affecting the magnitude and corresponding time of ground reaction force during the walking buffer period may be the step speed and the effective mass at the time of impact.
In the field of sports medicine, earlier studies mostly considered that the main cause of sports injuries was the accumulation of load or excessive impact, and the biomechanical studies of basic sports such as walking, running and jumping mostly took the time domain perspective as the entry point. With the in-depth study of frequency domain, scholars found that human bones (200–900 Hz) [13,32] and soft tissues (10–60 Hz) [33] have corresponding inherent frequencies respectively, while the impact force frequency domain during running is around 10–20 Hz [15,34], which is closer to the inherent frequency of soft tissues, making the impact force frequency and the inherent frequency of soft tissues resonate and lead to soft tissue injury. This view provides a new perspective on the mechanism of sports injury.
During walking, all parts of the foot are in full contact with the ground, which makes the foot function more sufficiently embodied. In this study, the ground reaction force in the load-bearing cushion phase during the walking support period was considered as a set of input signals with time-frequency domain characteristics, however, in the time-frequency domain perspective, our study did not find significant differences in the maximum signal power, corresponding time and impact force frequency between flatfoot patients and normal foot people during the load-bearing cushion phase, which suggests that after controlling for step speed, arch collapse has little effect on the frequency domain characteristics of plantar impact during low-intensity and short-term exercise, while significant changes in plantar impact characteristics may need to be further reflected by high-intensity or prolonged exercise.
This study showed that the maximum signal power of the arch support stage is higher than that of the heel contact stage, indicating that the plantar impact is mainly concentrated in the arch support stage. Meanwhile, the arch support stage exhibited a lower impact force frequency compared to that of the heel contact stage. Some scholars believe that the musculoskeletal system of the human body plays the role of a “low-pass filter” during movement, and its purpose is to ensure the stability of the head during movement so that the brain can accurately process visual and vestibular inputs and improve movement stability to prevent falls [35,36], while the angle of the joint, the degree of muscle activation, and the skeletal muscle composition all change the cut-off frequency of the “filter” [14,36,37]. The results of this study support this argument, even in different parts of the foot, structural differences can also make a change in the frequency of the impact force on each part of the foot. Shorten’s team found that high-frequency impact force was mainly present in the initial landing phase of running, while low-frequency impact force accounted for a gradual increase in the middle of running support [38]. Although the movement studied in our paper was walking, due to the similarity of movement patterns, the results of both this research and Shorten’s team suggest that there may be differences in impact frequencies in various parts of the foot due to structural differences. In the heel contact stage, the human body responds to ground impact with the ankle-hindfoot as the dominant, the heel bone is subjected to larger rigid impact resulting in a higher force change load rate and impact force frequency. While in the arch support phase, the weight-bearing cushion of the foot is dominated by the arch, and the mid-tarsal joint and plantar fascia sufficiently carry out elastic cushioning, thus showing smaller force change load rate and impact force frequency. The above differences indicate that frequency domain characteristics can be a new perspective for evaluating foot function.
In this study, the time-frequency domain analysis provided a new perspective on the plantar impact characteristics during the weight-bearing cushioning phase of the walking support period, but there are still some limitations in our study, the present research only investigated the time-frequency domain characteristics of impact force under low-intensity exercise like walking in male flatfoot patients, while the motion characteristics under high-load impact such as running and jumping in female and different age groups of patients with flatfoot also need some data support, and it is suggested that future studies should be supplemented in this aspect.
After controlling the gait speed, it is hard to find the difference in impact force frequency between flatfoot patients and normal foot people during the weight-bearing cushioning phase, the smaller impact force change load rate in the arch support stage of flatfoot patients may due to their lower arch rigidity, the better foot support footwear is recommended for flatfoot patients. Variations in foot function in different parts of the foot may cause different frequency domain characteristics during the weight-bearing cushioning phase.
It is recommended that future research on the plantar mechanics of flatfoot should extend the sample size and increase the study of injury mechanisms in people of different genders and ages during prolonged or high-intensity exercise.
The raw data supporting the conclusion of this article will be made available by the authors, without undue reservation.
The studies involving human participants were reviewed and approved by the University Ethics Committee of Hebei Normal University (No. 102772021RT034). The patients/participants provided their written informed consent to participate in this study.
XB and HH were in charge of designing the experiment. XB wrote the manuscript. PL, YL, AK, and JL revised the manuscript. All authors have read and approved the final manuscript.
This research was supported by Tsinghua University Initiative Scientific Research Program (2021THZWJC15), Science and Technology Research Project of Higher Education Institutions in Hebei Province (SQ2021215).
The authors declare that the research was conducted in the absence of any commercial or financial relationships that could be construed as a potential conflict of interest.
All claims expressed in this article are solely those of the authors and do not necessarily represent those of their affiliated organizations, or those of the publisher, the editors, and the reviewers. Any product that may be evaluated in this article, or claim that may be made by its manufacturer, is not guaranteed or endorsed by the publisher.
1. Ledoux WR, Shofer JB, Ahroni JH, Smith DG, Sangeorzan BJ, Boyko EJ. Biomechanical differences among pes cavus, neutrally aligned, and pes planus feet in subjects with diabetes. Foot Ankle Int (2003) 24(11):845–50. doi:10.1177/107110070302401107
2. Hillstrom HJ, Song J, Kraszewski AP, Hafer JF, Mootanah R, Dufour AB, et al. Foot type biomechanics part 1: Structure and function of the asymptomatic foot. Gait & Posture (2013) 37(3):445–51. doi:10.1016/j.gaitpost.2012.09.007
3. Newman P, Witchalls J, Waddington G, Adams R. Risk factors associated with medial tibial stress syndrome in runners: A systematic review and meta-analysis. Open Access J Sports Med (2013) 4:4229–41. doi:10.2147/OAJSM.S39331
4. Pfeiffer M, Kotz R, Ledl T, Hauser G, Sluga M. Prevalence of flat foot in preschool-aged children. Eur J Pediatr (2006) 118(2):634–9. doi:10.1542/peds.2005-2126
5. Scott G, Menz HB, Newcombe L. Age-related differences in foot structure and function. Gait & Posture (2007) 26(1):68–75. doi:10.1016/j.gaitpost.2006.07.009
6. Mickle KJ, Munro BJ, Lord SR, Menz HB, Steele JR. Foot pain, plantar pressures, and falls in older people: A prospective study. J Am Geriatr Soc (2010) 58(10):1936–40. doi:10.1111/j.1532-5415.2010.03061.x
7. Kim JA, Lim OB, Yi CH. Difference in static and dynamic stability between flexible flatfeet and neutral feet. Gait & Posture (2015) 41(2):546–50. doi:10.1016/j.gaitpost.2014.12.012
8. Chao TC, Jiang BC. A comparison of postural stability during upright standing between normal and flatfooted individuals, based on cop-based measures. Entropy (2017) 19(2):76–13. doi:10.3390/e19020076
9. Song J, Choe K, Neary M, Zifchock RA, Cameron KL, Trepa M, et al. Comprehensive biomechanical characterization of feet in usma cadets: Comparison across race, gender, arch flexibility, and foot types. Gait & Posture (2018) 60:60175–80. doi:10.1016/j.gaitpost.2017.12.001
10. Takabayashi T, Edama M, Inai T, Kubo M. Differences in rearfoot, midfoot, and forefoot kinematics of normal foot and flatfoot during running. J Orthop Res (2021) 39(3):565–71. doi:10.1002/jor.24877
11. Hageman ER, Hall M, Sterner EG, Mirka GA. Medial longitudinal arch deformation during walking and stair navigation while carrying loads. Foot Ankle Int (2011) 32(6):623–9. doi:10.3113/FAI.2011.0623
12. Shen Z, Xi-ni Z, Ke-dong C, Wei-jie F, Yu L. The development of the longitude arch motor function and its biomechanical contribution to human movement. China Sport Sci (2018) 38(05):73–9. doi:10.16469/j.css.201805008
13. Boyer KA, Nigg BM. Muscle tuning during running: Implications of an un-tuned landing. J Biomech Eng-t Asme (2006) 128(6):815–22. doi:10.1115/1.2354202
14. Boyer KA, Nigg BM. Changes in muscle activity in response to different impact forces affect soft tissue compartment mechanical properties. J Biomech Eng-t Asme (2007) 129(4):594–602. doi:10.1115/1.2746384
15. Allison HG, Joseph H, Timothy RD, Katherine AB. A comparison of the ground reaction force frequency content during rearfoot and non-rearfoot running patterns. Gait Posture (2017) 56:5654–9. doi:10.1016/j.gaitpost.2017.04.037
17. Beck TW, Stock MS, Defreitas JM. Shifts in emg spectral power during fatiguing dynamic contractions. Muscle Nerve (2014) 50(1):95–102. doi:10.1002/mus.24098
18. Sim T, Yoo H, Lee D, Suh SW, Yang JH, Kim H, et al. Analysis of sensory system aspects of postural stability during quiet standing in adolescent idiopathic scoliosis patients. J Neuroengineering Rehabil (2018) 15(1):54. doi:10.1186/s12984-018-0395-6
19. Cavanagh PR, Rodgers MM. The arch index - a useful measure from footprints. J Biomech (1987) 20(5), 547–51. doi:10.1016/0021-9290(87)90255-7
20. Niu WX, Yao J, Chu ZW, Jiang CH, Zhang M, Fan YB. Effects of ankle eversion, limb laterality, and ankle stabilizers on transient postural stability during unipedal standing. J Med Biol Eng (2015) 35(1):69–75. doi:10.1007/s40846-015-0007-x
21. Verdini F, Marcucci A, Benedetti MG, Leo T. Identification and characterisation of heel strike transient. Gait & Posture (2006) 24(1):77–84. doi:10.1016/j.gaitpost.2005.07.008
22. Chiu M, Wu H, Chang L. Gait speed and gender effects on center of pressure progression during normal walking. Gait & Posture (2013) 37(1):43–8. doi:10.1016/j.gaitpost.2012.05.030
23. Whittle MW. Generation and attenuation of transient impulsive forces beneath the foot: A review. Gait & Posture (1999) 10(3), 264–75. doi:10.1016/S0966-6362(99)00041-7
24. Lieberman DE, Venkadesan M, Werbel WA, Daoud AI, D'Andrea S, Davis IS, et al. Foot strike patterns and collision forces in habitually barefoot versus shod runners. Nature (2010) 463(7280):531–5. doi:10.1038/nature08723
25. Rice HM, Jamison ST, Davis IS. Footwear matters: Influence of footwear and foot strike on load rates during running. Med Sci Sport Exer (2016) 48(12):2462–8. doi:10.1249/MSS.0000000000001030
26. Wolf P, Stacoff A, Liu A, Nester C, Arndt A, Lundberg A, et al. Functional units of the human foot. Gait & Posture (2008) 28(3):434–41. doi:10.1016/j.gaitpost.2008.02.004
27. Chiu MC, Wu HC, Chang LY, Wu MH. Center of pressure progression characteristics under the plantar region for elderly adults. Gait & Posture (2013) 37(3):408–12. doi:10.1016/j.gaitpost.2012.08.010
28. Kelly LA, Cresswell AG, Racinais S, Whiteley R, Lichtwark G. Intrinsic foot muscles have the capacity to control deformation of the longitudinal arch. J R Soc Interf (2014) 11(93):20131188. doi:10.1098/rsif.2013.1188
29. Xiaotian B, Hongfeng H. The influence of arch difference on dynamic and static foot-ankle function. J Med Biomech (2021) 36(06):951–6. doi:10.16156/j.1004-7220.2021.06.019
30. Cifuentes-De La Portilla C, Pasapula C, Gutierrez-Narvarte B, Larrainzar-Garijo R, Bayod J. Peroneus longus overload caused by soft tissue deficiencies associated with early adult acquired flatfoot: A finite element analysis. Clin Biomech (2021) 86105383:105383. doi:10.1016/j.clinbiomech.2021.105383
31. Chu IT, Myerson MS, Nyska M, Parks BG. Experimental flatfoot model: The contribution of dynamic loading. Foot Ankle Int (2001) 22(3):220–5. doi:10.1177/107110070102200309
32. Nigg BM, Wakeling JM. Impact forces and muscle tuning: A new paradigm. Exerc Sport Sci Rev (2001) 29(1):37–41. doi:10.1097/00003677-200101000-00008
33. Wakeling JM, Nigg BM, Rozitis AI. Muscle activity damps the soft tissue resonance that occurs in response to pulsed and continuous vibrations. J Appl Physiol (2002) 93(3):1093–103. doi:10.1152/japplphysiol.00142.2002
34. Wang X, Zhang S, Fu W. Changes in impact signals and muscle activity in response to different shoe and landing conditions. J Hum Kinetics (2017) 565:5–18. doi:10.1515/hukin-2017-0018
35. Mercer JA, Devita P, Derrick TR, Bates BT. Individual effects of stride length and frequency on shock attenuation during running. Med Sci Sports Exerc (2003) 35(2):307–13. doi:10.1249/01.MSS.0000048837.81430.E7
36. Edwards WB, Derrick TR, Hamill J. Musculoskeletal attenuation of impact shock in response to knee angle manipulation. J Appl Biomech (2012) 28(5):502–10. doi:10.1123/jab.28.5.502
37. Allison HG, Katherine AB, Timothy RD, Joseph H. Impact shock frequency components and attenuation in rearfoot and forefoot running. J Sport Health Sci (2014) 3(2):113–21. doi:10.1016/j.jshs.2014.03.004
Keywords: gait, flatfoot, impact force, continuous wavelet transform, biomechanics
Citation: Bai X, Huo H, Lu P, Luan Y, Koga A and Liu J (2022) Analysis of plantar impact characteristics of walking in patients with flatfoot according to basic mechanical features and continuous wavelet transform. Front. Phys. 10:1058615. doi: 10.3389/fphy.2022.1058615
Received: 30 September 2022; Accepted: 22 November 2022;
Published: 02 December 2022.
Edited by:
Qipeng Song, Shandong Sport University, ChinaReviewed by:
Guohai Chen, Dalian University of Technology, ChinaCopyright © 2022 Bai, Huo, Lu, Luan, Koga and Liu. This is an open-access article distributed under the terms of the Creative Commons Attribution License (CC BY). The use, distribution or reproduction in other forums is permitted, provided the original author(s) and the copyright owner(s) are credited and that the original publication in this journal is cited, in accordance with accepted academic practice. No use, distribution or reproduction is permitted which does not comply with these terms.
*Correspondence: Jingmin Liu, bGptX3RoQG1haWwudHNpbmdodWEuZWR1LmNu
‡ORCID: Hongfeng Huo, orcid.org/0000-0002-8755-430X; Yisheng Luan, orcid.org/0000-0003-0858-1893
Disclaimer: All claims expressed in this article are solely those of the authors and do not necessarily represent those of their affiliated organizations, or those of the publisher, the editors and the reviewers. Any product that may be evaluated in this article or claim that may be made by its manufacturer is not guaranteed or endorsed by the publisher.
Research integrity at Frontiers
Learn more about the work of our research integrity team to safeguard the quality of each article we publish.