- 1Institute of Physics, Southern Federal University, Rostov-on-Don, Russia
- 2Laboratory of Astroparticle Physics and Cosmology, Paris, France
- 3Center for Cosmoparticle Physics “Cosmion” and National Research Nuclear University “MEPHI”, Moscow, Russia
Dark matter candidates are one of the profound signatures of new physics, reflecting existence of new stable particles. If such particles are charged they can bind with ordinary electrons, forming anomalous isotopes. Severe constraints on the anomalous hydrogen exclude new stable single charged particles, but the case of stable double charged particles is not excluded so easily. Similar to ordinary baryonic matter dark matter candidates can be in the form of neutral dark atoms in which new stable or sufficiently long living double charged particles are bound by ordinary Coulomb interaction. In the most simple case only negative double charged particles are bound in O-helium (OHe) dark atoms with primordial helium. OHe hypothesis can provide the solution for puzzles of direct dark matter searches by specifics of OHe interaction in the matter of underground detectors. OHe interaction in the matter can lead to formation of various exotic forms of O-nuclearities. Provided that the mass of the double charged particle is around 1.25 TeV OHe hypothesis can explain the observed positron annihilation line excess in the galactic bulge by pair production in de-excitation of OHe atoms colliding in this region. In the model of Walking Technicolor generation of primordial excess of negatively charged techniparticles (over their antiparticles) can be related to the baryon asymmetry of the Universe, giving proper relationship of baryonic and non-baryonic matter densities. Such primordial excess may be accompanied by a subdominant excess of longliving positively double charged techniparticles, whose decay to same sign pair of leptons can explain the high energy cosmic positron anomaly, detected by PAMELA and AMS02. This explanation should be confronted with the cosmic gamma ray background measured by FERMI/LAT, what put upper limit on the mass of a decaying double charged particle. It makes search for stable double charged particles at the LHC a direct probe for composite dark matter hypothesis.
1. Introduction
The nature of cosmological dark matter, dominating in the matter content of the modern Universe, is related to new physics. If it consists of particles these new particles are predicted beyond the Standard model (BSM). Such particles (see [1] for review and reference) should be stable, provide the measured dark matter density and be decoupled from plasma and radiation at least before the beginning of matter dominated stage. The easiest way to satisfy these conditions is to involve neutral elementary weakly interacting massive particles (WIMPs). However negative results of WIMP searches appeal to other possible BSM solutions for the dark matter problem.
In the list of such BSM candidates the existence of heavy stable charged particles bound in neutral dark atoms is of special interest. Such atoms can be bound by various new forces, but it turns out that even stable electrically charged particles can exist hidden in such atoms and bound by ordinary Coulomb interactions (see [1] and references therein).
Stable particles with a single charge ± 1 are excluded due to overproduction of anomalous isotopes. Even very small fraction of free +1 charged particles bound with electrons leads to overproduction of anomalous hydrogen isotopes that are severely constrained by the experimental data. Frozen out free -1 charged particles are captured by primordial helium nuclei, as soon as they are produced in the Big Bang Nucleosynthesis, and the formed +1 charged ions play the same dangerous role of “nuclei” of anomalous hydrogen isotopes.
However, there doesn't appear such an evident contradiction for negatively doubly charged particles.
There exist several types of BSM particle models where heavy stable –2 charged species, O−−, are predicted:
(a) AC-leptons, predicted as an extension of the Standard Model, based on the approach of almost-commutative (AC) geometry [2–4].
(b) Technileptons and anti-technibaryons in the framework of Walking Technicolor (WTC) [5–11].
(c) stable “heavy quark clusters” ŪŪŪ formed by anti-U quark of 4th generation [12]
(d) and, finally, stable charged clusters ū5ū5ū5 of (anti)quarks ū5 of 5th family can follow from the approach, unifying spins and charges[13].
It should be noted that the discovery and precise measurements of Higgs boson properties that appear to be very close to the predictions of the Standard model put severe constraints on the models (a)-(d). These constraints lead to more sophisticated WTC scenarios (see [14] for recent review) or imply strong suppression of heavy quarks to 125 GeV Higgs boson [15]. All these models also predict corresponding +2 charge particles. If these positively charged particles remain free in the early Universe, they can recombine with ordinary electrons in anomalous helium, which is not as much as hydrogen but still strongly constrained in terrestrial matter. Therefore, a cosmological scenario should provide a mechanism which suppresses anomalous helium. There are two possible mechanisms that can provide a suppression:
(i) The abundance of anomalous helium in the Galaxy may be significant, but in terrestrial matter a recombination mechanism could suppress this abundance below experimental upper limits [3]. The existence of a new U(1) gauge symmetry, causing new Coulomb-like long range interactions between charged dark matter particles, is crucial for this mechanism. This leads inevitably to the existence of dark radiation in the form of hidden photons.
(ii) Free positively charged particles are already suppressed in the early Universe and the abundance of anomalous helium in the Galaxy is negligible [12]. Such a charge asymmetric solution appeals to possible relationship between asymmetric dark matter and baryon asymmetry of the ordinary matter.
These two possibilities correspond to two different cosmological scenarios of dark atoms.
The first one is realized in the scenario with AC leptons, forming neutral AC atoms [3].
The second assumes a charge asymmetry of the O−− which forms the atom-like states with primordial helium [12]. If new stable species belong to non-trivial representations of the SU(2) electroweak group, sphaleron transitions at high temperatures can provide the relation between baryon asymmetry and excess of -2 charge stable species, as it was demonstrated in the case of WTC [5, 16].
After it is formed in the Standard Big Bang Nucleosynthesis (BBN), 4He screens the O−− charged particles in composite (4He++O−−) OHe “atoms” [12]. In all the models of OHe, O−− behaves either as a lepton or as a specific “heavy quark cluster" with strongly suppressed hadronic interactions.
Dark atoms offer an interesting possibility to solve the puzzles of direct and indirect dark matter searches. Here following Khlopov [1], Bulekov et al. [17], Gani et al. [18], and Khlopov [19] we review main features of dark atom cosmology and possible direct and indirect probes for its physical basis.
2. Dark Atom Cosmology
The cosmological scenario of the OHe Universe involves only one parameter of new physics−the mass of O−−. Such a scenario is insensitive to the properties of O−− (except for its mass), since the main features of the OHe dark atoms are determined by their nuclear interacting helium shell. In the scenario of OHe Universe, presented below it is assumed that elastic processes dominate in OHe-nucleus interactions.
Due to elastic nuclear interactions of its helium constituent with nuclei in the cosmic plasma, the O-helium gas is in thermal equilibrium with plasma and radiation on the Radiation Dominance (RD) stage, while the energy and momentum transfer from plasma is effective. The radiation pressure acting on the plasma is then transferred to density fluctuations of the O-helium gas and transforms them in acoustic waves at scales up to the size of the horizon.
At temperature
the energy and momentum transfer from baryons to O-helium is not effective [5, 12] because
where mo is the mass of the OHe atom and
Here
and is the baryon thermal velocity. Then O-helium gas decouples from plasma. It starts to dominate in the Universe after t~1012s at T ≤ TRM≈1eV, where TRM is the temperature at the transition from Radiation to Matter dominated stage. At Matter dominated stage O-helium “atoms" play the main dynamical role in the development of gravitational instability, triggering the large scale structure formation. The composite nature of O-helium determines the specifics of the corresponding dark matter scenario [1].
At Radiation dominated stage, corresponding to T>TRM, the total mass M of the OHe gas with density
is equal within the cosmological horizon lh = t to
In the period of decoupling T = Tod, this mass depends strongly on the O-helium mass S3 and is given by Khlopov and Kouvaris [5]
where M⊙ is the solar mass. O-helium is formed only at To and its total mass within the cosmological horizon in the period of its creation is
On the RD stage before decoupling, the Jeans length λJ of the OHe gas was restricted from below by the propagation of sound waves in plasma with a relativistic equation of state p = ϵ/3, being of the order of the cosmological horizon and equal to
After decoupling at T = Tod, it falls down to λJ~vot, where Though after decoupling the Jeans mass in the OHe gas correspondingly falls down
one should expect a strong suppression of fluctuations on scales M<Mo, as well as adiabatic damping of sound waves in the RD plasma for scales Mo<M<Mod. It can provide some suppression of small scale structure in the considered model for all reasonable masses of O-helium. The significance of this suppression and its effect on the structure formation needs a special study in detailed numerical simulations. In any case, it can not be as strong as the free streaming suppression in ordinary Warm Dark Matter (WDM) scenarios, but one can expect that qualitatively we deal with Warmer Than Cold Dark Matter model.
Being decoupled from baryonic matter, the OHe gas does not follow the formation of baryonic astrophysical objects (stars, planets, molecular clouds…) and forms dark matter halos of galaxies. It can be easily seen that O-helium gas is collisionless for its number density, saturating galactic dark matter. Taking the average density of baryonic matter one can also find that the Galaxy as a whole is transparent for O-helium in spite of its nuclear interaction. Only individual baryonic objects like stars and planets are opaque for it.
3. Dark Atoms and Puzzles of Direct Dark Matter Searches
In terrestrial matter O-helium atoms are slowed down and cannot cause significant nuclear recoil in the underground detectors, making them elusive in direct WIMP search experiments (where detection is based on nuclear recoil) such as CDMS, XENON100, LUX etc. The positive results of DAMA experiments (see [20] for review and references) can find in this scenario a nontrivial explanation due to a low energy radiative capture of OHe by intermediate mass nuclei [1].
At each underground level of the terrestrial matter local OHe concentration is determined by the equilibrium between incoming cosmic flux and OHe diffusion toward the center of Earth. Rapid adjustment (within an hour at the level of underground detectors) leads to its annual modulation due to the Earth's motion around the Sun, reflecting the annual modulation of the incoming cosmic flux, therefore the positive result of DAMA/NaI and DAMA/LIBRA experiments is explained by annual modulation in reaction of radiative capture of OHe
by nuclei in DAMA detector.
The crucial point in this explanation is the existence of a low lying energy level of OHe-nucleus bound state and annual modulation of energy release in OHe-nucleus capture to this level. This point needs proper quantum mechanical treatment, which appears to be not so simple due to complication of self-consistent treatment of interaction of helium shell of O-helium with the nucleus. However, in the square well and wall approximation it was shown [1] that there exists a few keV level for intermediate mass nuclei and such level doesn't exist for light and heavy nuclei. The positive result of DAMA/NaI and DAMA/LIBRA experiments follows then from annual modulation of the rate of Na capture by OHe to the 3 keV bound state of OHe-Na system.
This explains the negative results of the XENON100 and LUX experiments, since there is no such level in OHe-Xe system.
The rate of OHe capture by intermediate mass nuclei is determined by isospin violating electric dipole transition and thus proportional to the temperature. It leads to suppression of this effect in cryogenic detectors.
3.1. Inelastic Processes and O-Nuclearites
One should note that the nuclear physics of OHe is still in the course of development and its basic element for a successful and self-consistent OHe dark matter scenario is related to the existence of a dipole Coulomb barrier, arising in the process of OHe-nucleus interaction and providing the dominance of elastic collisions of OHe with nuclei.
Though OHe scenario seems to involve dominantly known atomic and nuclear physics, there is no small parameter in OHe-nucleus interaction. It makes the problem very complicated as compared with ordinary atoms that have electroweakly interacting electronic shells and strongly interacting nuclei of much smaller size. These two features proved small parameters in the ordinary atomic physics, which are not the case for OHe. Indeed, the size of the He shell is equal to the size of He nucleus and creation of barrier in OHe-nucleus interaction should be the result of a simultaneous action of nuclear attraction and Coulomb repulsion between He and approaching nucleus. Qualitatively, a possibility for such a solution can be illustrated by the following picture. At large distances Coulomb field of nucleus polarizes OHe so that Stark-effect attraction takes place. At some distance nuclear attraction changes the sign of polarization, but Coulomb repulsion moves it back, and then nuclear attraction moves He again toward nucleus and so on. Such oscillatory behavior can provide creation of potential barrier and this approach is now under quantitative investigation.
This problem, which implies correct quantum mechanical treatment, is the main open question of the composite dark matter [21]. The lack of such a barrier and essential contribution of inelastic OHe-nucleus processes seem to lead to inevitable overproduction of anomalous isotopes [22]. In that case one may need more sophisticated models retaining the ideas of dark atom scenario, which involve more elements of new physics, as e.g., proposed in Wallemacq [23].
On the other hand, even strongly suppressed inelastic processes inevitably lead to formation of anomalous isotopes due to O−− capture by nuclei. Capture by nucleus of multiple O−− particles can lead to existence of exotic superheavy nuclear states of O-nuclearites [18].
In the difference from early studies of bound systems of stable heavy negatively charged particles with nuclei [24–26] the approach of Gani et al. [18] is concentrated on effect of multiple O-particles capture by nuclei.
According Gani et al. [18] the most energetically favorable multi-O-particle distribution inside the nucleus with a density nO(r) is that follows the proton one, fully compensating the Coulomb field. Thereby O-particles, if their number were NO≥A, would be re-distributed to minimize the energy, and finally the density of O inside the atomic nucleus with radius R becomes
with
for O-nuclearite, that corresponds to V = const for r < R. Here np is proton density in the nucleus that is assumed constant inside the nucleaus. Excessive O-particles are pushed out. Thus, constructed O-nuclearite, has the energy
and thereby for arbitrary A it proves to be absolutely stable (if O is considered as a stable particle), till gravity is yet unimportant. The key point here is that it is profitable to have nO(r) = 2np(r), if there is a sufficient amount of O-particles.
Note that the value
and thereby the matter of the nuclearite is self-bound provided mo > 2.3mN, where mN is the mass of nucleon.
O-nuclearites can hardly be formed in the early Universe but their formation is possible in stars due to O-helium capture and the produced O-nuclearites can be released in the interstellar space after supernova explosions. If the amount of O-particles captured by star is sufficiently large, it can trigger collapse to black hole and according to de Lavallaz and Fairbairn [27] provide additional constraints on O−− particles. Such amount cannot be captured by neutron star owing to its small size, but can be stored at earlier stages of stellar evolution, when the star had the size of giant or supergiant [18].
4. Indirect Effects of Composite Dark Matter
The existence of such form of matter as O-helium should lead to a number of astrophysical signatures, which can constrain or prove this hypothesis. One of the signatures of O-helium can be a presence of an anomalous low Z/A component in the cosmic ray flux. O-helium atoms that are present in the Galaxy in the form of the dark matter can be destroyed in astrophysical processes and free double charged particles can be accelerated as ordinary charged particles. O-helium atoms can be ionized due to nuclear interaction with cosmic rays or in the front of a shock wave in the Supernova explosions, where they were effectively accumulated during star evolution [12]. If the mechanisms of X acceleration are effective, they might be observed in AMS02 cosmic ray experiments.
4.1. Excess of Positron Annihilation Line in the Galactic Bulge
OHe collisions in the central part of the Galaxy lead to OHe excitations, and de-excitations with pair production in E0 transitions can explain the excess of the positron-annihilation line, observed by INTEGRAL in the galactic bulge [1, 16, 28].
If 2S level of O-helium is excited, its direct one-photon transition to the 1S ground state is forbidden and the de-excitation mainly goes through direct pair production. In principle this mechanism of positron production can explain the excess in positron annihilation line from the galactic bulge, measured by the INTEGRAL experiment. Due to the large uncertainty of DM distribution in the galactic bulge this interpretation of the INTEGRAL data is possible in a wide range of masses of O-helium with the minimal required central density of O-helium dark matter at [29, 30] mo = 1.25TeV For the smaller values of mo on needs larger central density to provide effective excitation of O-helium in collisions Current analysis favors lowest values of central dark matter density, making possible O-helium explanation for this excess only for a narrow window around this minimal value (see Figure 1).
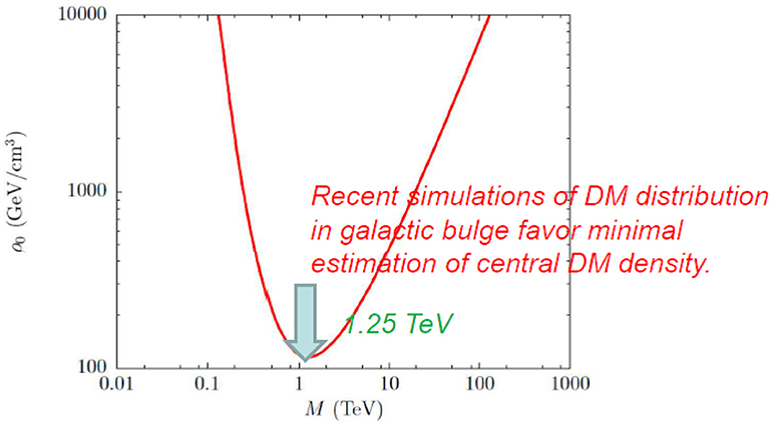
Figure 1. Dark matter is subdominant in the central part of Galaxy. It strongly suppresses it dynamical effect and causes large uncertainty in dark matter density and velocity distribution. At this uncertainty one can explain the positron line excess, observed by INTERGRAL, for a wide range of mo given by the curve with minimum at mo = 1.25TeV. However, recent analysis of possible dark matter distribution in the galactic bulge favor minimal value of its central density. The picture is taken with the according permission from Bulekov et al. [17].
4.2. Dark Atom Solution for High Energy Positron Excess
In a two-component dark atom model, based on Walking Technicolor, atom-like states, made of positive and negative doubly charged techniparticles, is present together with the dominant component of OHe dark atom. These techniparticle atoms weakly interact with matter and the upper limits from direct dark matter searches put constraints on this sparse WIMP-like dark atom component. In WTC models positively double charged particles may be very long living, but metastable and their decays to pairs of same-sign leptons can explain the excess of high-energy cosmic-ray positrons [31], found in PAMELA and AMS02 experiments [32–35]. This explanation is possible for the mass of decaying +2 charged particle below 1 TeV and depends on the branching ratios of leptonic channels (see Figure 2).
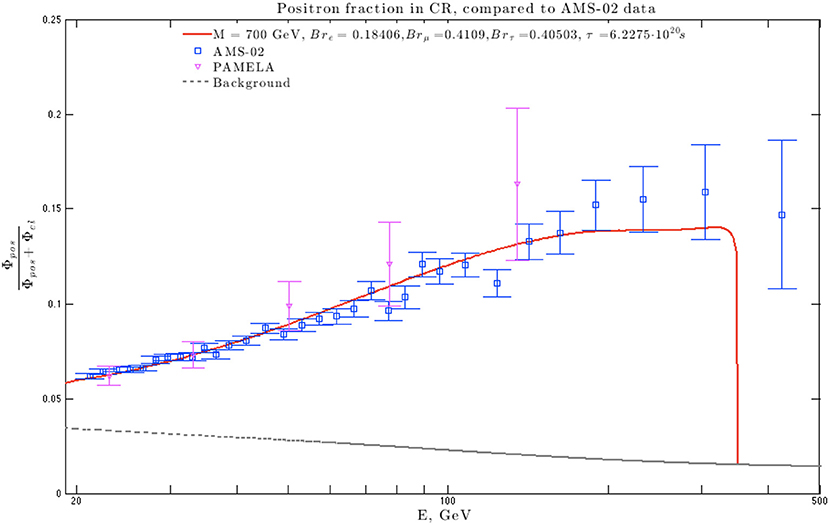
Figure 2. Best fit high energy positron fluxes from decaying composite dark matter in confrontation with the results of AMS02 experiment. The picture is taken with the according permission from Bulekov et al. [17].
Since even pure lepton decay channels are inevitably accompanied by gamma radiation the important constraint on this model follows from the measurement of cosmic gamma ray background in FERMI/LAT experiment [36] (see Figure 3). The multi-parameter analysis of decaying dark atom constituent model determines the maximal model independent value of the mass of decaying +2 charge particle, at which this explanation is possible
One should take into account that according to Belotsky et al. [37] even in this range hypothesis on decaying composite dark matter, distributed in the galactic halo, can lead to gamma ray flux exceeding the measured by FERMI/LAT. It may favor local source of the positron component e.g., from the decays in clumps of dark matter in vicinity of the Solar system. One should also note an alternative non-dark-matter nature of such a local source as a local supernova explosion two million years ago [38].
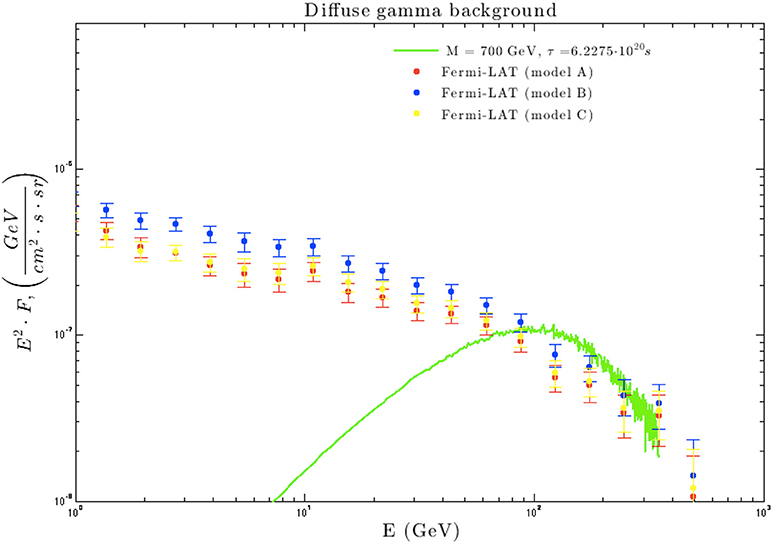
Figure 3. Gamma ray flux accompanying the best fit high energy positron fluxes from decaying composite dark matter reproducing the results of AMS02 experiment, in confrontation with FERMI/LAT measurement of gamma ray background. The picture is taken with the according permission from Bulekov et al. [17].
4.3. Sensitivity of Indirect Effect of Composite Dark Matter to the Mass of Their Double Charged Constituents
We see that indirect effects of composite dark matter strongly depend on the mass of its double charged constituents.
To explain the excess of positron annihilation line in the galactic bulge mass of double charged constituent of O-helium should be in a narrow window around
To explain the excess of high energy cosmic ray positrons by decays of constituents of composite dark matter with charge +2 and to avoid overproduction of gamma background, accompanying such decays, the mass of such constituent should be in the range
These predictions should be confronted with the experimental data on the accelerator search for stable double charged particles.
5. Searches for Stable Double Charged Particles
Production of pairs of elementary stable doubly charged heavy leptons is characterized by a number of distinct experimental signatures that would provide effective search for them at the experiments at the LHC and test the atom-like structure of the cosmological dark matter. Moreover, astrophysical consequences of composite dark matter model can reproduce experimentally detected excess in positron annihilation line and high energy positron fraction in cosmic rays only if the mass of double charged O−− particles is in the 1 TeV range. Here we discuss following Bulekov et al. [17] confrontation of these predictions and experimental data, as well as the current status and expected progress in experimental searches for stable double charged particles as constituents of composite dark matter.
A new charged massive particle with electric charge ≠1e would represent a dramatic deviation from the predictions of the Standard Model, and such a spectacular discovery would lead to fundamental insights and critical theoretical developments. Searches for such kind of particles were carried out in many cosmic ray and collider experiments (see for instance review in [39]).
In a tree approximation, such particles cannot decay to pair of quarks due to electric charge conservation and only decays to the same sign leptons are possible. The latter implies lepton number nonconservation, being a profound signature of new physics. In general, it makes such states sufficiently long-living in a cosmological scale.
Obviously, such experiments are limited to look only for the “long-lived” particles, which do not decay within a detector, as opposed to truly stable particles, which do not decay at all. Since the kinematics and cross sections for double charged particle production processes cannot be reliably predicted, search results at collider experiments are usually quoted as cross section limits for a range of charges and masses for well-defined kinematic models. In these early experiments, the mass limit was set at the level of up to 100 GeV (see for review [39]).
5.1. Searches at Large Hadron Collider
Significant increase of beam energy at the Large Hadron Collider (LHC) opens a new era in the high energy physics and allows accessing uncharted territories of particle masses. In this subsection the results of searches for the multi-charged particles, performed by the ATLAS and the CMS collaborations at LHC, will be described following Bulekov et al. [17].
Calculations of the cross sections assume that these particles are generated as new massive spin-1/2 ones, neutral under SU(3)C and SU(2)L.
5.1.1. ATLAS Experiment at LHC
In Run 1 (2010–2012), the ATLAS [40] collaboration at LHC performed two searches for long-lived multi-charged particles, including the double charged particles: one search with 4.4 fb−1 of data collected in pp collisions at TeV [41], and another one with 20.3 fb−1 collected at TeV [42].
Both these searches feature particles with large transverse momentum values, traversing the entire ATLAS detector. An energy loss of a double charged particle is by a factor of q2 = 4 higher than that of single charged particle. Such particles will leave a very characteristic signature of high ionization in the detector. More specifically, the searches look for particles with anomalously high ionization on their tracks in three independent detector subsystems: silicon pixel detector (Pixel) and transition radiation tracker (TRT) in the ATLAS inner detector, and monitoring drift tubes (MDT) in the muon system.
The estimate of the expected background originating from the SM processes and providing input into the signal region D was calculated to be 0.013±0.002(stat.)±0.003(syst.) events.
No events with double charged particles were found in neither 2011 or 2012 experimental data sets, setting the lower mass limits to 430 and 660 GeV, respectively, at 95% CL. The comparison of observed cross-section upper limits and theoretically predicted cross-sections is shown in Figure 4.
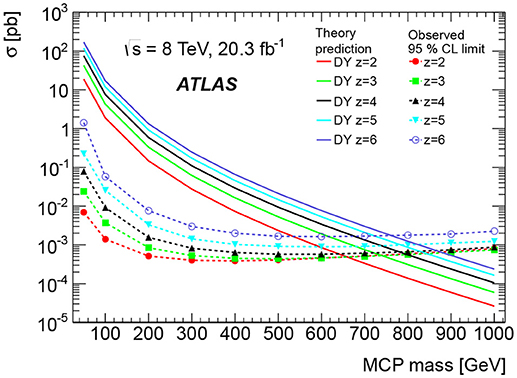
Figure 4. Observed 95% CL cross-section upper limits and theoretical cross-sections as functions of the multi-charged particles mass. Again, the double charged particles are denoted as “z = 2” (red points and lines). The picture is taken with the according permission from Bulekov et al. [17].
5.1.2. CMS Experiment at LHC
In parallel to the ATLAS experiment, the CMS [43] collaboration at LHC performed a search for double charged particles, using 5.0 fb−1 of data collected in pp collisions at TeV and 18.8 fb−1 collected at TeV [44].
Comparison between observed upper cross section limits and theoretically predicted cross section values for the 8 TeV is shown in Figure 5.
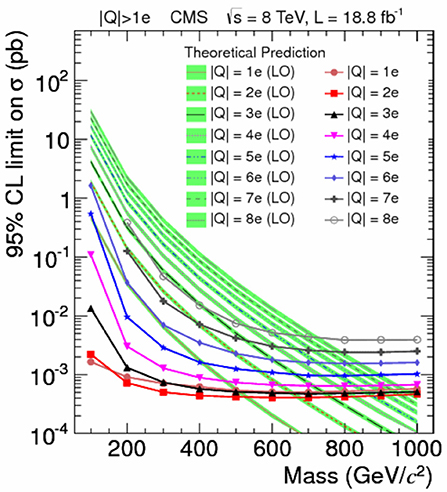
Figure 5. Observed 95% CL cross-section upper limits and theoretical cross-sections as functions of the multi-charged particles mass in CMS search at the TeV. The double charged particles are denoted as “|Q| = 2e”. The picture is taken with the according permission from Bulekov et al. [17].
For the 8 TeV search, the mass limit of 665 GeV was obtained. This result (within uncertainties) is very close to the ATLAS limit of 660 GeV for the 8 TeV data set. Also, CMS treated the results obtained at 7 and 8 TeV as combined. This allowed to push the lower mass limit to 685 GeV at 95% CL. A combination of the results of two experiments for 8 TeV would mean an increase of statistics by a factor of 2. Having said that, one can conclude that the mass limit based on the results of both experiment for double charged particles can be set at the level of about 730 GeV.
5.2. What One Expects From LHC Run 2
Following the discussion of the prospects of searches for multi-charged particles in ATLAS and CMS experiments in Bulekov et al. [17], it may be concluded that each of these two experiments will be able to set a lower mass limit on the double charged particles at m = 1000±50 GeV. Going further and considering the data taking periods of ATLAS and CMS until the end of Run 2 (end of 2018), one can estimate according to Bulekov et al. [17] a low limit on the double charged particles mass corresponding to the Run 2 data set. Several assumptions were made in these speculations [17]:
• by the end of 2018, ATLAS and CMS will each record about 120 fb−1 of TeV data;
• signal efficiency will remain at a present level in both experiments, without being compromised by high detector occupancy or any other effects;
• no double charged particle candidates will be in observed in the first place.
Considering all of the above, the ATLAS and CMS collaborations may each be expected to set the lower mass limits at the level of 1.2 TeV based on their analyses of the entire 13 TeV data set. If these two experiments combined their independently gathered statistics together for this kind of search, the limits would go as high as up to 1.3 TeV [17].
6. Conclusions
Dark Matter that is considered to be electrically neutral, potentially can be formed by stable heavy charged particles bound in neutral atom-like states by Coulomb attraction. Analysis of the cosmological data and atomic composition of the Universe gives the constrains on the particle charge showing that only -2 charged constituents, being trapped by primordial helium in neutral O-helium states, can avoid the problem of overproduction of the anomalous isotopes of chemical elements, which are severely constrained by observations.
Cosmological model of O-helium dark matter can even explain puzzles of direct dark matter searches. This explanation involves minimal number of parameters of new physics, being based on known laws of quantum mechanics and atomic and nuclear physics. However, we have seen that the nontrivial features of OHe-nucleus interaction still leave open the crucial question on the existence and effect of dipole potential barrier in this interaction. Such barrier provides dominance of elastic processes in OHe interaction with matter and existence of shallow potential well in OHe interaction with intermediate mass nuclei. Existence of a 3 keV level in such a well in OHe-Na interaction and annual modulation of energy release due to capture to this level can explain positive result of DAMA/NaI and DAMA/LIBRA experiments, as well as absence of such signal in other detectors.
O-helium hypothesis inevitably leads to existence of anomalous nuclear isotopes, in which single O−− is captured, or to exotic states of O-nuclearites with multiple O-particles in nuclei. Search for such anomalous form of nuclear matter extends the set of probes for dark-atom hypothesis.
The crucial problem of OHe scenario is the nuclear physics of OHe. Though it involves only known atomic and nuclear physics the proper quantum mechanical treatment is very complicated in the lack of small parameters in the problem of OHe-nucleus interaction. Such treatment should also take into proper account simultaneous action of Coulomb and nuclear forces on He from the approaching nucleus. There is a hint to solve this problem and the development of the quantitative analysis is under way. As soon as this problem is solved all the richness of the astrophysical features of OHe scenario will find proper physical basis (see e.g., [45]).
OHe hypothesis can also explain observed excess of positron annihilation line in the galactic bulge and high energy positron fraction of cosmic rays. Such explanation implies upper limits on the mass of a double charged constituents within 1 TeV range, challenging their searches at the LHC.
Stable charge -2 states (O−−) can be elementary like AC-leptons or technileptons, or look like technibaryons. The latter, composed of techniquarks, reveal their structure at much higher energy scale and should be produced at colliders and accelerators as elementary species. They can also be composite like “heavy quark clusters” ŪŪŪ formed by anti-U quark in one of the models of fourth generation [12] or ū5ū5ū5 of (anti)quarks ū5 of stable 5th family in the approach [13].
In the context of composite dark matter scenario accelerator search for stable doubly charged leptons acquires the meaning of direct critical test for existence of charged constituents of cosmological dark matter.
The signature for AC leptons and techniparticles is unique and distinctive what allows to separate them from other hypothetical exotic particles. Composite dark matter models can explain observed excess of high energy positrons and gamma radiation in positron annihilation line at the masses of O−− in the range of 1 TeV, it makes search for double charged particles in this range direct experimental test for these predictions of composite dark matter models.
Test for composite O−− can be only indirect: through the search for heavy hadrons, composed of single U or Ū and light quarks (similar to R-hadrons).
The ATLAS and CMS collaborations at the Large Hadron Collider are searching for the double charged particles since 2011. The most stringent results achieved so far exclude the existence of such particles up to their mass of 680 GeV. This value was obtained by both ATLAS and CMS collaborations independently. It is expected that if these two collaborations combine their independently gathered statistics of LHC Run 2 (2015–2018), the lower mass limit of double charged particles could reach the level of about 1.3 TeV. It can provide severe test for composite dark matter origin of possible indirect effects of dark matter.
One can conclude that signatures of composite dark matter provide an extensive set of direct and indirect probes challenging their physical and astrophysical test.
Author Contributions
The author confirms being the sole contributor of this work and has approved it for publication.
Conflict of Interest Statement
The author declares that the research was conducted in the absence of any commercial or financial relationships that could be construed as a potential conflict of interest.
Acknowledgments
I express my gratitude to K. Belotsky, O. Bulekov, J. R. Cudell, C. Kouvaris, M. Laletin, A. S. Romaniouk, and Yu. S. Smirnov for their collaboration in obtaining original results on which this review is based. The paper involves with proper references the results published by author in Khlopov [1], Bulekov et al. [17], Gani et al. [18], and Khlopov [19]. The work was supported by grant of Russian Science Foundation (project N-18-12-00213).
References
1. Khlopov MY. Fundamental particle structure in the cosmological dark matter. Int J Mod Phys. (2013) 28:1330042. doi: 10.1142/S0217751X13300421
3. Fargion D, Khlopov M, Stephan CA. Cold dark matter by heavy double charged leptons? Class Quantum Grav. (2006) 23:7305. doi: 10.1088/0264-9381/23/24/008
5. Khlopov MY, Kouvaris C. Strong interactive massive particles from a strong coupled theory. Phys Rev D (2008) 77:065002. doi: 10.1103/PhysRevD.77.065002
6. Sannino F, Tuominen K. Orientifold theory dynamics and symmetry breaking. Phys Rev D (2005) 71:051901. doi: 10.1103/PhysRevD.71.051901
7. Hong DK, Hsu SD, Sannino F. Composite Higgs from higher representations. Phys Lett B (2004) 597:89. doi: 10.1016/j.physletb.2004.07.007
8. Dietrich DD, Sannino F, Tuominen K. Light composite Higgs from higher representations versus electroweak precision measurements: predictions for LHC. Phys Rev D (2005) 72:055001. doi: 10.1103/PhysRevD.72.055001
9. Dietrich DD, Sannino F, Tuominen K. Light composite Higgs and precision electroweak measurements on the Z resonance: an update. Phys Rev D (2006) 73 :037701. doi: 10.1103/PhysRevD.73.037701
10. Gudnason SB, Kouvaris C, Sannino F Towards working technicolor: effective theories and dark matter. Phys Rev D (2006) 73:115003. doi: 10.1103/PhysRevD.73.115003
11. Gudnason SB, Kouvaris C, Sannino F. Dark matter from new technicolor theories. Phys Rev D (2006) 74:095008. doi: 10.1103/PhysRevD.74.095008
12. Khlopov MY. Composite dark matter from 4th generation. JETP Lett. (2006) 83:1–4. doi: 10.1134/S0021364006010012
13. Mankoc-Borstnik N. Unification of spins and charges in Grassmann space? Mod Phys Lett A (1995) 10:587. doi: 10.1142/S0217732395000624
15. Khlopov MY, Shibaev RM. Probes for 4th generation constituents of dark atoms in Higgs boson studies at the LHC. Adv High Ener Phys. (2014) 2014:406458. doi: 10.1155/2014/406458
16. Khlopov MY, Kouvaris C. Composite dark matter from a model with composite Higgs boson. Phys Rev D (2008) 78:065040 doi: 10.1103/PhysRevD.78.065040
17. Bulekov OV, Khlopov MY, Romaniouk AS, Smirnov YS. Search for double charged particles as direct test for dark atom constituents. Bled Works Phys. (2017) 18:11.
18. Gani VA, Khlopov MY, Voskresensky DN. Double charged heavy constituents of dark atoms and superheavy nuclear objects. Phys Rev D (2019) 99:015024. doi: 10.1103/PhysRevD.99.015024
19. Khlopov MY. Probes for dark matter physics. Int J Mod Phys D (2018) 27:1841013. doi: 10.1142/S0218271818410134
20. Bernabei R, Belli P, d'Angelo S, Di Marco A, Montecchia F, Cappella F, et al Dark Matter investigation by DAMA at Gran Sasso. Int J Mod Phys A (2013) 28:1330022. doi: 10.1142/S0217751X13300226
22. Cudell JR, Khlopov MY, Wallemacq Q. Some potential problems of OHe composite dark matter. Bled Works Phys.(2014) 15:66.
23. Wallemacq Q. Milli-interacting dark matter. Phys Rev D (2013) 88:063516. doi: 10.1103/PhysRevD.88.063516
24. Cahn RN, Glashow SL. Chemical signatures for superheavy elementary particles. Science (1981) 213:607. doi: 10.1126/science.213.4508.607
25. Pospelov M. Particle physics catalysis of thermal big bang nucleosynthesis. Phys Rev Lett. (2007) 98:231301. doi: 10.1103/PhysRevLett.98.231301
26. Kohri K, Takayama F. Big bang nucleosynthesis with long-lived charged massive particles. Phys Rev. (2007) D76:063507. doi: 10.1103/PhysRevD.76.063507
27. de Lavallaz A, Fairbairn M. Neutron stars as dark matter probes. Phys Rev. (2010) D81:123521.doi: 10.1103/PhysRevD.81.123521
28. Cudell JR, Khlopov MY, Wallemacq Q. Dark atoms and the positron-annihilation-line excess in the galactic bulge. Adv High Ener Phys. (2014) 2014:869425. doi: 10.1155/2014/869425
29. Finkbeiner DP, Weiner N. Exciting dark matter and the INTEGRAL/SPI 511 keV signal. Phys Rev. (2007) D76:083519. doi: 10.1103/PhysRevD.76.083519
30. Teegarden BJ, Watanabe K, Jean P, Knödlseder J, Lonjou V, Roques JP, et al. INTEGRAL/SPI limits on electron-positron annihilation radiation from the galactic plane. Astrophys. J. (2005) 621:296–300. doi: 10.1086/426859
31. Belotsky K, Khlopov M, Kouvaris C, Laletin M. Decaying Dark Atom constituents and cosmic positron excess. Adv High Ener Phys. (2014) 2014:214258. doi: 10.1155/2014/214258
32. Adriani O, Barbarino GC, Bazilevskaya GA, Bellotti R, Bianco A, Boezio M, et al. Cosmic-Ray Positron Energy Spectrum Measured by PAMELA. Phys Rev Lett. (2013) 111:081102. doi: 10.1103/PhysRevLett.111.081102
33. Aguilar M, Alberti G, Alpat B, Alvino A, Ambrosi G, Andeen K, et al. First result from the alpha magnetic spectrometer on the international space station: precision measurement of the positron fraction in primary cosmic rays of 0.5-350 GeV. Phys Rev Lett. (2013) 110:141102. doi: 10.1103/PhysRevLett.110.141102
34. Aguilar M, Aisa D, Alvino A, Ambrosi G, Andeen K, Arruda L, et al. Electron and positron fluxes in primary cosmic rays measured with the alpha magnetic spectrometer on the international space station. Phys Rev Lett. (2014) 113:121102. doi: 10.1103/PhysRevLett.113.121102
35. Accardo L, Aguilar M, Aisa D, Alpat B, Alvino A, Ambrosi G, et al. High statistics measurement of the positron fraction in primary cosmic rays of 0.5-500 GeV with the alpha magnetic spectrometer on the international space station. Phys Rev Lett. (2014) 113:121101. doi: 10.1103/PhysRevLett.113.121101
36. Ackermann M, Ajello M, Albert A, Baldini L, Barbiellini G, Bechtol K, et al. Fermi LAT search for dark matter in gamma-ray lines and the inclusive photon spectrum. Phys Rev. (2012) D86:022002. doi: 10.1103/PhysRevD.86.022002
37. Belotsky K, Budaev R, Kirillov A, Laletin M. Fermi-LAT kills dark matter interpretations of AMS-02 data. Or not? J Cosmol Astropart Phys. (2017) 1701:021. doi: 10.1088/1475-7516/2017/01/021
38. Kachelriess M, Neronov A, Semikoz DV. Signatures of a two million year old supernova in the spectra of cosmic ray protons, antiprotons and positrons. Phys Rev Lett. (2015) 115:181103. doi: 10.1103/PhysRevLett.115.181103
39. Fairbairn M, Kraan AC, Milstead DA, Sjostrand T, Skands P, Sloan T. Stable massive particles at colliders. Phys Rep. (2007) 438:1. doi: 10.1016/j.physrep.2006.10.002
40. Aad G, Abat E, Abdallah J, Abdelalim AA, Abdesselam A, Abdinov Q, et al. The ATLAS experiment at the CERN large hadron collider. J Instrumet. (2008) 3:S08003. doi: 10.1088/1748-0221/3/08/S08003
41. Aad G, Abajyan T, Abbott B, Abdallah J, Abdel Khalek S, Abdelalim AA, et al. Search for long-lived, multi-charged particles in pp collisions at TeV using the ATLAS detector. Phys Lett B (2013) 722:305. doi: 10.1016/j.physletb.2013.04.036
42. Aad G, Abbott B, Abdallah J, Abdinov O, Aben R, Abolins M, et al. Search for heavy long-lived multi-charged particles in pp collisions at TeV using the ATLAS detector. Eur Phys J C (2015) 75:362. doi: 10.1140/epjc/s10052-015-3534-2
43. Chatrchyan S, Hmayakyan G, Khachatryan V, Sirunyan AM, Adam W, Bauer T, et al. The CMS experiment at the CERN LHC. J Instrument. (2008) 3:S08004. doi: 10.1088/1748-0221/3/08/S08004
44. Chatrchyan S, Khachatryan V, Sirunyan AM, Tumasyan A, Adam W, Bauer T, et al. Searches for long-lived charged particles in pp collisions at and 8 TeV. J High Energy Phys. (2013) 1307:122. doi: 10.1007/JHEP07(2013)122
Keywords: elementary particles, dark matter, dark atoms, stable double charged particles, composite dark matter
Citation: Khlopov MY (2019) Direct and Indirect Probes for Composite Dark Matter. Front. Phys. 7:4. doi: 10.3389/fphy.2019.00004
Received: 19 September 2018; Accepted: 09 January 2019;
Published: 29 January 2019.
Edited by:
Roman Pasechnik, Lund University, SwedenReviewed by:
Choong Sun Kim, Yonsei University, South KoreaAlexander Merle, Max-Planck-Institut für Physik, Germany
Giacomo Cacciapaglia, UMR5822 Institut de Physique Nucleaire de Lyon (IPNL), France
Copyright © 2019 Khlopov. This is an open-access article distributed under the terms of the Creative Commons Attribution License (CC BY). The use, distribution or reproduction in other forums is permitted, provided the original author(s) and the copyright owner(s) are credited and that the original publication in this journal is cited, in accordance with accepted academic practice. No use, distribution or reproduction is permitted which does not comply with these terms.
*Correspondence: Maxim Y. Khlopov, a2hsb3BvdkBhcGMudW5pdi1wYXJpczcuZnI=