- 1Prokhorov General Physics Institute of the Russian Academy of Sciences, Moscow, Russia
- 2All-Russian Phytopathology Research Institute, Big Vyazyomy, Russia
- 3Institute of Fertility of Soils of South Russia, Persianovka, Rostov Region, Russia
- 4Federal State Budgetary Scientific Institution “Federal Scientific Agroengineering Center VIM” (FSAC VIM), Moscow, Russia
This study aimed to develop a sustainable industrial chemical engineering technology to improve the interaction between technology, plants, and soil in agriculture. The signaling crosstalk between H2O2 and NO and that between H2O2 and Ca2+ influence plant developmental and physiological processes. Many promising technologies for crop stimulation and protection are based on a thorough study of the environmental impact of various physical factors. A low-temperature, high-frequency plasma was generated via cathode high-frequency glow discharge and used for the electrolysis of an aqueous solution of a low concentration of the strong electrolyte KH2PO4, with an electrolysis-activated solution named Plasmolite. The Plasmolite solution yielded a Raman (red) scattering spectrum with a maximum at 1,640 cm−1, which was associated with hydrogen atom vibrations, and other bands at 875, 930, 1,050, and 1,123 cm−1, which were associated with the aqueous electrolyte solution plasma treatment. Based on the goal of producing an optimal H2O2 concentration of 100 μM, two types of seeds were exposed to a Plasmolite-based 2 × 10–5 M KH2PO4 solution moisturizing medium for three days. Approximately 92% of the spring spelled seeds (grade “Gremme”) that were exposed to this test solution sprouted, compared with 76% of the seeds exposed to a control solution. The spring rye seeds (grade “Onokhoyskaya”) that were exposed to the test solution sprouted at a rate of 90% compared with 75% in the control. The percentage of seeds that sprouted with a root length of more than 6 mm was approximately 80% for the test solution, compared to 50% for the control. Based on these results, the use of Plasmolite is considered to be promising for the production of activated H2O2 for protecting plants and stimulating growth, particularly for enhancing the functions of K and P2O5 of fertilizers.
Introduction
Soil and plant health and productivity are crucial for sustainable development [1–4]. Agricultural landscapes and soils can be altered due to the fact that farmers and agribusinesses fail to take advantage of up-to-date technologies in sustainable chemical engineering, which provide long-term soil and plant stability and productivity [5]. Alterations in the soil can cause the uncontrolled transfer of solutions [6], the degradation of organic matter [7], limiting the potential use of humic substances (HSs) to stimulate remediation of soil [8], and restricting polymicrobial association to the initiate biological processes in soil [9]. The increasing use of standard toxic agro-chemicals has led to negative environmental effects [10]. Chemical conversions of agro-chemicals and interactions between agro-chemicals are also often dangerous. Most agricultural land across the world is in at-risk farming zones, and it is necessary to use anti-stress regulators to enable ongoing successful agriculture.
Promising technologies for crop stimulation and protection are based on a thorough dosed physical factor environmental impact [11]. Special attention should be paid to the use of small amounts of environmentally safe and low-cost stimulators. It is important to attempt to administer stimulants as close as possible to the target area [10–12]. Hydrogen peroxide (H2O2) is a reactive oxygen species and serves as an important plant stimulator. It is found in nanomolar to low-micromolar concentrations in the environment [13–15] and is generated widely in biological systems [16, 17].
Changes in H2O2 levels may impact metabolic and antioxidant enzyme activities, encouraging plant growth and stress tolerance [18–20]. Signaling crosstalks between H2O2 and NO and between H2O2 and Ca2+ have been shown to influence seed germination, photosynthesis, root growth, and stomatal closure modulation [18–21]. However, the mechanisms that allow for the different functions of H2O2 in plants still need to be determined [22].
Pre-sowing seed treatment, foliar plant treatment, and soil treatment using solutions with a low concentration of H2O2 have been shown to increase agricultural plant tolerance to stress [17], resistance to pathogens [23], antioxidant activities [24], and the starch content in leaves and fruits [25]. Soaking seeds in solutions made up of various concentrations of H2O2 has been shown to enhance stomata density, increase the length and quantity of the histological components of leaves and roots [26–30], and reduce fungi infestation in seeds [31]. The concentration of H2O2 used in these treatments, however, needs to be controlled to avoid harmful effects. For example, the application of 5% H2O2 has been sh wn to destroy 90% of organic matter in soil [32]. Seeds taking in 10–100 mM H2O2 at 25 or 29 °C were observed to germinate in three days, but higher H2O2 concentrations were inhibitory, and applying H2O2 at concentrations of 1,000 and 3,000 mM fully inhibited germination [33]. The growth of Arabidopsis has been inhibited by applications of 1 mM H2O2 [34]. In mammals and yeast, H2O2 toxicity occurs at low-millimolar, but not micromolar, concentrations [35–37].
The specific targets of O2− and H2O2 toxicity in plants have not been extensively studied and their effects are often obscured under the blanket term “oxidative stress”. The technology of H2O2 production, treatment dosage, duration, and timing is of vital importance for implementation. A promising field of research and practice is the use of electrolysis to achieve biological activation in aqueous solutions. The use of KH2PO4 at concentrations from 10 mM to 1 M as the supporting electrolyte is more effective for the electrolytic production of hydrogen than the use of a solution of 25 wt% KOH [38]. The electrolytic method for preparing potassium dihydrogen phosphate [39] allowed the electrolysis of 1–8 M KH2PO4 to be carried out.
Various methods and devices for producing activated water using electrochemical methods have been described [40]. For example, nonequilibrium low-temperature plasma, formed in water vapor in contact with an aqueous electrolyte, has been shown to generate reactive oxygen and nitrogen [12, 16, 17]. It is important to note that active forms of oxygen and nitrogen strongly influence biological processes [20, 41].
The high variability of the sources and doses of H2O2 in different experiments show a strong need for carrying out new studies on the production of H2O2 and its application to plants and soil.
High-frequency low-temperature plasma generated via cathode high-frequency glow discharge can be used to perform electrolysis on an aqueous solution of a strong electrolyte KH2PO4 at low concentration. This Plasmolite is an electrolysis-activated aqueous solution of a controlled concentration and offers an environmentally friendly stimulator of plant growth and development [42], while also acting as a remediation substance [43]. The Plasmolite production process is fundamentally different than traditional methods of water activation. Plasmolite is produced in a plasma-chemical reactor by the action of a non-equilibrium plasma of a high-frequency glow discharge and plasma cathode electrolysis.
Glow discharge occurs in water vapor between the metal cathode and the electrolyte surface (anode). In this case, the electrolyte is exposed to charged particles (electrons, negative ions) and neutral molecules. Electrochemical action occurs during electrolysis between the metal anode and the plasma cathode (plasma-electrolyte interface). The use of a plasma cathode shows an ion recharge process different to that shown by the use of a metal cathode. Electrolyte solutions of potassium salts of nitric or phosphoric acid are used. It is possible to change Plasmolite properties such as pH, redox potential, and H2O2 concentration by choosing the material of the glow discharge cathode, the composition of the electrolyte, and the duration of exposure. The H2O2 concentration is usually 10−3 M. The high concentration of H2O2 in Plasmolite makes it beneficial in agriculture when diluted 100 to 1,000 fold. It is also diluted with distilled water for use in experimental studies on animals and plants. Low-temperature plasma affects the proliferative activity of cells and the repair functions of biological tissues in animals and plants [44]. Plasmolite has been developed in the Prokhorov General Physics Institute of the Russian Academy of Sciences (RAS) [11, 45]. There have been promising results for the electrolytic production of activated H2O2 in plant stimulation and protection, and particularly for enhancing the functions of K and P2O5 of fertilizers.
Farming processes that stimulate plants involve risks and it is necessary to correct the conditions of plant organogenesis. Many older methods that are still in use in agriculture, including irrigation and remediation technologies, are incapable of creating stable responses in agrobiogeosystems to plant stimulation. To ensure the maintenance of continuous soil architecture and a stable supply of moisture for sustainable plant organogenesis, the use of the biogeosystem technique (BGT) methodology has been proposed [2]. BGT-based sustainable chemical engineering technology has a synergistic effect on soil and plant stimulation, achieving long-term soil stability and plant productivity [46, 47].
The current study used an aqueous solution made up of a low concentration of the strong electrolyte KH2PO4 to activate sprouting in plant seeds. The KH2PO4 solution was produced via electrolysis using the high-frequency low-temperature plasma of a cathode glow discharge (Plasmolite). We propose that this approach be used as a constituent part of future BGT sustainable chemical engineering in the field of agriculture. We also developed a robotic device that undertakes continuous intra-soil pulse discrete watering while simultaneously supplying the H2O2 Plasmolite.
Materials and Methods
We studied the germination of seeds exposed to an electrolytically activated aqueous solution [38, 39]. Electrolysis was performed using high-frequency low-temperature plasma of a cathode glow discharge. The process was developed in the Prokhorov General Physics Institute of the RAS [40]. The process and the activated aqueous solution as a product of the process were each named Plasmolite. We positioned the Plasmolite as a plant growth stimulator.
The active electrode used was a platinum wire with a diameter of 0.5 mm. The passive electrode was a rod of PGI brand pyrolytic graphite. A voltage of 370 V with a frequency of 110 kHz was applied to the electrodes. The electrolyte was constantly mixed using a magnetic stirrer. The “electrode–solution” layer contact matter was refreshed, and the solution was maintained at a uniform temperature. The temperature of the electrolyte was controlled using forced air cooling. The solution temperature was maintained between 50°C and 55°C.
An oscillogram was taken of the active electrode. Raman spectroscopy deploying red light and a U1000 spectrometer (Renishaw, United Kingdom) was used to study the Plasmolite solution. An argon laser, with a radiation line of 514.53 nm, was used for excitation. The Plasmolite redox potential and acidity were measured.
The Plasmolite activation limit was the 100 μM H2O2 concentration in the electrolyte. The H2O2 concentration was determined using the iodometry method. The redox titration was used for determining the oxidizing agent concentration in the solution [48]. Two experiments were carried out to study the use of Plasmolite to stimulate plant germination.
The seeds were soaked in an aqueous solution that included a low concentration of the strong electrolyte KH2PO4 with H2O2 content (Plasmalite) [22, 28–30, 49]. We avoided the use of an inhibitory dose of H2O2 [33].
Experiment 1
Seeds of spring spelled grade “Gremme” were germinated in a Petri dish on a soaked paper filter. A solution of 2 × 10–5 M KH2PO4 was used for paper towel soaking as the control condition. The Plasmolite stimulating solution was used in the experimental condition. Before carrying out the soaking, the Plasmolite solution was diluted with distilled water in a ratio of 1–500 to achieve a KH2PO4 concentration of 2 × 10–5 M.
The Petri dishes with seeds were kept in a thermostat at a temperature of +20°C. The paper towel was kept wet by adding the 2 × 10–5 M KH2PO4 solution (control) and the Plasmolite stimulating solution diluted with distilled water in a ratio of 1–100, every 12 h. The experiment was carried out for three days. We then calculated the percentage of seeds that germinated.
Experiment 2
Spring rye seeds grade “Onokhoyskaya” were germinated in a Petri dish on a paper towel soaked with 2 × 10–5 M KH2PO4 (control condition), and with Plasmolite stimulating solution. Before carrying out the soaking, the Plasmolite solution was diluted with distilled water in a ratio of 1–500.
The Petri dishes with seeds were kept in a thermostat at a temperature of +20°C. The paper towel was kept wet by adding the 2 × 10–5 M KH2PO4 solution (control), and the Plasmolite stimulating solution was diluted with distilled water in a ratio of 1–100, every 12 h.
Experiment 2 was also carried out for three days and we calculated the percentage of seeds that sprouted. The percentage of seedlings with root lengths of more than 6 mm were also recorded.
Results
The acquired active electrode oscillogram is presented in Figure 1 [50]. Raman (red) scattering spectrum of the Plasmolite solution was also obtained. The scattering maximum was at 1,640 cm−1, and bands at 875 cm−1, 930 cm−1, 1,050 cm−1, and 1,123 cm−1 were also observed previously [16]. The Plasmolite redox potential ranged from −1,000 mV to +1,500 mV, and its pH ranged from 3 to 12 during its synthesis.
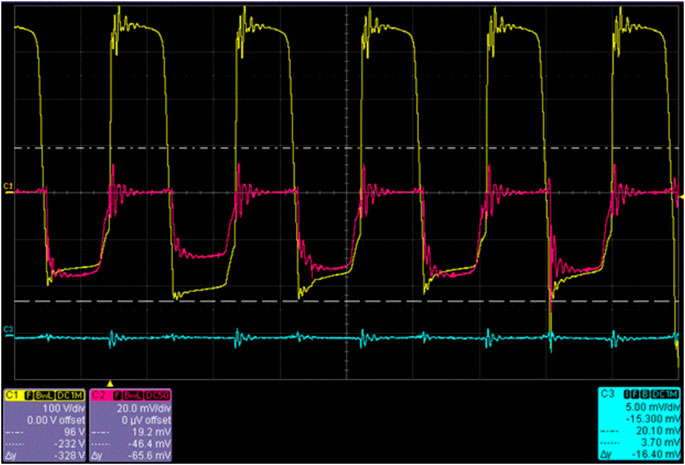
FIGURE 1. Voltage (yellow curve), current (red curve), and plasma glow (blue curve) oscillograms of the active electrode.
The Plasmolite solution was stored in the dark at a temperature of +20°C. The solution activation level was characterized by the H2O2 content, determined using the iodometry method. The concentration of H2O2 in the solution was 6 × 10–5 M on the first and third days after the production of the Plasmolite solution, and 3 × 10–5 M on the sixth day.
The plant germination results for Experiment 1 are presented in Table 1 and Figure 2. The percentage of grade “Gremme” spring spelled seeds that germinated three days after the beginning of seed germination was higher when fresh Plasmolite moisturizing medium was used than when the control moisturizing medium was used (Table 1). These seeds were also observed to be better developed three days after the start of the seed germination on a soaked paper towel in a Petri dish at a temperature +20°C when the Plasmolite medium was used (Figure 2B) than when the control was used (Figure 2A).
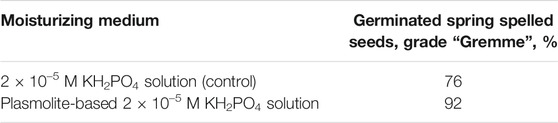
TABLE 1. Percents of spring spelled seeds, grade “Gremme”, that germinated three days after the beginning of seed germination for the two moisturizing mediums tested.
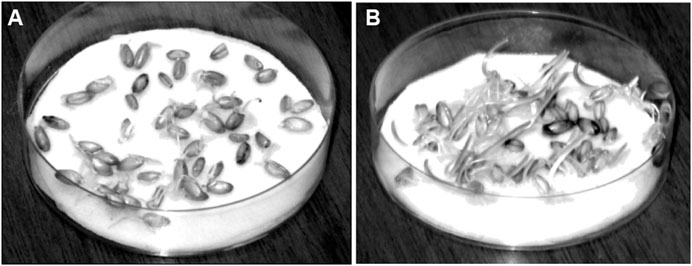
FIGURE 2. Spring spelled seedling, grade “Gremme”, three days after the beginning of seed germination on a soaked paper towel in a Petri dish at a temperature of +20 °C (A) Paper towel soaked with a control 2 × 10–5 M KH2PO4 solution; (B) Paper towel soaked with a Plasmolite-based 2 × 10–5 M KH2PO4 solution.
The results of plant germination for Experiment 2 are presented in Table 2. A greater percentage of the grade “Onokhoyskaya” spring rye seeds developed root systems with root lengths of more than 6 mm three days after the beginning of the seed germination when the Plasmolite medium was used than when the control was used.

TABLE 2. Percents of spring rye seeds, grade “Onokhoyskaya”, that developed root systems three days after the beginning of the seed germination on a soaked paper towel in a Petri dish at a temperature of +20°C for the two moisturizing mediums tested.
The data for Experiments 1 and 2 showed the positive role of Plasmolite as a plant growth stimulant. However, no appropriate technology is currently capable of producing enough Plasmolite to meet the practical needs of large scale agriculture. There are also currently no effective devices for applying Plasmolite to the soil. We propose the use of BGT methodology involving an intra-soil pulse continuous-discrete watering robotic system [2, 51, 52], which could simultaneously water and add the stimulant to the soil (Figure 3).
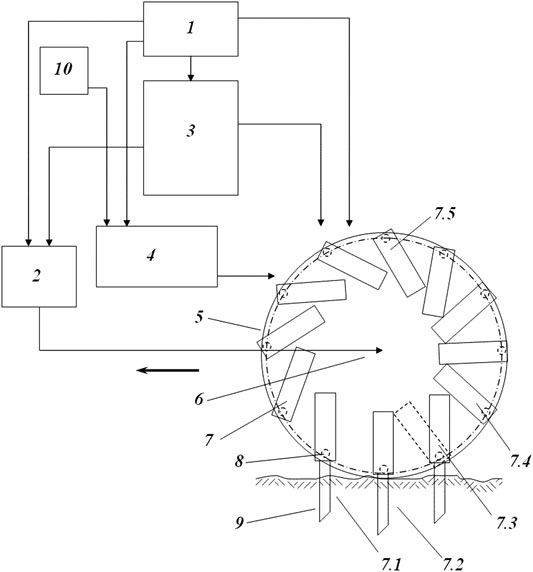
FIGURE 3. Robotic device for carrying out intra-soil pulse continuous-discrete watering and at the same time supplying the soil with Plasmolite H2O2. Power supply unit 1, wheel chassis unit 2, control unit 3, water supply unit 4, disc 5, axis 6, syringe element 7, syringe element positions 7.1–7.5, flexible supply coupling 8, retractable syringe 9, Plasmolite H2O2 (and/or other substances) supply unit 10.
Discussion
Properties of the Plasmolite Solution
Based on the voltage, current, and the plasma glow oscillograms of the active electrode (Figure 1), the results of the present study indicate that electrolysis of the aqueous solution of strong electrolyte KH2PO4 can proceed actively at a low electrolyte concentration (0.01 M).
The Raman (red) scattering spectrum maximum at 1,640 cm−1 of the Plasmolite solution was associated with hydrogen atoms vibrations. The scattering spectrum bands at 875, 930, 1,050, and 1,123 cm−1 were associated with the treatment of aqueous electrolyte solution with plasma.
The Plasmolite solution redox potential ranged from −1,000 mV to +1,500 mV and its pH ranged from 3 to 12 during its synthesis. These data indicate a high amount of plasma cathode electrolysis [40–44, 53].
As a plasma-forming gas, the glow discharge in water vapor initiates the plasma-chemical decomposition of a water molecule. Free electron dissociative adhesion in the plasma leads to the formation of the negatively charged H2O−ion. The water molecule decomposes when the electron gas temperature is higher than 1.5–3.5 eV [40]. The overall reaction can be described using the equations:
Where M is a molecule (ion) playing the role of a third body necessary for the progress of the reaction. The reaction starts with an electron impact. The chain process includes a dissociative attachment reaction that increases the effectiveness of the process [44, 53]. One H2O2 molecule is synthesized per molecule of hydrogen released.
The decrease in Plasmolite solution H2O2 concentration from 6 × 10–5 M on the first day after Plasmolite production to 3 × 10–5 M on the sixth day as described in the present study reflected a reduction in Plasmolite solution activity over time. The seed and/or plant should be treated with the Plasmolite solution immediately after it is produced, or better yet while it is being produced.
The dual nature of H2O2 as a toxic molecule on the one hand, and as a signal molecule on the other, ensures its ability to exercise precise spatial and temporal control on the production and degradation of Plasmolite H2O2. The levels of the H2O2 concentration in germinating seeds and young seedlings can be modulated via pre-sowing seed treatment [54].
As described in the Results above, a higher percentage of grade “Gremme” spring spelled seeds (in Experiment 1) germinated when they were soaked in the Plasmolite-based 2 × 10–5 M KH2PO4solution experimental moisturizing medium than when they were soaked in the 2 × 10–5 M KH2PO4 solution used as the control. The number of grade “Onokhoyskaya” spring rye seeds (in Experiment 2) that sprouted was 20% higher in the 2 × 10−5 M KH2PO4-based Plasmolite solution than in the control 2 × 10−5 M KH2PO4 solution (Table 1), and the percentage of sprouted seeds with a root length of more than 6 mm was 60% higher when Plasmolite was used than when the control medium was used (Table 2). These data indicate the high biological efficiency of Plasmolite as a plant organogenesis stimulant [24, 26]. The H2O2 concentration in the Plasmolite was extremely low compared to that of standard H2O2 used to stimulate seeds and plants in previous research [25, 28].
These results indicated that the Plasmolite solution distinctly influenced the seeds in a way different from the influence of standard H2O2 on seeds. The standard H2O2 concentration of 100 mM stimulates seed germination [28, 33]. In contrast, the Plasmolite solution inhibits plant germination even at a 100-fold lower H2O2 concentration of 1 mM [16]. The H2O2 concentration in Plasmolite is similar to the concentration of H2O2 in coastal terrain rainwater [14, 15].
We expect future studies to improve understanding of this phenomenon. The low active concentration of Plasmolite is promising because a small reagent volume is more suitable for the development of a combined robotic system. This potential system could be used for watering, stimulating, and protecting plants and to achieve better conditions for the functioning of the HSs and polymicrobial biofilms in the soil.
The pretreatment of seeds and stimulation of plants with H2O2 has been shown to positively influence plant productivity in agriculture [33], horticulture [25], and other spheres of biological activity. The positive impact of H2O2 treatment has been attributed to its suppression of pathogen activity and reduction of seed infestation with fungi [31, 32]. Other studies have indicated that treating plants with H2O2 increases the antioxidant defense of plants and improves their tolerance to different stresses [22]. The results of the present study are promising not only for the production of H2O2 via electrolysis for the stimulation and protection of plants, but they also provide insights into the potential functions of Plasmolite as a fertilizer that contains electrolytically activated K and P2O5.
Plant organogenesis can be orchestrated by applying H2O2 from early seedling growth up to harvesting [30]. Analysis of the growth and development of seedlings (Figure 2) at different stages of morphogenesis indicated their dependence on the amount of H2O2 in the activated solution used. Plant and soil health can be improved depending on the H2O2 dose used [31, 55].
The effect of H2O2 on plant physiology and the biological processes in the soil are synergistic [22]. To fully achieve this effect, and achieve the full direct and indirect potential of H2O2, it is important to improve the functioning of the soil organic matter (SOM), dissolved organic matter (DOM), HSs, and polymicrobial biofilms in the soil. The intra-soil injection of liquid fertilizer provides agronomical advantages [56, 57]. This approach is beneficial for protecting plants and watering the soil.
We propose the use of the biogeosystem technique (BGT) methodology [52] for applying the Plasmolite H2O2 and realizing its potential to stimulate plants and biological processes in the soil. Bacteria are the main microbial representatives in the rhizosphere. Multiple mechanisms have been identified for the stimulation of plant growth by rhizobacteria [12]. Plant organogenesis and productivity, however, face soil biology limitations, namely connected to the conditions for rhizosphere development.
Plasmolite Solution Plant Stimulation Technology Based on the Biogeosystem Technique Methodology
Currently, the available structures and architectures that are used in soil geophysical systems [58, 59] are inappropriate for rhizobacteria and the synthesis of soil organic matter [60], soil humeome functions [61], and plant growth. Soil compaction and illuviation are biological stress factors that cause a low tolerance to the environment in plants. A fine multilevel soil aggregate system [62, 63] can be provided by carrying out intra-soil milling on top of 20–45 cm of soil. This procedure improves the biological capabilities of the soil [64]. New abundant soil interfaces resulting from intra-soil milling [65] ensure a high rate of occurrence of biological processes in the multilevel soil architecture system, and would increase the biological potential of Plasmolite H2O2. Another BGT methodology involves the intra-soil pulse continuous-discrete watering paradigm [2]. The use of an intra-soil pulse continuous-discrete robotic watering system provides the possibility to simultaneously supply Plasmolite H2O2 to the soil. Performing intra-soil pulse continuous-discrete watering has been shown to yield matrix potentials of −0.2 to −0.4 MPa, values much lower than those resulting from standard irrigation, but at the same time a priority for plant and soil biome organogenesis. This discrete intra-soil watering is vital for Plasmolite H2O2 function because there would be no need to supply excess H2O2 to compensate for the loss of water from the soil to the vadose zone since this would not occur. New BGT technology could also be used to address the issues resulting from an excessive supply of water, which is itself a dangerous plant stress-factor that can become a problem in standard irrigation.
Intra-soil pulse continuous-discrete robotic watering could provide an optimal amount of Plasmolite H2O2 for plant growth stimulation. This method could be beneficial in achieving an improved turnover of the soil matter since this type of watering strictly controls the spread of water throughout the soil. As indicated in the present study and shown in Figure 3, this type of simultaneous water and Plasmolite H2O2 supply is possible. It is anticipated that the robotic device, our patent, and current research [2, 51] will provide a basis for developing a sustainable chemical engineering technology for soil watering, plant and soil stimulation, and plant protection. The Plasmolite H2O2 and/or other substances can be supplied effectively, ensuring the proper management of plant organogenesis when the amount of water in the soil is sufficient for plant growth. The robotic system provides a synergistic effect of Plasmolite H2O2, fertilization, soil organic matter [7], HSs [66], polymicrobial biofilm starters [9], high rates of soil biological process, and high soil productivity [55].
The mechanisms of the different functions of H2O2 in plants still need to be determined [22]. This task becomes more complicated when accounting for the new data provided by this study and the BGT* methodology. Nevertheless, seed and plant stimulation with H2O2 is of high importance, because the signaling crosstalk between H2O2 and NO and that between H2O2 and Ca2+ are crucial for plant developmental and physiological processes at every stage of plant organogenesis.
Conclusion
The biologically activated product Plasmolite was produced by carrying out electrolysis of an aqueous solution of a low concentration (0.01 M) of the strong electrolyte KH2PO4. Electrolysis was achieved by using a high-frequency low-temperature plasma of a cathode glow discharge.
The application of a solution of Plasmolite KH2PO4 with a concentration of 2 × 10–5 M yielded higher percentages of seeds that germinated and that sprouted, with root lengths of more than 6 mm than a control solution that used 2 × 10–5 M KH2PO4 as the moisturizing medium. The concentration of the activation agent H2O2 was 6 × 10–5 M. The obtained data indicate that the Plasmolite solution serves as a plant growth activator.
This study also proposes a robotic device that can perform intra-soil pulse continuous-discrete watering and, at the same time, supply Plasmolite-activated H2O2 to the soil. This provides a sustainable industrial chemical engineering technology for achieving long-term productivity and ensuring the health of soils and plants.
Data Availability Statement
The raw data supporting the conclusions of this article will be made available by the authors, without undue reservation.
Author Contributions
SVB: Writing–Original Draft, Investigation; YD: Conceptualization, Investigation, Writing–Original Draft, Supervision; AG: Resources; VK: Validation, Writing–Review & Editing; AE: Investigation, Methodology; VS: Investigation, Formal analysis, Data Curation, Software; EK: Formal analysis, Data Curation; SVG: Resources, Funding acquisition; AD: Funding acquisition, Formal analysis; YL: Conceptualization, Data Curation; AI: Conceptualization, Project administration.
Funding
This work was supported by a grant from the Ministry of Science and Higher Education of the Russian Federation for large scientific projects in priority areas of scientific and technological development (grant number 075-15-2020-774).
Conflict of Interest
The authors declare that the research was conducted in the absence of any commercial or financial relationships that could be construed as a potential conflict of interest.
Supplementary Material
The Supplementary Material for this article can be found online at: https://www.frontiersin.org/articles/10.3389/fphy.2020.618320/full#supplementary-material.
References
1. Gudkov SV, Andreev SN, Barmina EV, Bunkin NF, Kartabaeva BB, Nesvat AP, et al. Effect of visible light on biological objects: physiological and pathophysiological aspects. Phys Wave Phenom (2017) 25:207–13. doi:10.3103/S1541308X17030074
2. Gudkov SV, Grinberg MA, Sukhov V, Vodeneev V. Effect of ionizing radiation on physiological and molecular processes in plants. J Environ Radioact (2019) 202:8–24. doi:10.1016/j.jenvrad.2019.02.001
3. Keesstra SD, Bouma J, Wallinga J, Tittonell P, Smith P, Cerdà A, et al. The significance of soils and soil science towards realization of the United Nations Sustainable Development Goals. Soils (2016) 2:111–28. doi:10.5194/soil-2-111- 2016
4. Van Mansvelt J-D. Soil fertility in agriculture: Russia–Western Europe–USA: in the past and today. Biogeosyst Tech (2017) 4:220–31. doi:10.13187/bgt.2017.2.220
5. Gudkov SV, Shafeev GA, Glinushkin AP, Shkirin AV, Barmina EV, Rakov AV, et al. Production and use of selenium nanoparticles as fertilizers. ACS Omega (2020) 5(28):17767–74. doi:10.1021/acsomega.0c02448
6. Endovitsky AP, Batukaev AA, Minkina TM, Kalinitchenko VP, Mandzhieva SS, Sushkova SN, et al. Ions association in soil solution as the cause of lead mobility and availability after application of phosphogypsum to chernozem. J Geochem Explor (2017) 182:185–92. doi:10.1016/j.gexplo.2016.08.018
7. Kalinichenko VP, Glinushkin AP, SokolovZinchenko MSVE, Minkina TM, Mandzhieva SS, Sushkova SN, et al. Impact of soil organic matter on calcium carbonate equilibrium and forms of Pb in water extracts from Kastanozem complex. J Soils Sediments (2018) 19(6):2717–28. doi:10.1007/s11368-018-2123-z
8. Pukalchik MA, Panova MI, Karpukhin MM, Yakimenko OS, Kydralieva KA, Terekhova VA. Using humic products as amendments to restore Zn and Pb polluted soil: a case study using rapid screening phytotest endpoint. J Soils Sediments (2018) 18(3):750–61. 10.1007/s11368-017-1841-y
9. Swidsinski A. The colonic bioreactor–a forerunner model for future biotechnology (function, role, products and management). In: Fifth international conference of CIS IHSS on humic innovative technologies “humic substances and living systems; 2019 October 19–23, Moscow, Russia: NT Press (2019). p. 22.
10. Mann RM, Hyne RV, Choung CB, Wilson SP. Amphibians and agricultural chemicals: review of the risks in a complex environment. Environ Pollut (2009) 157:2903–27. doi:10.1016/j.envpol.2009.05.015
11. He K, Zhang J, Wang X, Zeng Y, Zhang L. A scientometric review of emerging trends and new developments in agricultural ecological compensation. Environ Sci Pollut Res Int (2018) 25:16522–32. doi:10.1007/s11356-018-2160-6
12. Matilla MA, Krell T. Plant growth promotion and biocontrol mediated by plant-associated bacteria. In: D Egamberdieva, and P Ahmad, editors. Plant microbiome: stress response. Microorganisms for sustainability. Singapore, Asia: Springer (2018). p. 45–80.
13. Li X, Imlay JA. Improved measurements of scant hydrogen peroxide enable experiments that define its threshold of toxicity for Escherichia coli. Free Radic Biol Med (2018) 120:217–27. doi:10.1016/j.freeradbiomed.2018.03.025
14. Deng Y, Zuo Y. Factors affecting the levels of hydrogen peroxide in rainwater. Atmos Environ (1999) 33(9):1469–78. doi:10.1016/S1352-2310(98)00239-8
15. Willey JD, Kieber RJ, Lancaster RD. Coastal rainwater hydrogen peroxide: concentration and deposition. J Atmos Chem (1996) 25:149–65. doi:10.1007/BF00053789
16. Apasheva L, Danyleiko Y, Egorov A, Korshunov A, Demin D, Sidorov V, et al. Activation of aqueous solutions by high-frequency glow discharge plasma in water vapor to stimulate growth and control diseases of agricultural plants. IOP Conf Ser Earth Environ Sci (2019) 390:012027. doi:10.1088/1755-1315/390/1/012027
17. Leon J, Lawton MA, Raskin I. Hydrogen peroxide stimulates salicylic acid biosynthesis in tobacco. Plant Physiol (1995) 108(4):1673–8. doi:10.1104/pp.108.4.1673
18. Liu J, Macarisin D, Wisniewski M, Sui Y, By S, Norelli J, et al. Production of hydrogen peroxide and expression of ros-generating genes in peach flower petals in response to host and non-host fungal pathogens. Plant Pathol (2013) 62:820–8. doi:10.1111/j.1365-3059.2012.02683.x
19. Vavilala SL, Gawde KK, Sinha M, D'Souza JS. Programmed cell death is induced by hydrogen peroxide but not by excessive ionic stress of sodium chloride in the unicellular green alga Chlamydomonas reinhardtii. Eur J Phycol (2015) 50:422–38. doi:10.1080/09670262.2015.1070437
20. Gudkov SV, Chernikov AV. Bruskov VI. Chemical and radiological toxicity of uranium compounds. Russ J Gen Chem (2016) 86:1531–8. doi:10.1134/S1070363216060517
21. Shi K, Li X, Zhang H, Zhang G, Liu Y, Zhou Y, et al. Guard cell hydrogen peroxide and nitric oxide mediate elevated CO2 -induced stomatal movement in tomato. New Phytol (2015) 208:342–53. doi:10.1111/nph.13621
22. Niu L, Liao W. Hydrogen peroxide signaling in plant development and abiotic responses: crosstalk with nitric oxide and calcium. Front Plant Sci (2016) 7:230. doi:10.3389/fpls.2016.00230
23. Mukherjee M, Larrimore KE, Ahmed NJ, Bedick TS, Barghouthi NT, Traw MB, et al. Ascorbic acid deficiency in Arabidopsis induces constitutive priming that is dependent on hydrogen peroxide, salicylic acid, and the NPR1 gene. Mol Plant Microbe Interact (2010) 23:340–51. doi:10.1094/MPMI-23-3-0340
24. Ismail SZ, Khandaker MM, Mat N, Boyce AN. Effects of hydrogen peroxide on growth, development and quality of fruits: a review. J Agron (2015) 14:331–6. doi:10.3923/ja.2015.331.336
25. Ozaki K, Uchida A, Takabe T, Shinagawa F, Tanaka Y, Takabe T, et al. Enrichment of sugar content in melon fruits by hydrogen peroxide treatment. J Plant Physiol (2009) 166(6):569–78. doi:10.1016/j.jplph.2008.08.007
26. Ozaki T, Zarea MJ. Hydrogen peroxide affects plant growth promoting effects of Azospirillum. J Crop Sci Biotechnol (2016) 19:167–75. doi:10.1007/s12892-015-0127-4
27. Richards SL, Laohavisit A, Mortimer JC, Shabala L, Swarbreck SM, Shabala S, et al. Annexin 1 regulates the H2O2-induced calcium signature in Arabidopsis thaliana roots. Plant J (2014) 77:136–45. doi:10.1111/tpj.12372
28. Barba-Espín G, Hernández JA, Diaz-Vivancos P. Role of H₂O₂ in pea seed germination. Plant Signal Behav (2012) 7(2):193–5. doi:10.4161/psb.18881
29. Gondim FA, Gomes-Filho1 E, Lacerda CF, Prisco1 JT, Neto ADA, Marques EC. Pretreatment with H2O2 in maize seeds: effects on germination and seedling acclimation to salt stress. Braz J Plant Physiol (2010) 22(2):103–12. 10.1590/S1677-04202010000200004
30. Espin GB, Díaz-Vivancos P, Clemente-Moreno MJ, Faize M. Hydrogen peroxide as inductor of seed germination: effect on antioxidative metabolism and plant hormone contents in pea seedlings. Acta Horticu (2010) 898:229–36. doi:10.17660/ActaHortic.2011.898.28
31. Szopińska D. Effects of hydrogen peroxide treatment on the germination, vigour and health of Zinnia elegans seeds. Folia Hortic (2014) 26(1):19–29. doi:10.2478/fhort-2014-0002
32. Mikutta R, Kleber M, Kaiser K, Jahn R. Review: organic matter removal from soils using hydrogen peroxide, sodium hypochlorite, and disodium peroxodisulfate. Soil Sci Soc Am J (2005) 69:120–35. doi:10.2136/sssaj2005.0120
33. Dufková H, Berka M, Luklová M. Eggplant germination is promoted by hydrogen peroxide and temperature in an independent but overlapping manner. Molecules (2019) 24:4270. doi:10.3390/molecules24234270
34. Claeys H, Van Landeghem S, Dubois M, Maleux K, Inzé D. What is stress? Dose-Response effects in commonly used in Vitro stress assays. Plant Physiol (2014) 165:519–27. doi:10.1104/pp.113.234641
35. Nakamura J, Purvis ER, Swenberg JA. Micromolar concentrations of hydrogen peroxide induce oxidative DNA lesions more efficiently than millimolar concentrations in mammalian cells. Nucleic Acids Res (2003) 31:1790–5. doi:10.1093/nar/gkg263
36. Semchyshyn HM, Valishkevych BV. Hormetic effect of H2O2 in Saccharomyces cerevisiae: involvement of TOR and glutathione reductase. Dose Response (2016) 14:1559325816636130–12. doi:10.1177/1559325816636130
37. Belosludtsev KN, Belosludtseva NV, Dubinin MV. Diabetes mellitus, mitochondrial dysfunction and Ca2+-dependent permeability transition pore. Int J Mol Sci (2020) 21:6559. doi:10.3390/ijms21186559
38. De Silva M, Bergel A, Féron D, Basséguy R. Hydrogen production by electrolysis of a phosphate solution on a stainless steel cathode. Int J Hydrogen Energy (2010) 35(16):8561–8. doi:10.1016/J.IJHYDENE.2010.05.101
39. Yang L, Gou P, Zhang Z, Wang X, inventors , Sichuan University , et al. Electrolytic method for preparing potassium dihydrogen phosphate. China patent CN 102732906A (2012).
40. Baburin NV, Belov SV, Danyleiko Yu K, Egorov AV, Lebedeva T, Nefedov SM, et al. Heterogeneous recombination in water vapor plasma. Rep Acad Sci (2009) 426(4):468–70. doi:10.1007/s10527-020-09953-x
41. Niedre M, Ptterson MS, Wilson BC. Direct near-infrared luminescence detection of singlet oxygen generated by photodynamic therapy in cells in vitro and tissues in vivo. Photochem Photobiol (2002) 75(4):382–91. doi:10.1562/0031-8655(2002)075<0382:DNILDO>2.0.CO;2
42. WilsonPatterson AV, Rubtsova NA, Vedeneeva YA, Komissarov GG. Photocatalytic activity of chlorophyll in hydrogen peroxide generation in water. Rep Acad Sci (2008) 421(6):773–6. doi:10.1134/S0012500808080065
43. Rodrigo MA, Oturan N, Oturan MA. Electrochemically assisted remediation of pesticides in soils and water: a review. Chem Rev (2014) 114(17):8720–45. doi:10.1021/cr500077e
44. Ashurov MK, Belov SV, Gudkov SV, Danyleiko YK, Egorov AB, Savranskii VV, et al. Effects of low-temperature plasma glow discharge on the proliferative activity of cells and the repair functions of tissues in animals and plants. Biomed Eng (2020) 53:407–12. doi:10.1007/s10527-020-09953-x
45. Rusanov VD, Fridman AA, Sholin GV. The physics of a chemically active plasma with nonequilibrium vibrational excitation of molecules. Sov Phys Usp (1981) 24:447–74. doi:10.1070/PU1981v024n06ABEH004884
46. Kalinitchenko VP, Glinushkin AP, Minkina TM, Mandzhieva SS, Sushkova SN, Sukovatov VA, et al. Chemical soil-biological engineering theoretical foundations, technical means, and technology for safe intrasoil waste recycling and long-term higher soil productivity. ACS Omega (2020a) 5(28):17553–64. doi:10.1021/acsomega.0c02014
47. Kalinitchenko VP, Glinushkin AP, Sokolov MS, Sharshak VK, Ladan EP, Minkina TM, et al. Nature-based technologies of biogeosystem technique. Agrochem (2020b) 2:61–8. doi:10.31857/S0002188120020052
49. Smirnoff N, Arnaud D. Hydrogen peroxide metabolism and functions in plants. New Phytol (2018) 221(3):1197–214. doi:10.1111/nph.15488
50. Ashurov M, Belov S, Danyleiko Y, Otamirzaev N, Korshunov A, Demin D, et al. Method of preparation and biological activity of aqueous hydrogen peroxide solution. IOP Conf Ser Earth Environ Sci (2019) 390. doi:10.1088/1755-1315/390/1/012028
51. Kalinichenko VP. Inventor; Institute of Fertility of Soils of South Russia, assignee. Method of intra-soil pulse discrete irrigation. Russian Federation patent RU 2386243 (2010).
52. Kalinitchenko VP. Optimizing the matter flow in biosphere and the climate of the Earth at the stage of technogenesis by methods of biogeosystem technique (problem-analytical review). Int J Environ Probl (2016) 4(2):99–130. doi:10.13187/ijep.2016.4.99
53. Belov SV, Danileiko YK, Nefedov SM, Osiko VV, Salyuk VA, Sidorov VA. Specific features of generation of low-temperature plasma in high-frequency plasma electrosurgical apparatuses. Biomed Eng (2011) 5(2):59–63. doi:10.1007/s10527-011-9210-4
54. Wojtyla Ł, Lechowska K, Kubala S, Garnczarska M. Different modes of hydrogen peroxide action during seed germination. Front Plant Sci (2016) 7, 66. doi:10.3389/fpls.2016.00066
55. Glinushkin AP, Kudeyarov VN, Sokolov MS, Zinchenko VE, Chernenko VV. Nature-similar technologies of the biogeosystem technique in solving a global social and environmental problem. Biogeosyst Tech (2018) 5(2):159–96. doi:10.13187/bgt.2018.2.159
56. Niemoeller B, Harms HH, Lang T. Injection of liquids into the soil with a high-pressure jet. Agric Eng Int (2011) 13:2.
57. Silva MJ, Franco HCJ, Magalhães PSG. Liquid fertilizer application to ratoon cane using a soil punching method. Soil Tillage Res (2017) 165:279–85. doi:10.1016/j.still.2016.08.020
58. Lin H. Understanding soil architecture and its functional manifestation across scales. Part I: overviews and fundamentals. In: Hydropedology. Netherlands, EU: Elsevier (2012). p. 41–74.
59. Totsche KU, Amelung W, Gerzabek MH, Guggenberger G, Klumpp E, Knief C, et al. Kögel-Knabner I. Microaggregates in soils. Review Article. Special Issue: methodological advances in studying the soil–plant–atmosphere gas exchange. J Plant Nutr Soil Sci (2018) 181(1):104–36. 10.1002/jpln.201600451
60. Roth V, Lange M, Simon C, Hertkorn N, Bucher S, Goodall T, et al. Persistence of dissolved organic matter explained by molecular changes during its passage through soil. Nat Geosci (2019) 12:755–61. doi:10.1038/s41561-019-0417-4
61. Piccolo A, Spaccini R, Savy D, Drosos M, Cozzolino V. The soil humeome: chemical structure, functions and technological perspectives. In: S VazJr, editor. Sustainable agrochemistry. Cham, SW: Springer (2019). p. 183–222.
62. Kharitonova GV, Shein EV, Krutikova VO, Ostrouhov AV. Calcium carbonate formations in edaphic components of ecosystems. Biogeosystem Tech (2018) 5(2):197–212. doi:10.13187/bgt.2018.2.197
63. Shein EV, Kharitonova GV, Bayasgalan A, Gantumur S, Krutikova VO, Kharitonov EV. Salt neoformations in soils of Central Mongolia. Biogeosyst Tech (2017) 4(1):66–81. doi:10.13187/bgt.2017.1.66
64. Kalinichenko V. Biogeosystem technique as a problem. Biogeosystem Tech (2014) 1(1):4–19. doi:10.13187/bgt.2014.1.4
65. Grassian VH. Physical chemistry of environmental interfaces: aerosols, nanomaterials and indoor surfaces. Chemist (2019) 91:13–7.
Keywords: plasma, glow discharge, hydrogen peroxide, plant growth stimulation, biogeosystem technique
Citation: Belov SV, Danyleiko YK, Glinushkin AP, Kalinitchenko VP, Egorov AV, Sidorov VA, Konchekov EM, Gudkov SV, Dorokhov AS, Lobachevsky YP and Izmailov AY (2021) An Activated Potassium Phosphate Fertilizer Solution for Stimulating the Growth of Agricultural Plants. Front. Phys. 8:618320. doi: 10.3389/fphy.2020.618320
Received: 26 October 2020; Accepted: 27 November 2020;
Published: 14 January 2021.
Edited by:
Laura Stricker, ETH Zürich, SwitzerlandReviewed by:
Galina Kharitonova, Far Eastern Branch of the Russian Academy of Sciences, RussiaVitalii Pichura, Kherson State Agrarian University, Ukraine
Copyright © 2021 Belov, Danyleiko, Glinushkin, Kalinitchenko, Egorov, Sidorov, Konchekov, Gudkov, Dorokhov, Lobachevsky and Izmailov. This is an open-access article distributed under the terms of the Creative Commons Attribution License (CC BY). The use, distribution or reproduction in other forums is permitted, provided the original author(s) and the copyright owner(s) are credited and that the original publication in this journal is cited, in accordance with accepted academic practice. No use, distribution or reproduction is permitted which does not comply with these terms.
*Correspondence: Evgeny M. Konchekov, eukmek@gmail.com