- 1Dipartimento di Scienze di Base e Applicate per l’Ingegneria, “Sapienza” Università di Roma, Rome, Italy
- 2Istituto Nazionale di Fisica Nucleare, Laboratori Nazionali di Frascati, Frascati, Italy
- 3Istituto Nazionale di Fisica Nucleare, Sezione di Milano, Milan, Italy
- 4Dipartimento di Fisica, “Sapienza” Università di Roma, Rome, Italy
- 5Museo Storico della Fisica e Centro Studi e Ricerche Enrico Fermi, Rome, Italy
- 6Istituto Nazionale di Fisica Nucleare, Sezione di Roma, Rome, Italy
- 7Scuola di Specializzazione in Fisica Medica, “Sapienza” Università di Roma, Rome, Italy
- 8Dipartimento di Fisica, Università degli Studi di Milano, Milan, Italy
- 9Integrated Radiation and Image Sensors (IRIS), Fondazione Bruno Kessler, Trento, Italy
Secondary neutrons produced in particle therapy (PT) treatments are responsible for the delivery of a large fraction of the out-of-target dose as they feebly interact with the patient body. To properly account for their contribution to the total dose delivered to the patient, a high precision experimental characterisation of their production energy and angular distributions is eagerly needed. The experimental challenge posed by the detection and tracking of such neutrons will be addressed by the MONDO tracker: a compact scintillating fiber detector exploiting single and double elastic scattering interactions allowing for a complete neutron four-momentum reconstruction. To achieve a high detection efficiency while matching the fiber (squared,
Introduction
Particle therapy (PT) is a modern technique of solid tumor treatment that exploits the energy deposit of charged light ion beams, highly localised in the target region defined as “Bragg-Peak,” to preserve the healthy tissues. The interaction of therapeutic beams with the patient, besides releasing the dose necessary to induce the tumor cells apoptosis, produces secondary particles (neutral and charged radiation and beam fragments) whose contribution to the total dose is generally negligible. However, secondary neutrons can deposit an absolute nonnegligible energy in and out of field even far away from the target volume (voxel) as they are weakly interacting with the patient's body [1 2]. Their contribution has hence to be taken into account with the highest possible precision when performing the treatment plan optimisation and evaluating the dose absorbed by the organs at risk. To this aim, a high precision experimental characterisation of the secondary neutron spectra and fluxes is eagerly needed.
So far, the experimental attempts have been focused on measuring the total neutron production yields and energies, regardless of their production points summing up all the contributions coming from different production processes. To separate the secondary neutrons, produced directly from the beam interactions with the patient, from the ternary neutral component generated in the iterative interactions of fragmentation products with the treatment room and the patient itself, a tracking detector would hence be needed. Tracking the primary neutrons produced by the PT beam interactions with the patient tissues, which have energies of hundreds of MeV, is extremely challenging from the experimental point of view.
The MONDO tracker [3, 4] was designed, as a primary goal, to provide such capability for these ultrafast neutrons and to this aim exploits the detection single (SES) and double (DES) (consecutive) elastic scattering interactions. The detector is made out of a compact matrix of thin plastic scintillating fibers, assembled in orthogonally oriented layers, and has a total size of
The tracker layout has been optimised to ensure a detection efficiency of the order of
The readout sensor will be able to cover the full detector area, providing the necessary spatial resolution (matching the fiber granularity) and detection efficiency. An autotrigger algorithm will be implemented using a two-level strategy (at pixel and chip level), allowing a significant combinatorial background reduction, while keeping a very high efficiency for signal events [6].
The strategy to evaluate the tracker’s expected performance when detecting monoenergetic neutrons originating from a point-like source has already been discussed in detail elsewhere [7]. In this contribution, we present the evaluation of the expected performance using a detailed MC simulation of the neutrons production during a carbon ion PT treatment. The MC simulation was implemented using an accurate description of the detector geometry and accounted also for the trigger strategy.
The pixel size has been chosen in order to oversample the fiber; however, a fiber-pixel coupling is not expected to be possible, as the size of the fibers can range with a tolerance of about
At present time, the Mylar foils and the glue are not included in the MC simulation.
The simulation was used as the basis for the background rejection algorithm development and to evaluate the performance of the fragment energy measurement strategies (for both partially and fully contained tracks). In this work, the elastic scattering event selection was performed exploiting MC truth information: protons emitted from single and double elastic scattering are selected using an elastic flag that identified the events. The secondary neutron spectra expected from a carbon ion beam impinging on a PMMA target have been used to evaluate the detector neutron tracking performance in a PT case scenario.
In the following, we present the simulation details, and we report the expected detector performance, in terms of both back-pointing resolution and efficiency.
Materials and Methods
To evaluate the expected performance of the MONDO neutron tracker in a clinical environment, an MC simulation has been used. In the following, we describe the procedure used to evaluate the detector performance and the details about the simulation configuration.
The MC simulation studies discussed in this manuscript, used to evaluate the expected performance of the MONDO tracker in the context of PT applications, are based on the FLUKA code (FLUKA 2011.2c) [8, 9]. In particular, the study presented hereafter aimed at the evaluation of the detector backtracking efficiency and energy resolution.
The back-pointing performance is crucial in order to discriminate the scattered neutrons coming from the target and the ones produced by subsequent interactions with the treatment room environment. The latter contribution is an unavoidable background when using traditional, nontracking, neutron detectors. In this case, only the overall neutron production can be measured. Instead, by using a tracking detector like MONDO, it is possible to measure the neutron flux disentangling the different components. The precision achievable on the neutron production point plays a crucial role in this task. For this reason, an MC simulation has been set up to evaluate the MONDO potential in detecting the emission point of the neutrons produced by PT hadron beams (e.g., p or 12C). The neutron production induced by a hadron beam is represented schematically in Figure 1. MONDO is a compact, movable detector and can be placed in different positions and at different angles with respect to the neutron production point. In this way, it is possible to reconstruct neutron production fluxes as a function of the emission angles. Moreover, the irreducible background of ternary neutrons produced from secondary interactions with patients and with the treatment room can be identified and rejected, thanks to the tracking capability of the detector. The distance between detector and neutrons production source (e.g., the fraction of solid angle) will be adapted to the specific treatment room and chosen beam rate. Assuming that the tracker is used to study the neutron production induced by PT beams, different strategies in the data analysis can be pursued depending on the experimental conditions. It is possible to exploit the reconstruction of single elastic scattering events (SES), assuming that the production point is known when studying the beam interactions with a thin target. Whenever the production point is not known (as in the case of PT applications), it is however possible to perform the full reconstruction of double elastic scattering events (DES) and measure the neutron four-momentum disentangling the primary and secondary radiation components. In Figure 1, a schematic representation of a DES event reconstruction is shown, showing the principle behind the neutron emission point measurement.
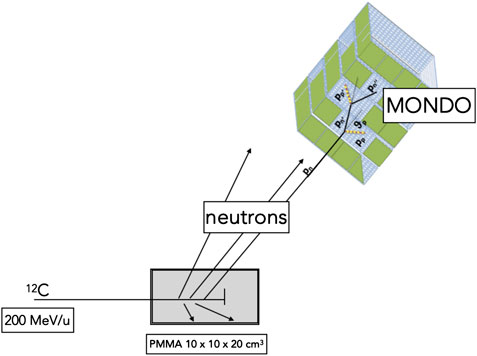
FIGURE 1. Schematic representation of the detection of a DES event in MONDO for a neutron produced in a target by a particle therapy beam. The beam (p or 12C) interacts with the target (e.g., patient tissues or material placed on the beamline) producing neutrons detectable in MONDO. The neutron emission point can be measured once the neutron four-momentum reconstruction is performed and the relative position of the detector and the target is known.
A detailed description of the detection strategy of how SES and DES can be used to measure the neutron characteristic is discussed elsewhere [4, 10]. In the following, the two-step approach adopted in the simulation to specifically reproduce the conditions expected in the PT environment is discussed.
Firstly, the expected neutron spectra, produced in PT treatments, have been obtained by means of a dedicated MC simulation. The energy of the neutrons produced by the interactions of 12C ions of 200 MeV/u energy with a PMMA phantom (volume: 10 × 10 × 20 cm3) has been obtained. The primary ions were shot at the center of the entrance phantom face (x and y planes), along the z-axis.
The second step has been the study of the interaction of such neutrons with the detector in a simplified setup: neutrons with the proper energy spectra (obtained in the previous step) have been generated, assuming a point-like source placed
The MONDO tracker layout used for the simulation has been optimised for the reconstruction of neutrons produced in PT applications [4]. The simulated detector has 800 layers of
The response of the MONDO detector has been carefully included in the simulation to evaluate the resolution on the neutron energy and direction reconstruction, for both SES and DES events. The interactions of all the particles with the fiber matrix have been recorded by FLUKA and the details in the conversion of the collected light, at the fiber output, and the number of pixels that had an over threshold signal have been obtained.
The SPAD-based readout, described in Ref. 5, has been implemented in the simulation allowing us to test the reconstruction algorithms starting from the information provided in terms of pixels that have a signal over the threshold when a particle interacts with the corresponding fiber. The readout response has been simulated in an external dedicated code that uses as input the energy release in the fibers and processes this information following the measured performances [6]. When a scintillating fiber is crossed by a particle, it releases a
must be applied to all the considered photons impinging on the considered pixel.
The noise of the detector (dark current) has been included in the simulation of the detector but in this study, this contribution has not been taken into account and only the physical interactions of the particles with the detector have been considered. A more detailed experimental characterisation of the sensor is needed in order to implement reasonable values and obtain a realistic tracking evaluation.
The detector performance has been studied using different energy spectra as input: either monochromatic or following the expected energy dependence in case of a PT application scenario. The obtained results are shown in the following section.
Results
Monochromatic Beams
The kinetic energy of the recoil protons produced via elastic scattering interaction is calculated via range measurements in the MONDO baseline approach. Therefore, full containment of the proton tracks in the detector is required. To study the impact on the efficiency achievable in the neutron tracking, monoenergetic neutron beams in the range between 30 and 300 MeV have been studied. Neutrons undergoing both SES and DES were reconstructed applying the full containment constraint to the scattered protons and the obtained results were presented in Ref. 11. The expected DES (SES) interactions range from
For this reason, a different and complementary strategy has been developed in order to recover some of the events that were not totally contained inside the detector. In order to compute the proton kinetic energy, the deposited energy in each fiber and the timing of the scintillation photons have been studied. The proton
For all the events with proton crossing at least 10 fibers, the length of the particle tracks inside the detector has been reconstructed for both contained and noncontained protons and the value of the energy loss in the single fibers provided from the FLUKA simulation has been collected for the first
The results are shown in Figure 2 both for the neutron four-momentum reconstruction performed using the range (fully contained events, shown in orange) and for the energy loss inside the fibers for partially contained events (shown in green). While for neutron incoming energies below
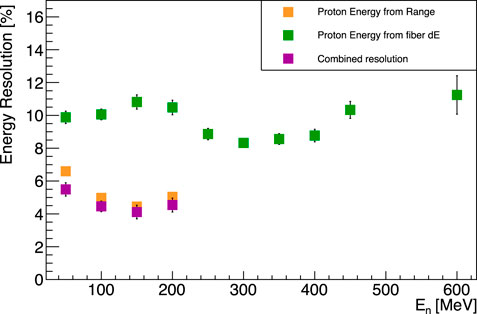
FIGURE 2. Energy resolution for DES events as a function of the incoming neutron kinetic energy. Orange squares represent the energy resolution obtained via energy-range measurements while the green squares show resolution obtained from the
While the energy resolution achievable with the range method is more performing (
The monoenergetic beams have been used to study the back-pointing resolution as a function of the incoming neutron energy. The event reconstruction proceeds as detailed in Ref. 10: DES events are flagged and selected by means of MC truth information, the events are processed, and the production point is obtained, backtracking the reconstructed neutron in the plane that contains the point-like source. The distribution in the x and y planes for neutrons with incoming energy of
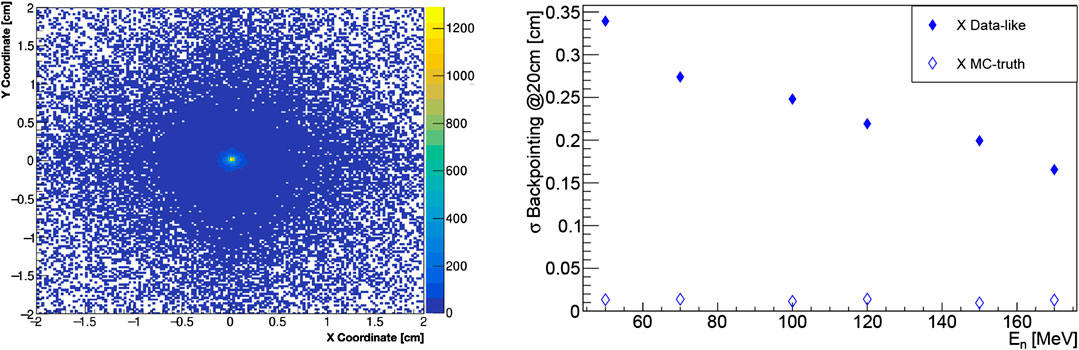
FIGURE 3. (Left) Two-dimensional reconstructed emission point at
The projections along the x and y directions can be obtained and a Gaussian fit can be performed in order to extract the single neutron reconstruction emission point resolution. The results for the projections in the x direction are shown in Figure 3 (right, full markers) as a function of the neutron energies. In order to evaluate the intrinsic resolution on the neutron production point related to the uncertainty on the production angles and energy loss in the fibers, the back-pointing has been computed exploiting the MC truth kinetic energy of the protons; thus, the empty markers show the resolution obtained in backtracking the neutron on its production plane. As can be seen, such contribution is nearly negligible and confirms the observation that the dominant contribution to the neutron tracking resolution in DES events is related to the error in reconstructing the protons angle and energy. The achievable resolution is below
PT-Like Beams
The energy spectra of the neutrons produced in the interactions of carbon ions of
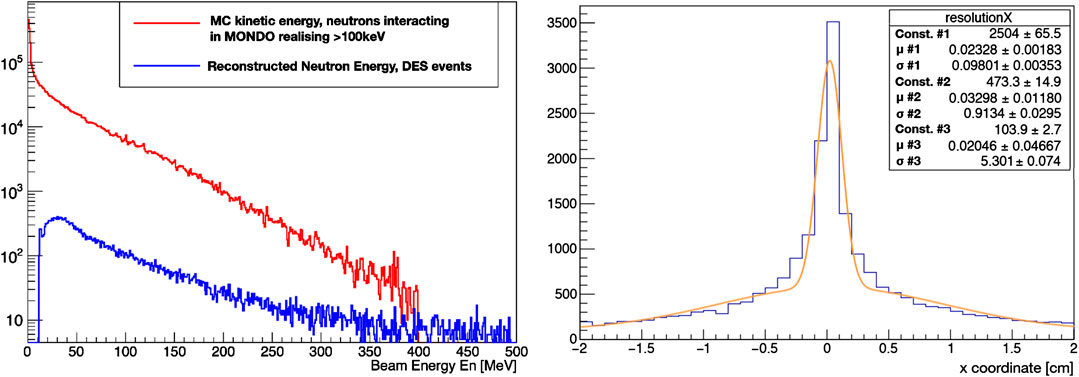
FIGURE 4. (Left) Comparison between the simulated neutron spectrum generated from a
For all the reconstructed events, a production point distribution in the plane containing the point-like source has been obtained. In Figure 4 (right), the profile along the x-axis of the reconstructed point is shown. A similar symmetric behavior in the x and y projections is observed as in the case of monoenergetic beams. A fit with a function implementing three Gaussian components is performed in order to account for the different contributions to the tracking resolution and the impact of a tail at large values: an overall resolution of
Discussion
The potential of the MONDO tracker in characterising the neutrons produced in a PT treatment with carbon ions has been explored using neutrons from a point-like source placed
While the geometry and readout structure have been implemented in the MC simulation, the detector electronic noise and the fiber crosstalk have not yet been introduced in the analysis of the MC-data events, as an experimental and complete characterisation of the readout sensor coupled with the scintillating fibers is still ongoing.
A preliminary event reconstruction software for which the full simulation chain is accounted, starting from the particles’ interactions with the MONDO fibers up to the signal collection (readout response in terms of pixels with signals over threshold), has been implemented and DES events have been reconstructed and used to evaluate the detector performance.
Results have been obtained for monoenergetic beams and for neutrons generated with the energy spectrum expected in PT applications. In that condition, an energy resolution of
The preliminary results presented hereafter fully support the capability of MONDO to monitor the neutrons produced in PT treatments with the required precision needed to disentangle the secondary and ternary neutron components, thus the direct production of beam interactions with the patient tissues from the neutrons produced by interactions with the surrounding environment.
Data Availability Statement
The raw data supporting the conclusions of this article will be made available by the authors, without undue reservation.
Author Contributions
All the authors contributed to the MONDO detector conception and development. MT contributed to the prototype test and readout characterisation. GB developed the MC code of the detector and participated in the prototype test beams. AB and GT contributed to the prototype readout characterisation and to the mechanical assembly of prototypes. MD, PD and YD contributed to the prototype test beams and to the MC development. MF and GF contributed to the readout characterisation and MC development. RM contributed to the prototype test characterisation, to the readout conception and test, to the MC development, and to the mechanical definition of the assembly strategy. MaM contributed to the mechanical definition and assembly procedure definition of the detector and its prototype. IM, SM, and AnS developed the MC framework for the carbon on target simulation. AdS, AlS, and VP developed the MC code of the detector, contributed to the readout conception, and participated in the test and characterisation of the detector prototypes and in the mechanical definition of the assembly strategy. LG, EM, and LP developed the innovative readout sensor SBAM. MiM supervised, coordinated, and contributed to the full system development and implementation, to the detector characterisation, and to the MC framework development.
Conflict of Interest
The authors declare that the research was conducted in the absence of any commercial or financial relationships that could be construed as a potential conflict of interest.
References
1. Gunzert-Marx K, Schardt D, Simon RS. Fast neutrons produced by nuclear fragmentation in treatment irradiations with 12C beam. Radiat Protect Dosim (2004). 110:595–600. doi:10.1093/rpd/nch138.
2. Hultqvist M, Gudowska I. Secondary absorbed doses from light ion irradiation in anthropomorphic phantoms representing an adult male and a 10 year old child. Phys Med Biol (2010). 55:6633–53. doi:10.1088/0031-9155/55/22/004
3. Schardt M, Patera V, Pinci D, Sarti A, Sciubba A, Spiriti E. Mondo: A neutron tracker for particle therapy secondary emission fluxes measurements. Nucl Instrum Methods Phys Res Sect A Accel Spectrom Detect Assoc Equip (2016). 824:210–211. doi:10.1016/j.nima.2015.10.109.
4. Marafini M, Gasparini L, Mirabelli R, Pinci D, Patera V, Sciubba A, et al. MONDO: a neutron tracker for particle therapy secondary emission characterisation. Phys Med Biol (2017). 62:3299–312. doi:10.1088/1361-6560/aa623a.
5. Mirabelli R, Gasparini L, Magi M, Marafini M, Pinci D, Sarti A, et al. The mondo detector prototype development and test: steps toward an spad-cmos-based integrated readout (SBAM sensor). IEEE Trans Nucl Sci (2018). 65:744–51. doi:10.1109/TNS.2017.2785768.
6. Manuzzato E, Gioscio E, Mattei I, Mirabelli R, Patera V, Sarti A, et al. A 16 × 8 Digital-SiPM array with distributed trigger generator for low SNR particle tracking. IEEE Solid-State Circuits Lett (2019). 2:75–8. doi:10.1109/LSSC.2019.2934598.
7. Valle S, Battistoni G, Patera V, Pinci D, Sarti A, Sciubba A, et al. The mondo project: A secondary neutron tracker detector for particle therapy. Nucl Instrum Methods Phys Res Sect A: Accel Spectrom Detect Ass Equip (2017). 845:556–9. doi:10.1016/j.nima.2016.05.001.
8. Ferrari A, Sala PR, Fasso A, Ranft J. FLUKA: a multi-particle transport code (Program version 2005) (2005). p. 405. CERN-2005-010, SLAC-R-773, INFN-TC-05-11 (2005).
9. Battistoni G, Bauer J, Boehlen T. T, Cerutti F, Chin M. P. W, Dos Santos Augusto R, et al. The fluka code: An accurate simulation tool for particle therapy. Front Oncol (2016). 6:116. doi:10.3389/fonc.2016.00116.
10. Giacometti V, Battistoni G, De Simoni M, Dong Y, Fischetti M, Gioscio E, et al. Characterisation of the mondo detector response to neutrons by means of a fluka Monte Carlo simulation. Radiat Meas (2018). 119:144–149. doi:10.1016/j.radmeas.2018.10.006.
11. Gioscio E, Battistoni G, Bochetti A, De Simoni M, Dong Y, Fischetti M, et al. Development of a novel neutron tracker for the characterisation of secondary neutrons emitted in particle therapy. Nucl Instrum Methods Phys Res Sect A Accel Spectrom Detect Assoc Equip (2020). 958:162862. doi:10.1016/j.nima.2019.162862.
Keywords: neutron tracking, particle therapy, carbon ions radiotherapy, secondary radiation monitoring, SPAD technology
Citation: Toppi M, Battistoni G, Bochetti A, De Maria P, De Simoni M, Dong Y, Fischetti M, Franciosini G, Gasparini L, Magi M, Manuzzato E, Mattei I, Mirabelli R, Muraro S, Parmesan L, Patera V, Perenzoni M, Sarti A, Schiavi A, Sciubba A, Traini G, Valle SM and Marafini M (2020) The MONDO Tracker: Characterisation and Study of Secondary Ultrafast Neutrons Production in Carbon Ion Radiotherapy. Front. Phys. 8:567990. doi: 10.3389/fphy.2020.567990
Received: 23 June 2020; Accepted: 16 October 2020;
Published: 19 November 2020.
Edited by:
Laurent Ottaviani, Aix-Marseille Université, FranceReviewed by:
Peter R. Hobson, Queen Mary University of London, United KingdomDavid Christian, Fermilab (DOE), United States
Copyright © 2020 Toppi, Battistoni, Bochetti, De Maria, De Simoni, Dong, Fischetti, Franciosini, Gasparini, Magi, Manuzzato, Mattei, Mirabelli, Muraro, Parmesan, Patera, Perenzoni, Sarti, Schiavi, Sciubba, Traini Valle and Marafini. This is an open-access article distributed under the terms of the Creative Commons Attribution License (CC BY). The use, distribution or reproduction in other forums is permitted, provided the original author(s) and the copyright owner(s) are credited and that the original publication in this journal is cited, in accordance with accepted academic practice. No use, distribution or reproduction is permitted which does not comply with these terms.
*Correspondence: Riccardo Mirabelli, cmljY2FyZG8ubWlyYWJlbGxpQHJvbWExLmluZm4uaXQ=