- Centre for THz Research, China Jiliang University, Hangzhou, China
We have fabricated a Jesus-cross structure on aluminum foil using the femtosecond laser technique. Using the terahertz time-domain spectroscopy (THz-TDS) system, the transmission properties of free-standing double-layer crossing fractal structure are tested. The parameters of the proposed structure were optimized using the finite element frequency domain technology of commercial software CST Microwave Studio package. The dimensions of the aluminum foil periodically patterned with crossing fractal structure are 1.5 × 1.5 cm2. The resonant frequencies of the proposed structure are 0.216 and 0.735 THz with 3-dB bandwidths of 62 and 15 GHz, respectively. The transmission ratio can reach to 0.89 and 0.57. It indicates the structure having dual-band filtering performance. This work has the potential to open a new avenue as a filter working for free-space terahertz radiation.
Introduction
Nowadays, terahertz (THz) wave has attracted widespread concern due to its unique applications such as wireless communication, imaging, security, etc. As an essential part of a terahertz wave system, terahertz wave manipulation is highly required. Different functional THz devices have been reported, such as modulator [1], filters [2, 3], switches [4–6], phase shifters [7], polarizers [8], absorbers [9, 10], and splitters [11–13]. We all know that terahertz wave filter is a kind of important signal processing device [14–16]. However, most of the reported terahertz filters are fabricated using photolithography processes, which results in high cost and is time consuming. Recently, various concepts of terahertz wave filters utilizing liquid crystal, frequency selective surface (FSS), graphene, photonic crystal, or metamaterial have been described [17–24]. To the best of our knowledge, relatively few studies on simple and efficient method to fabricate terahertz filter are reported. Therefore, terahertz filters are required for further research, and it is very valuable to find a simple method for fabricating terahertz filter.
In this article, we present a free-standing double-layer crossing fractal structure, which consists of symmetrical periodic metallic crossing fractal patterned on aluminum foil. We demonstrate a technique using femtosecond laser for aluminum foil fabrication to make a compact and free-standing terahertz band-pass filter. Theoretical simulation was carried out using the full-wave finite element frequency domain method of the commercial software CST Microwave Studio package. The measured terahertz transmission response spectrum shows a reasonable correspondence with simulation. The designed structure has simplicity, small size, high transmittance, and low loss. In addition, the free-standing double-layer crossing fractal-based terahertz filters are suitable for application in terahertz systems due to their small size and fabrication using femtosecond laser high-precision micromachining technology. This work has the potential to open a new avenue as a dual-band filter working for free-space terahertz wave radiation.
Device Structure Design and Parameters Study
The structure of the present free-standing crossing fractal is depicted in Figure 1. The array of crossing fractal structures is fabricated on aluminum foil with a conductivity of 3.56 × 107 S/m. The geometrical parameters of the crossing fractal unit cell are of a, g, h, and t. The structure is calculated using CST Microwave Studio. The unit cell is applied with periodic boundary conditions. Terahertz propagation vector is perpendicularly incident to the crossing fractal structure. The optimized dimensions of the crossing fractal structure are as follows: a = 500 μm, aluminum foil thickness of 10 μm, t = 45 μm, h = 480 μm, s = 135 μm, and g = 30 μm. Here, we have simulated the frequency behavior of the power transmission based on the varied parameters such as lattice period a and distance between two layer aluminum foils t. Figure 2 shows the frequency behavior of the crossing fractal-air-crossing fractal power transmission for different lattice periods of the crossing fractal geometrical parameter a as the other parameters using their optimized values. From Figure 2, one sees that the lattice period a mainly controls the first resonance frequency of the proposed structures. Particularly, it can be found that the first resonance frequency moves downward as the lattice period a increases. For the crossing fractal-air-crossing fractal structure, one can see that the first resonant central frequency is 0.24 THz with transmittance of 0.96. At this time, the 3-dB bandwidth is 0.189–0.287 THz, covering the terahertz communication region, when a equals 500 μm. In addition, the second resonant central frequency is 0.64 THz. The 3-dB bandwidth is from 0.584 to 0.704 THz.
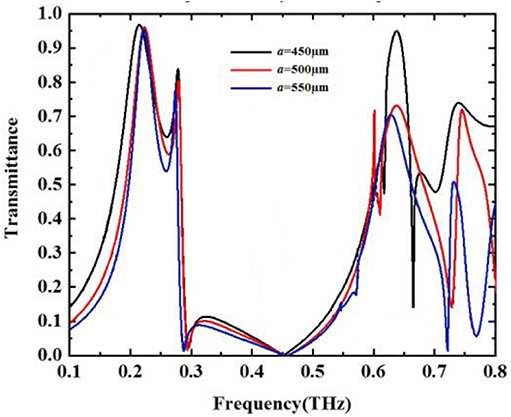
Figure 2. Transmittance spectra of the proposed crossing fractal-air-crossing fractal structure for various values of a.
When the distance between two layer aluminum foils (t) changes from 35 to 55 μm, the other sizes of the proposed crossing fractal structure still adopt the optimized values. Figure 3 depicts the terahertz power transmission with different frequencies. It can be noted that the first and the second resonance central frequencies move downward as the distance between two layer aluminum foils of the parameter t is increased on the crossing fractal-air-crossing fractal structure. When t is equal to 45 μm, the two resonant peaks are of 0.24 and 0.64 THz, respectively. The 3-dB bandwidth of the first and second resonance peaks range from 0.189 to 0.287 THz and from 0.584 to 0.704 THz, respectively. To cover the terahertz communication frequency band, we set the gap between two layer aluminum foils (t) as 45 μm. To clarify the transmission mechanism of the proposed structure, we simulated the electric field and surface current of double-layer crossing fractal structure. From Figures 4A,B, we can find that the resonance of 0.24 THz is generated by the electric dipole response of two perpendicular crossing arms. The surface current mainly flows on the perpendicular metal arms of the crossing fractal pattern (see Figure 4B). For the resonance frequency of 0.64 THz, as shown in Figures 4C,D, the resonance is stimulated by the electric dipole response of the horizontal arm. The surface free charge accumulated at the left and right areas of the horizontal arm forms the external electric field (see Figure 4D). It is pretty obvious that the first and the second transmission peaks are associated with the resonances of the crossing fractal structure.
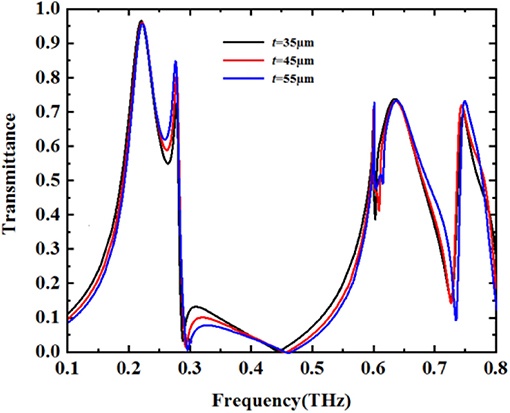
Figure 3. Transmittance spectra of the proposed crossing fractal-air-crossing fractal structure for various values of t.
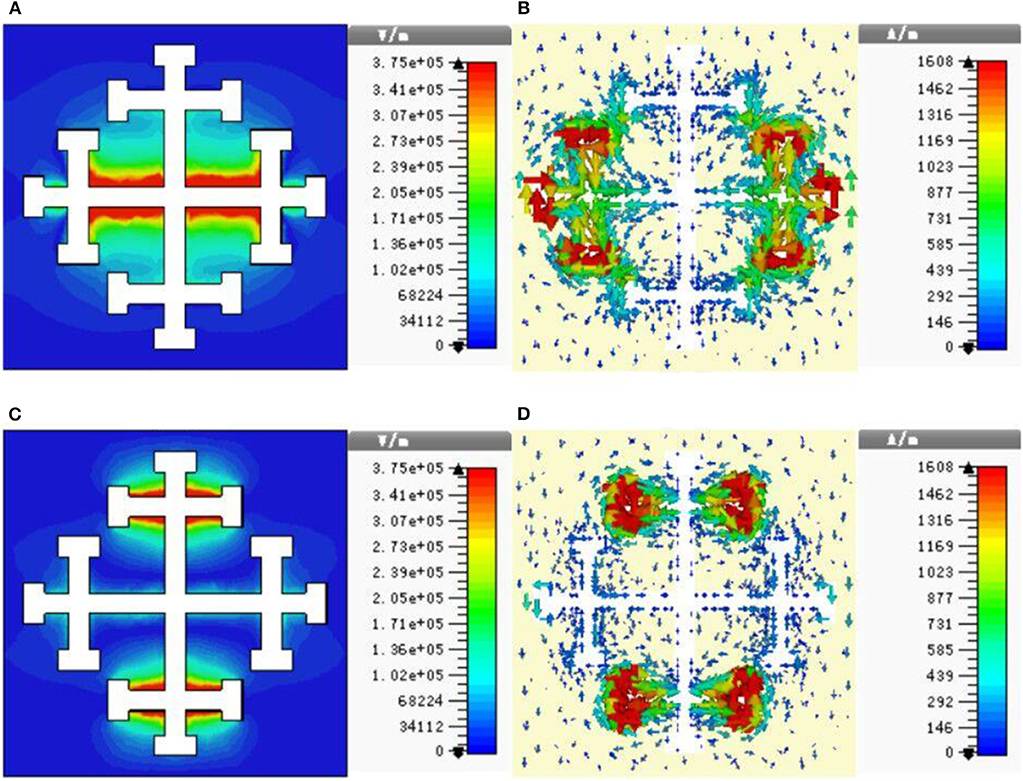
Figure 4. Electric field distribution of the unit cell at the resonance frequency of (A) 0.24 THz and (C) 0.64 THz and the simulated surface current distribution on the crossing fractal layer at the resonance frequency of (B) 0.24 THz and (D) 0.64 THz.
Fabrication and Measurement
According to the optimized physical dimensions, the proposed free-standing crossing fractal structure is fabricated on a 10-μm aluminum foil with a conductivity of 3.56 × 107 S/m using an ultrafast high-intensity laser technique. The femtosecond laser has 45 fs pulse width, 800 nm wavelength, and 1 kHz repetition rate. In addition, the laser with 50 μJ pulse energy, 10 μm spot size, and 1 mm/s moving speed is employed to fabricate crossing fractal structure. The aluminum foil is placed on a precise computer-controlled platform. An objective lens is used to focus the femtosecond laser on the aluminum foil surface. Figure 5 shows the photography of the fabricated structure and a local enlarged microscopic image. A Z2 THz-TDS system from Zomega Co. Ltd. is employed to test the terahertz power transmission of the present crossing fractal-air-crossing fractal structure at room temperature of 25°C. To achieve a high signal/noise ratio in the frequency range from 0.2 to 0.8 THz, each spectrum was obtained by averaging three scans. Thus, the results provided here are repeatable and credible. Figure 6 shows the measured and simulated transmittance spectra of the proposed crossing fractal structure. The transmittance of the single-layer structure can be given by S = |S21|2, where S21 is the transmission coefficient. Similarly, the power transmittance of the two-layer structure is expressed as T = |S|2. The measured results show that the crossing fractal-air-crossing fractal structure has a 3-dB bandwidth of 62 GHz from 0.216 to 0.278 THz with center frequency located at 0.245 THz, and 15 GHz from 0.66 to 0.81 THz with center frequency located at 0.735 THz. The first and the second transmission peaks of the crossing fractal-air-crossing fractal structure are 0.89 and 0.57, respectively. The experimental result has some striking discrepancy with the simulation due to the limitation of its mechanical tolerance, which has been neglected in our simulation.
Conclusion
In summary, we proposed a dual-band terahertz band-pass filter based on the free-standing crossing fractal structure working for free-space terahertz radiation. We analyzed the filtering spectrum performance with various geometrical parameters of the double-layer crossing fractal structure. Using the femtosecond laser technique, we have fabricated double-layer Jesus-cross structure on aluminum foil. The frequency response of the filter is tested using THz-TDS. The resonant peaks of the filter are at 0.245 THz with the transmittance of 0.89 and 0.735 THz with the transmittance of 0.57, respectively. The experimental result has some discrepancies with those of our simulation. Owing to the symmetrical characteristic of the free-standing crossing fractal structure, the proposed filter is polarization insensitive. Our design will have great potential applications in terahertz communications, imaging, and terahertz sensor systems due to its simple structure and ease of manufacturing.
Data Availability Statement
All datasets generated for this study are included in the article/supplementary material.
Author Contributions
R-HX carried out the whole experiment. JL wrote the paper. All authors discussed the results and contributed to the paper.
Funding
This work was supported by the National Natural Science Foundation of China (Grant Nos. 61871355 and 61831012).
Conflict of Interest
The authors declare that the research was conducted in the absence of any commercial or financial relationships that could be construed as a potential conflict of interest.
References
1. Han Z, Kohno K, Fujita H, Hirakawa K, Toshiyoshi H. MEMS reconfigurable metamaterial for terahertz switchable filter and modulator. Opt Express. (2014) 22:21326–39. doi: 10.1364/OE.22.021326
2. Rao L, Yang D, Zhang L, Li T, Xia S. Design and experimental verification of terahertz wideband filter based on double-layered metal hole arrays. Appl Opt. (2012) 51:912–8. doi: 10.1364/AO.51.000912
3. Miyamaru F, Hangyo M. Anomalous terahertz transmission through double-layer metal hole arrays by coupling of surface plasmon polaritons. Phys Rev B. (2005) 71:165408. doi: 10.1103/PhysRevB.71.165408
4. Javan A, Granpayeh N. Terahertz wave switch based on photonic crystal ring resonators. Opt. Quant Electron. (2008) 40:695–705. doi: 10.1007/s11082-008-9257-y
5. Li J, He J, Hong Z. Terahertz wave switch based on silicon photonic crystals. Appl Opt. (2007) 46:5034–7. doi: 10.1364/AO.46.005034
6. Wilk R, Vieweg N, Kopschinski O, Koch M. Liquid crystal based electrically switchable Bragg structure for THz waves. Opt Express. (2009) 17:7377–82. doi: 10.1364/OE.17.007377
7. Wu Y, Ruan X, Chen C, Shin Y, Lee Y, Niu J, et al. Graphene/liquid crystal based terahertz phase shifters. Opt Express. (2013) 21:21395–402. doi: 10.1364/OE.21.021395
8. Huang Z, Parrott E, Park H, Chan H, MacPherson E. High extinction ratio and low transmission loss thin-film terahertz polarizer with a tunable bilayer metal wire-gridstructure. Opt Lett. (2014) 39:793–6. doi: 10.1364/OL.39.000793
9. Fu SM, Zhong YK, Ju NP, Tu MH, Chen BR, Lin A. Broadband polarization-insensitive metamaterial perfect absorbers using topology optimization. IEEE Photon J. (2016) 8:1–11. doi: 10.1109/JPHOT.2016.2602335
10. Tang JY, Xiao ZY, Xu KK, Ma XL, Wang ZH. Polarization-controlled metamaterial absorber with extremely bandwidth and wide incidence angle. Plasmonics. (2016) 11:1393–9. doi: 10.1007/s11468-016-0189-2
11. Benjamin S, Weng B, Shepherd R, Abbott D, Fumeaux C. Inkjet printed conductive polymer-based beam-splitters for terahertz applications. Opt Mater Express. (2013) 3:1242–9. doi: 10.1364/OME.3.001242
12. Lai W, Born N, Schneider L, Rahimi-Iman A, Balzer J, Koch M. Broadband antireflection coating for optimized terahertz beam splitters. Opt Mater Express. (2015) 5:2812–9. doi: 10.1364/OME.5.002812
13. Li JS, Xu DG, Yao JQ. Compact terahertz wave polarizing beam splitter. Appl Opt. (2010) 49:4494–7. doi: 10.1364/AO.49.004494
14. Kaliteevski MA, Brand S, Cook JG, Abram RA, Chamberlain JM. Terahertz filter based on refractive properties of metallic photonic crystal. Opt Express. (2008) 16:7330–5. doi: 10.1364/OE.16.007330
15. Lo SZA, Murphy TE. Nanoporous silicon multilayers for terahertz filtering. Opt Lett. (2009) 34:2921–3. doi: 10.1364/OL.34.002921
16. Liang L, Jin B, Wu J, Huang Y, Ye Z, Huang X, et al. A flexible wideband bandpass terahertz filter using multi-layer metamaterials. Appl Phys B. (2013) 113:285–90. doi: 10.1007/s00340-013-5470-x
17. Lin Y, Qian Y, Ma F, Liu Z, Kropelnicki P, Lee C. Development of stress-induced curved actuators for a tunable THz filter based on double split-ring resonators. Appl Phys Lett. (2013) 102:111908. doi: 10.1063/1.4798244
18. Lu M, Li W, Brown ER. Second-order bandpass terahertz filter achieved by multilayer complementary metamaterial structures. Opt Lett. (2011) 36:1071–3. doi: 10.1364/OL.36.001071
19. Li S, Liu H, Sun Q, Huang N. A tunable terahertz photonic crystal narrow-band filter. IEEE Photon Technol Lett. (2015) 27:752–4. doi: 10.1109/LPT.2015.2391127
20. Vieweg N, Born N, Al-Naib I, Koch M. Electrically tunable terahertz notch filters. Infrared J Millimeter Terahertz Waves. (2012) 33:327–32. doi: 10.1007/s10762-012-9877-y
21. Yang K, Liu S, Arezoomandan S, Nahata A, Sensale-Rodriguez B. Graphene-based tunable metamaterial terahertz filters. Appl Phys Lett. (2014) 105:093105. doi: 10.1063/1.4894807
22. Correas-Serrano D, Gomez-Diaz JS, Carrier JP, Melcón 'AA. Graphene-based plasmonic tunable low-pass filters in the terahertz band. IEEE Trans Nanotechnol. (2014) 13:1145–53. doi: 10.1109/TNANO.2014.2344973
23. Lee ES, Lee SG, Kee CS, Jeon TI. Terahertz notch and low-pass filters based on band gaps properties by using metal slits in tapered parallel-plate waveguides. Opt Express. (2011) 19:14852–9. doi: 10.1364/OE.19.014852
Keywords: terahertz transmission, transmission ratio, fractal, CST, THz-TDS
Citation: Xiong R-H and Li J (2020) Terahertz Transmission Characteristics of Free-Standing Fractal Jesus-Cross Structure. Front. Phys. 8:23. doi: 10.3389/fphy.2020.00023
Received: 04 November 2019; Accepted: 27 January 2020;
Published: 27 February 2020.
Edited by:
Lin Chen, University of Shanghai for Science and Technology, ChinaReviewed by:
Dejun Liu, Shanghai Normal University, ChinaQiye Wen, University of Electronic Science and Technology of China, China
Min Hu, University of Electronic Science and Technology of China, China
Copyright © 2020 Xiong and Li. This is an open-access article distributed under the terms of the Creative Commons Attribution License (CC BY). The use, distribution or reproduction in other forums is permitted, provided the original author(s) and the copyright owner(s) are credited and that the original publication in this journal is cited, in accordance with accepted academic practice. No use, distribution or reproduction is permitted which does not comply with these terms.
*Correspondence: Jiu-sheng Li, lijsh2008@126.com