- 1Department of Dermatology and Department of Laser and Aesthetic Medicine, Shanghai Ninth People's Hospital, School of Medicine, Shanghai Jiao Tong University, Shanghai, China
- 2School of Biomedical Engineering, Shanghai Jiao Tong University, Shanghai, China
Pulsed lasers at the near infrared (NIR) range have been widely used in dermatology. Ultrashort pulsed picosecond lasers are found with the specific ability of very effective activation of skin repair and remodeling along with significant photodamage. Femtosecond lasers, with a shorter pulse width, may be a promising alternative to current NIR lasers in clinic. In this study, we performed optical micromachining by a femtosecond laser at 1,030 nm to skin of live mice in two modes of scanning of focused laser and direct irradiation by unfocused laser. The acute and one-day delayed immune molecular responses of the skin to the micromachining are studied by immunofluorescence microscopy of the skin sections. Our data shows the focused laser can activate remodeling of skin without any significant immune responses. In contrast, the direct irradiation by the unfocused laser activate significant immune responses in the deep dermis with high regulation of interleukin. Those results suggest focused femtosecond laser is of good promising potential in activation of skin remodeling and repairing with little immune or physical damage.
Introduction
Lasers have been widely used in clinical dermatology for diagnostics and therapy. In the treatment of immune and pigmented diseases of skin, micro-plastic surgery, and photo-rejuvenation, different types of lasers are selected for different therapy purposes [1–8]. The continuous-wave lasers at different wavelengths (usually at the visible range) that can be directly absorbed by the pigments and biomolecules in skin can disrupt the abnormal pigments and activate immune processes for inflammation treatment. The pulsed lasers, especially the Q-switched nanosecond lasers at the near infrared (NIR) range, can generate significant thermal effect in dermis for wound healing, regenerative medicine and photo-rejuvenation. Recently, ultrashort picosecond pulsed lasers are found with significant improvement on tattoo removal treatment of discrete pigmented lesions, rejuvenation, melasma treatment and scar revision in clinic [9–17].
Generally, the pulse width of practical picosecond lasers varies from 300 to 900 ps in clinical dermatology. Compared with the traditional nanosecond pulsed and continuous-wave lasers, the short pulse width of picosecond laser brings a series of advantages [18–20]. Specifically, the thermal deposition decreased significantly. . Briefly, focused picosecond laser pulses contribute high peak power density (over 1013 W/cm2) within the focus of laser beam to produce optical breakdown via multiphoton absorption. This process generates microscopic cavitation bubbles in tissue. The bubbles expand, further generate strong mechanical shockwaves, and finally result in tissue disruption. Notably, residual thermal damage around the micro damage areas is involved in this process. The intense photomechanical effect provides efficient removal of target pigment molecules and promotes skin rejuvenation by direct damage to collagen [9, 21]. Nevertheless, the laser pulses at 100 ps level still lead large thermal diffusion to induce off-target area mechanical damage and non-negligible thermal damage and subsequent edema, inflammation, and other complex immune processes. Currently, the clinical picosecond laser therapy has been found with postoperative complications including erythema, post-inflammatory, hyperpigmentation and blister [9, 22, 23]. Therefore, it is promising to explore lasers with even shorter pulse width in skin micromachining.
In last decades, femtosecond laser has been applied in clinical diagnosis and treatment as a new generation laser technology. Compared to picosecond lasers, femtosecond lasers have much shorter pulse duration (tens to hundreds femtosecond) and thus totally different mechanism of acting with tissue [24, 25]. Optical ablation by femtosecond laser is easily restricted in the focused area with little thermal effect, also enabling the refractive surgery by femtosecond laser [23]. However, femtosecond laser is rarely applied in dermatology. In this study, we visualize the key immune molecular dynamics of skin to micromachining by femtosecond laser. Our data present the activation of skin immune processes and tissue remodeling, and further the molecular mechanism of skin after the laser micromachining.
Materials and Methods
Femtosecond-Laser Micromachining
A femtosecond laser at 1,030 nm (pulse width: 200 fs, repetition rate: 1 MHz) was coupled into a microscope system to perform micromachining on live mice (Figure 1A). The laser beam was expanded to match the back aperture of the objective (×20, N.A. = 0.65) and then focused by it onto the mouse skin at around 50–100 μm deep. The laser focus was around 1 μm in diameter. The micromachining was accomplished by the scanning of the galvo-mirror that could be predefined by any region on the skin of mice. In this study, a rectangular area of 5 mm × 0.64 mm at the mouse back was scanned line by line by the laser. Each line was 0.64 mm, defined by 640 points. Each point was exposed to the laser for 200 µs. The area was finally scanned by 5,000 lines as a trial. The laser power was used at 0.2 W–1 W at the skin surface. After experiments, the mice were sacrificed and the skin tissue were dissected for analysis.
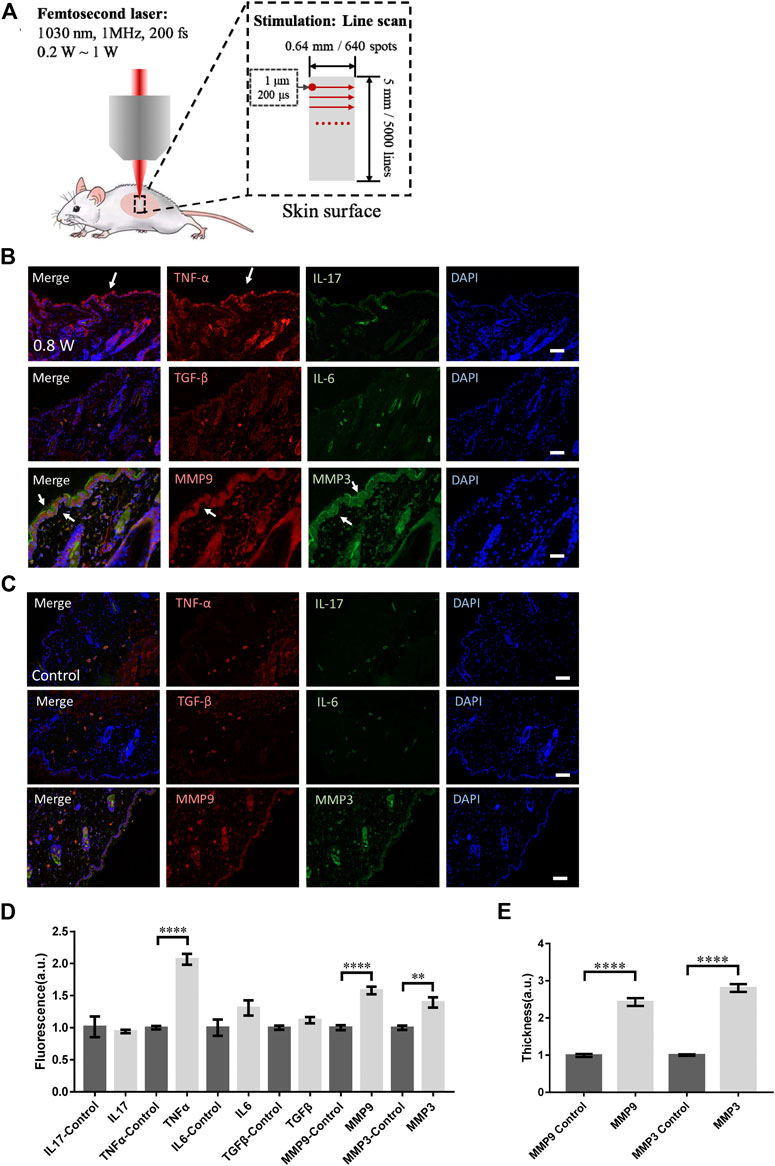
FIGURE 1. Skin responses to micromachining by femtosecond laser. (A) The scheme of micromachining by femtosecond laser on skin of mouse. The scanning of femtosecond laser was controlled by a pair of galvo-mirror. The molecular responses to femtosecond laser micromachining of the skin tissue at 0.8 W (B) and control (C). Arrows: the immunofluorescence of the molecules. Bar: 100 μm. (D) Statistic results of the fluorescence intensity in the experimental and control groups (n = 24 samples from six independent trails). (E) The skin thickness with positive MMP3 and MMP9 signals (n = 24 samples from six independent trails). *p < 0.05. **p < 0.01. ***p < 0.001. ****p < 0.0001, by students’ t-test.
Animal Preparation and Histological Section of Skin
For in vivo experiments, 6- to 9-weeks-old Kunming mice were anesthetized with 4% chloral hydrate. Before experiments, the hair was removed by hair removal cream (Veet Hair Removal Cream, Reckitt Benckiser Ltd.). After the surgical level of anesthesia was reached, the skin was treated by the femtosecond laser. After that, the skin tissues were removed and fixed in 4% paraformaldehyde. All experimental procedures regarding the care and use of SD rat pups were reviewed and approved by the Animal Ethics Committee of Shanghai ninth People’s Hospital and the School of Biomedical Engineering, Shanghai Jiao Tong University. All efforts were made to minimize possible pain and discomfort of the animals during the experimental procedures.
Immunofluorescence Labeling and Microscopy
Paraffin-embedded skin biopsies were cut into 5 µm sections and placed on glass slides. Slides were placed in a 60°C oven overnight, deparaffinized with xylene, then rehydrated with serial ethanol dilutions. The tissue section was placed in a repair box filled with EDTA antigen retrieval buffer (PH 8.0) in a microwave oven for antigen retrieval and were blocked using 10% rabbit serum. Sections were incubated with primary antibodies overnight at 4°C. Sections were double-stained for immunofluorescence of TNF-α (rabbit polyclonal anti-TNF-α, 1:200, Affinity Biosciences, AF7014) and IL-17 (mouse monoclonal anti-IL-17, 1:200, Santa Cruz, sc-374218), MMP3 (mouse monoclonal anti-MMP3,1:200, Proteintech, 66338-1-lg) and MMP9 (rabbit polyclonal anti-MMP9, 1:200, Proteintech, 10375-2-AP), and IL-6 (rat monoclonal anti-IL6R, 1:100, Abcam, ab83053) and TGF-β (rabbit polyclonal anti- TGF-β, 1:200, Proteintech, 21898-1-AP). Then the sections were washed with PBS for 5 min and incubated with diluted secondary antibody for 1.5 h at room temperature. Goat anti-Rat IgG secondary antibody conjugated with FITC (1:200, Servicebio), Goat anti-Mouse IgG secondary antibody conjugated with Alexa Fluor ® 488 (1:400, Servicebio) and Goat anti-rabbit IgG secondary antibody conjugated with Cy3 (1:300, Servicebio) were used for microscopy. Sections were simultaneously counterstained with DAPI before fluorescent microscopy.
Images were acquired by a confocal microscopy system (Olympus FV3000). The power of three excitation lasers at 405 nm, 473 nm, and 543 nm was set at the level of 0.1 mW, which could excite fluorophores effectively with moderate photobleaching. The image size was set as 512 × 512 pixels and time interval for each pixel was 2 µs.
Results
Acute Molecular Responses to Femtosecond Laser Micromachining
We investigated the responses of immune molecules in skin tissue to femtosecond laser micromachining at different powers. To eliminate the thermal accumulation between laser pulses, the pulse repetition rate was set at only 1 MHz. During the micromachining, the transient thermal effect of each femtosecond pulse can decay before the subsequent following pulse. In this regard, the average total thermal effect of the laser was little. At first, the skin tissue scanned by the focused femtosecond laser at 0.8 W line by line was dissected. The immunofluorescence microscopy of IL-17, TNF-α, IL-6, and TGF-β in the histosections of the tissue were performed respectively. Immediately it could be found TNF-α was significantly assembled in the epidermis (Figure 1B) compared with the control (Figure 1C). The immune molecules might come from the keratinocytes in epidermis that suffered the laser stimulation. No signals from IL-17, IL-6, or TGF-β that are usually involved in T cells could be found, indicating the acute response of the skin activated by the focused laser was only unspecific innate immune responses at epidermis (Figure 1D).
Specifically, we examined the matrix metalloproteinase 3 (MMP3) and 9 (MMP9) that are involved in tissue remodeling, wound healing, and breakdown of extracellular matrix proteins including collagen and elastin. As shown in Figures 1B,D, the MMP3 and MMP9 were both significantly upregulated in both epidermis and dermis, indicating the remodeling initiation of the laser-treated skin. The thickness of the skin with those molecular distribution was quantified in Figure 1E. Notably, MMP3 could be found highly in epidermis. In those processes, no physical or physiological damage could be found in the histosections. Still, the focused femtosecond laser could activate the collagen or elastin repair. This result suggests the upregulation of MMP family does not require physical injury by optical micromachining to collagen or elastin. The low level of TGF-β under this condition was consistent with this suspicion.
We then measured the molecular responses to the laser stimulation at different powers. As shown in Figures 2A,B, the TNF-α in epidermis increased moderately at 0.4 W but no signals could be found at 0.2 W. Compared with the control (Figure 1C), no significant differences could be found in IL-6, IL-17, or TGF-β (Figure 2D). The TNF-α level treated at 0.4 W was still significantly higher (Figure 2E). Consistently, the upregulation of MMP3 and MMP9 was also significantly higher at 0.4 W but no signals at 0.2 W (Figure 2E). The thickness of the skin stained with MMP9 and MMP3 signals was also consistent with the result of immunofluorescence (Figure 2F). Therefore, the immune process initiation requires a laser power threshold. At lower laser powers, cells deep in dermis could not be activated for MMP3 and MMP9 expression.
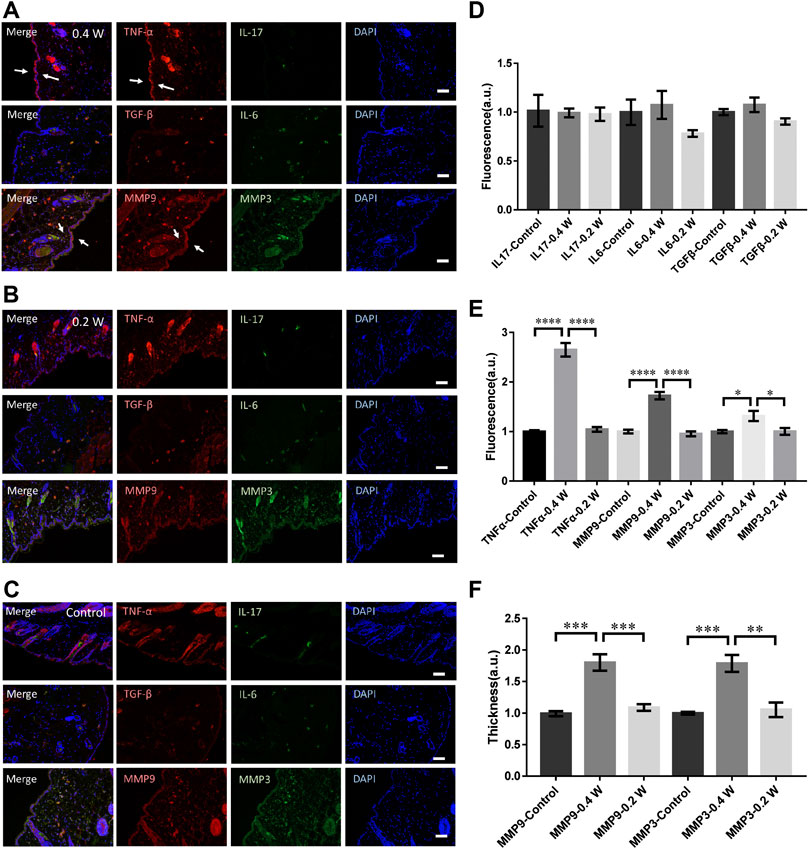
FIGURE 2. Molecular responses of skin to femtosecond-laser micromachining at 0.4 W (A) and 0.2 W (B) respectively. (C) negative control. (D) Statistic results of the fluorescence intensity of IL-17, IL-6, and TGF-β in the experimental and control groups (n = 24 samples from six independent trails). Statistic results of the fluorescence intensity (E) and skin thickness (F) of TNF-α, MMP3, and MMP9 in the experimental and control groups respectively (n = 24 samples from six independent trails). Bar: 100 μm. *p < 0.05. **p < 0.01. ***p < 0.001. ****p < 0.0001, by students’ t-test.
Long-Term Molecular Dynamics to Femtosecond Laser Micromachining
We investigated the long-term molecular responses of skin, in which the mice recovered 1 day after the laser micromachining at 0.8 W. The skin was then dissected for immunofluorescence microscopy, as shown in Figure 3A. The TNF-α level decreased significantly compared with the acute responses, indicating an anti-inflammatory and repair process of skin tissue. But it was still significantly higher than it of control (Figures 3B,C). No signals from IL-17, IL-6, or TGF-β were found either (Figures 3A,C). Therefore, the tissue damage was strictly confined in the laser scanning volume and only innate immune responses were activated. The expression level of MMP3 and MMP9 were still significantly high (Figure 3C). The thickness of the skin with positive MMP3 and MMP9 was consistently higher (Figure 3D). During the repair, the irradiated epidermis initiated cornification to detach from the skin, together with MMP3. Hence, taken the acute MMP expression together, MMP3 might be involved in the remodeling of both dermis, epidermis, and specifically, keratoderma. In general, the focused femtosecond laser could effectively activate the remodeling of collagen/elastin without complex immune responses of skin.
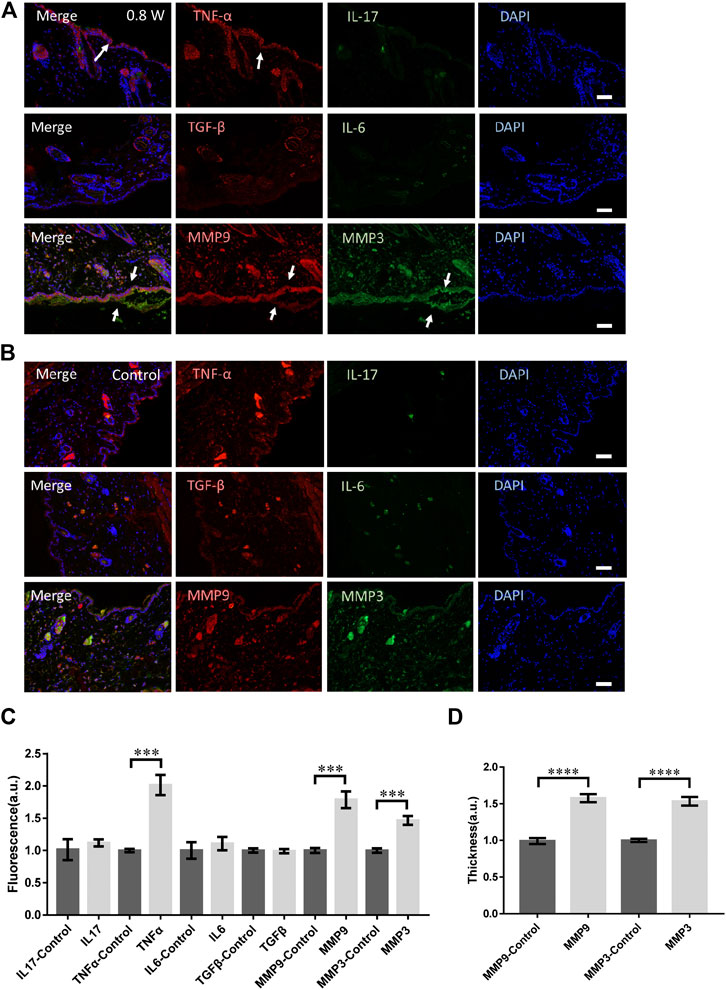
FIGURE 3. The molecular responses to the laser micromachining after one-day recovery. The immunofluorescence of the skin treated at 0.8 W after one-day recovery (A) and the control (B) respectively. (C) The statistics of the fluorescence intensity of each molecule with immune-staining. (D) The skin thickness with MMP3 and MMP9 positive. n = 12 samples from four independent trails. Bar: 100 μm. *p < 0.05. **p < 0.01. ***p < 0.001. ****p < 0.0001, by students’ t-test.
Molecular Responses to Defocused Laser Irradiation
As a comparison, the mice were irradiated by the femtosecond laser at 1 W directly without any focusing for 15 s. The laser spot was around 2 mm in diameter. In this case, the laser could propagate into skin in a deeper distance while the photon density was lower (106 fold lower). As shown in Figure 4A, no obvious physical damage could be found in those skin tissues. The immunofluorescence of TGF-β, IL-17, and IL-6 were all significantly higher (Figure 4B). The TGF-β generally distributed in the epidermis, suggesting the physical damage to skin. IL-6 was upregulated through the whole skin tissue and the muscle, fat under it. But the signals from TNF-α, MMP3, and MMP9 all decreased significantly (Figure 4C), indicating the tissue remodeling might not be initiated. The collagen and elastin repair was not activated either.
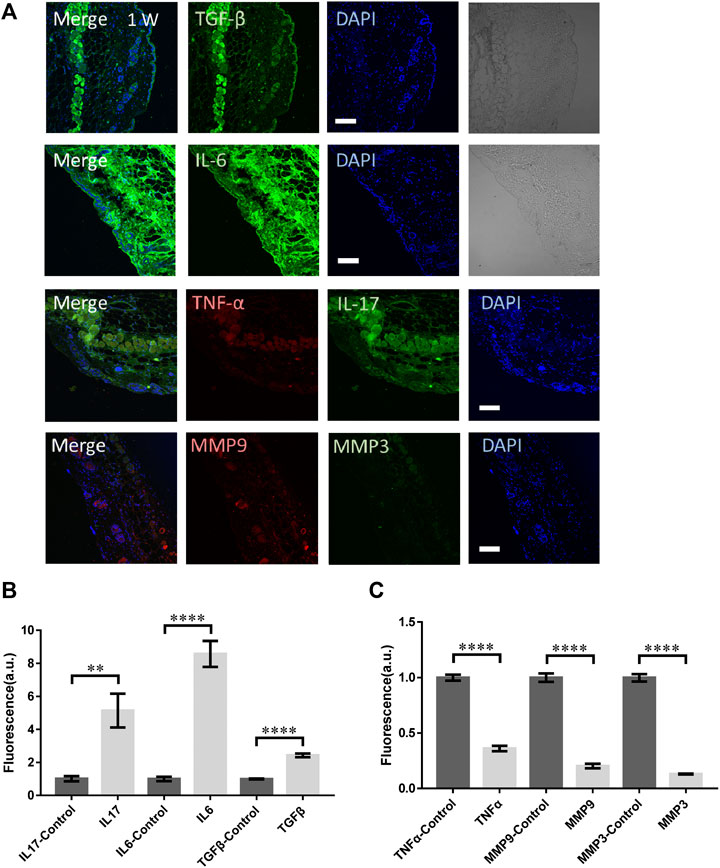
FIGURE 4. The molecular responses of skin to the unfocused laser irradiation at 1 W. (A) The immunofluorescence images and (B,C) the statistical analysis of the laser-treated samples and control respectively. n = 6 samples from three independent trails. Bar: 100 μm. *p < 0.05. **p < 0.01. ***p < 0.001. ****p < 0.0001, by students' t-test.
To compare with the clinic treatment, a widely-used picosecond laser in clinic (PicoSure, Cynosure) at 755 nm was used to treat skin of mouse. The laser spot was 2.5 mm in diameter and pulse width 750 ps. The micromachining was performed at 0.8 W (4.07 J/cm2) for 6 s. As presented in Figures 5A,B, the signals from TNF-α, TGF-β, IL-17, and IL-6 were all significantly higher. Unfortunately, the MMP3 and MMP9 did not show any increase (Figure 5C). Hence the skin suffered mostly damage with little remodeling or repairing.
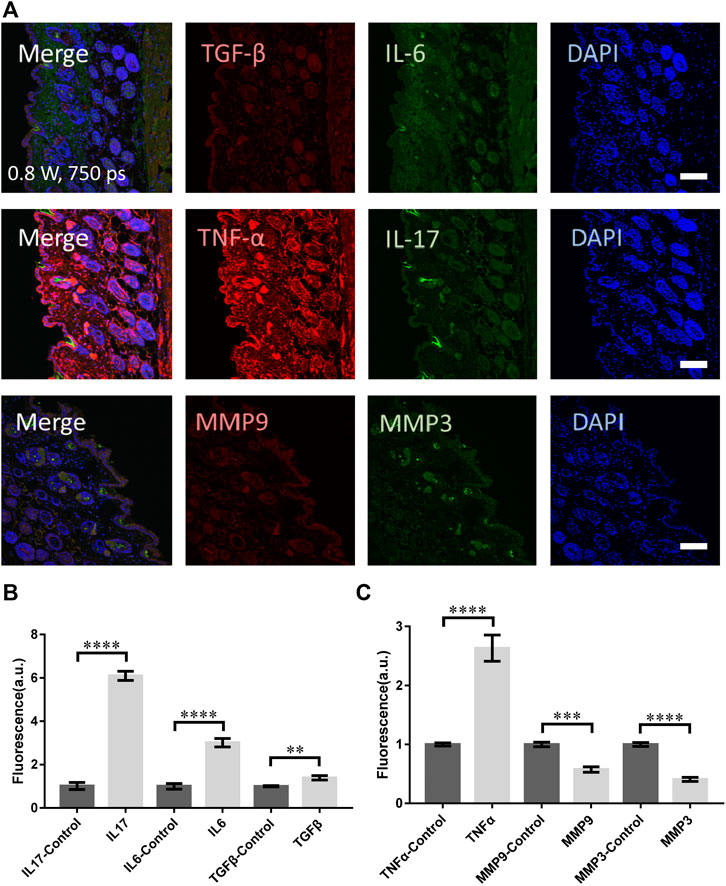
FIGURE 5. The molecular responses of skin to the clinical picosecond laser at 755 nm, irradiation at 0.8 W for 6 s. (A) The immunofluorescence images and (B,C) the statistical analysis of the samples with and without laser treatment respectively. n = 6 samples from three independent trails. Bar: 100 μm. *p < 0.05. **p < 0.01. ***p < 0.001. ****p < 0.0001, by students' t-test.
Conclusion
In this study, we for the first time report the molecular responses of skin tissue from live mice to the femtosecond laser micromachining. According to our data, the focused femtosecond laser can activate the remodeling of skin tissue effectively by the immediately upregulation of MMP3 and MMP9. The low level of IL-6, IL-17, and TGF-β in the skin tissue after laser micromachining suggest no significant immune response or the involvement of T cells participate in this process. The upregulation of TNF-α indicates only innate immune response is activated accompanying with the repair of collagen/elastin initiate in the dermis. In contrast, the unfocused femtosecond laser can activate significant immune responses in deep dermis, indicated by the high-level IL-6, IL-17, and TGF-β. But no collagen repair or skin remodeling could be found in this case. The clinical picosecond laser showed similar results but less damage effect. Those results suggest the focused femtosecond-laser stimulation can activate repair and remodeling of skin tissue without any damage (including physical damage and immune damage) or inflammation. Therefore, focused femtosecond laser micromachining is of promising potential in skin repair and photorejuvenation.
Data Availability Statement
The original contributions presented in the study are included in the article/Supplementary Material, further inquiries can be directed to the corresponding authors
Ethics Statement
The animal study was reviewed and approved by the Animal Ethics Committee of School of Biomedical Engineering and Shanghai Ninth People's Hospital, Shanghai Jiao Tong University.
Author Contributions
HX, YZ, and HH conceived the study. YW and SW performed the experiments. HH contributed to data analysis and manuscript preparation.
Funding
The work was supported by Science and Technology Commission Shanghai Municipality 18QA1402300, and National Natural Science Foundation of China (81661168014 and 62022056).
Conflict of Interest
The authors declare that the research was conducted in the absence of any commercial or financial relationships that could be construed as a potential conflict of interest.
References
1. Yun SH, Kwok SJJ. Light in diagnosis, therapy and surgery. Nat Biomed Eng (2017) 1:1–16. doi:10.1038/s41551-016-0008
2. Alexiades-Armenakas MR, Dover JS, Arndt KA. The spectrum of laser skin resurfacing: nonablative, fractional, and ablative laser resurfacing. J Am Acad Dermatol (2008) 58:719–40. doi:10.1016/j.jaad.2008.01.003
3. Novoselova EG, Glushkova OV, Cherenkov DA, Chudnovsky VM, Fesenko EE. Effects of low-power laser radiation on mice immunity. Photodermatol Photoimmunol Photomed (2006) 22:33–8. doi:10.1111/j.1600-0781.2006.00191.x
4. Shah S, Alster TS. Laser treatment of dark skin: an updated review. Am J Clin Dermatol (2010) 11:389–97. doi:10.2165/11538940-000000000-00000
5. Hsu VM, Aldahan AS, Mlacker S, Shah VV, Nouri K. The picosecond laser for tattoo removal. Laser Med Sci (2016) 31:1733–7. doi:10.1007/s10103-016-1924-9
6. Kung K, Shek SY, Yeung CK, Chan HH. Evaluation of the safety and efficacy of the dual wavelength picosecond laser for the treatment of benign pigmented lesions in Asians. Laser Surg Med (2019) 51:14–22. doi:10.1002/lsm.23028
7. Habbema L, Verhagen R, Van Hal R, Liu Y, Varghese B. Minimally invasive non-thermal laser technology using laser-induced optical breakdown for skin rejuvenation. J Biophot (2012) 5:194–9. doi:10.1002/jbio.201100083
8. DiBernardo BE, Pozner JN. Intense pulsed light therapy for skin rejuvenation. Clin Plast Surg (2016) 43:535–40. doi:10.1016/j.cps.2016.03.008
9. Wu DC, Goldman MP, Wat H, Chan HHL. A systematic review of picosecond laser in dermatology: evidence and recommendations. Laser Surg Med (2020) [Epub ahead of print]. doi:10.1002/lsm.23244
10. Alegre-Sanchez A, Jiménez-Gómez N, Moreno-Arrones ÓM, Fonda-Pascual P, Pérez-García B, Jaén-Olasolo P, et al. Treatment of flat and elevated pigmented disorders with a 755-nm alexandrite picosecond laser: clinical and histological evaluation. Laser Med Sci (2018) 33:1827–31. doi:10.1007/s10103-018-2459-z
11. Chalermchai T, Rummaneethorn P. Effects of a fractional picosecond 1,064 nm laser for the treatment of dermal and mixed type melasma. J Cosmet Laser Ther (2018) 20:134–9. doi:10.1080/14764172.2017.1376098
12. Khetarpal S, Desai S, Kruter L, Prather H, Petrell K, Depina J, et al. Picosecond laser with specialized optic for facial rejuvenation using a compressed treatment interval. Laser Surg Med (2016) 48:723–6. doi:10.1002/lsm.22551
13. Weiss RA, McDaniel DH, Weiss MA, Mahoney AM, Beasley KL, Halvorson CR. Safety and efficacy of a novel diffractive lens array using a picosecond 755 nm alexandrite laser for treatment of wrinkles. Laser Surg Med (2017) 49:40–4. doi:10.1002/lsm.22577
14. Choi YJ, Nam JH, Kim JY, Min JH, Park KY, Ko EJ, et al. Efficacy and safety of a novel picosecond laser using combination of 1 064 and 595 nm on patients with melasma: a prospective, randomized, multicenter, split-face, 2% hydroquinone cream-controlled clinical trial. Laser Surg Med (2017) 49:899–907. doi:10.1002/lsm.22735
15. Chayavichitsilp P, Limtong P, Triyangkulsri K, Pratumchart N. Comparison of fractional neodymium-doped yttrium aluminum garnet (Nd:YAG) 1064-nm picosecond laser and fractional 1550-nm erbium fiber laser in facial acne scar treatment. Laser Med Sci (2020) 35:695–700. doi:10.1007/s10103-019-02891-5
16. Brauer JA, Reddy KK, Anolik R, Weiss ET, Karen JK, Hale EK, et al. Successful and rapid treatment of blue and green tattoo pigment with a novel picosecond laser. Arch Dermatol (2012) 148:820–3. doi:10.1001/archdermatol.2012.901
17. Tanghetti EA. The histology of skin treated with a picosecond alexandrite laser and a fractional lens array. Laser Surg Med (2016) 48:646–52. doi:10.1002/lsm.22540
18. Balu M, Lentsch G, Korta DZ, König K, Kelly KM, Tromberg BJ, et al. In vivo multiphoton-microscopy of picosecond-laser-induced optical breakdown in human skin. Laser Surg Med (2017) 49:555–62. doi:10.1002/lsm.22655
19. Chung HJ, Lee HC, Park J, Childs J, Hong J, Kim H, et al. Pattern analysis of 532- and 1064-nm microlens array-type, picosecond-domain laser-induced tissue reactions in ex vivo human skin. Laser Med Sci (2019) 34:1207–15. doi:10.1007/s10103-018-02711-2
20. Habbema L, Verhagen R, Van Hal R, Liu Y, Varghese B. Efficacy of minimally invasive nonthermal laser-induced optical breakdown technology for skin rejuvenation. Laser Med Sci (2013) 28:935–40. doi:10.1007/s10103-012-1179-z
21. Sakio R, Ohshiro T, Sasaki K, Ohshiro T. Usefulness of picosecond pulse alexandrite laser treatment for nevus of Ota. Laser Ther (2018) 27:251–5. doi:10.5978/islsm.27_18-OR-22
22. Bernstein EF, Schomacker KT, Basilavecchio LD, Plugis JM, Bhawalkar JD. Treatment of acne scarring with a novel fractionated, dual-wavelength, picosecond-domain laser incorporating a novel holographic beam-splitter. Laser Surg Med (2017) 49:796–802. doi:10.1002/lsm.22734
23. Vogel A, Noack J, Hüttman G, Paltauf G. Mechanisms of femtosecond laser nanosurgery of cells and tissues. Appl Phys B (2005) 81(8):1015–47. doi:10.1007/s00340-005-2036-6
24. Chung SH, Mazur E. Surgical applications of femtosecond lasers. J Biophotonics (2009) 2:557–72. doi:10.1002/jbio.200910053
Keywords: femtosecond laser, micromachining, skin, immune response, clinic application
Citation: Wang Y, Wang S, Zhu Y, Xu H and He H (2021) Molecular Response of Skin to Micromachining by Femtosecond Laser. Front. Phys. 9:637101. doi: 10.3389/fphy.2021.637101
Received: 02 December 2020; Accepted: 11 January 2021;
Published: 16 February 2021.
Edited by:
Chao Tian, University of Science and Technology of China, ChinaReviewed by:
Zhichao Fan, UCONN Health, United StatesHong Leng, Soochow University, China
Cheng Lei, Wuhan University, China
Copyright © 2021 Wang, Wang, Zhu, Xu and He. This is an open-access article distributed under the terms of the Creative Commons Attribution License (CC BY). The use, distribution or reproduction in other forums is permitted, provided the original author(s) and the copyright owner(s) are credited and that the original publication in this journal is cited, in accordance with accepted academic practice. No use, distribution or reproduction is permitted which does not comply with these terms.
*Correspondence: Yujie Zhu, zyjfd@163.com; Hui Xu, 2201691904@qq.com
†These authors have contributed equally to this work