- 1Department Therapeutic Radiology and Dermatology, Yale School of Medicine, New Haven, CT, United States
- 2Department Chemistry and Biochemistry, Northern Illinois University, DeKalb, IL, United States
Quantum biology typically involves light exciting an electron to a higher energy state, or a magnetic field splitting a single state into several having different energies, or tunneling to flout the energy barrier between states. In chemiexcitation, electrons reach an excited state without light. A ground-state chemical reaction creates a reaction product born in the excited state, due to transient mixing between ground- and excited-state wavefunctions when the reaction intermediate molecule is twisted. We outline the chemiexcitation process and its biological triggers, describe the distinctive molecules susceptible to chemiexcitation, and review recent evidence that melanin in the human retina is chemiexcited as a strategy to prevent age-related macular degeneration.
1 Introduction
In ordinary chemistry, molecular collisions excite a molecule’s vibrational or rotational states to an energy that overcomes an activation energy barrier and rearranges the sharing of bonded electron pairs in their electronic ground state. Even redox reactions remain in the ground state. Photosynthesis reaches 30-fold higher energies, using a photon to excite an electron from the ground state to a high-energy orbital. High energy levels also have altered orbital shapes. This combination allows reactions that are quantum forbidden in body-temperature biochemistry. A classic example occurs in DNA when UV excitation joins adjacent cytosines or thymines by concerted formation of two bonds—the cyclobutane pyrimidine dimer (CPD) that is lethal, mutagenic, and carcinogenic (Brash, 2015; Schreier et al., 2015).
If, in addition, the excited electron’s spin flips, the typical singlet state is converted to a triplet. This spin flip is also forbidden, making it likely in only special molecules and functional groups. It is enabled by spin mixing between the two states (Turro and Kraeutler, 1980; Woodward, 2002). Decay back to the singlet ground state is also forbidden, resulting in the triplet state having a much longer lifetime than the excited singlet state (msec vs. nsec). This million fold longer lifetime allows a triplet excited state molecule to transfer its energy to an acceptor or react chemically. O2 is interesting because its ground state electronic configuration is a triplet. Ambient O2 therefore reacts with triplet excited states via energy transfer to form highly reactive singlet oxygen.
2 Chemiexcitation
Chemiexcitation is instead a chemical reaction, one in which a ground-state reactant creates a product molecule born in the excited state (>3 eV, 70 kcal/mol, 40,000 K) rather than being excited from the ground state. In biology, a prior reaction with oxygen creates a moiety termed a dioxetane (Figure 1A), a strained–C–O–O–C–ring, or a version of it, that has unusual chemical and quantum mechanical properties (Adam and Cilento, 1982; Brash and Goncalves, 2023). When its host molecule is twisted by ambient thermal energy, its energy level surfaces assume unusual shapes (Figure 1B). In the chemiexcitation reaction per se, twisting causes the ground state singlet energy level to increase and the excited state triplet energy level to decrease, with the two meeting at a conical intersection or, in this case, a seam that erases the energy difference (Farahani et al., 2013). This meeting of the energy surfaces facilitates crossing from the ground state singlet to the excited triplet. The O-O and C-C bonds break to form two carbonyls, one of which acquires most of the energy. Moreover, carbonyls are excited via an n→π* transition, which favors crossing from singlet to triplet; the result is an excited triplet state carbonyl. Then as the 2 C’s separate, the singlet and triplet energies of the molecule again diverge. Ultimately, the high triplet energy came from the C–C bond, the O–O bond, and free radical or singlet oxygen that created the strained dioxetane. The archetype is bioluminescence in lower organisms (Wilson and Hastings, 2013). Chemiexcitation in mammals was sought for 50 years, but was not found until we discovered CPDs generated in the dark (“dark CPDs,” dCPD) by chemiexcitation of the pigment melanin in skin melanocytes long after UV radiation ended (Premi et al., 2015; Delinasios et al., 2018; Lawrence et al., 2018; Fajuyigbe et al., 2021).
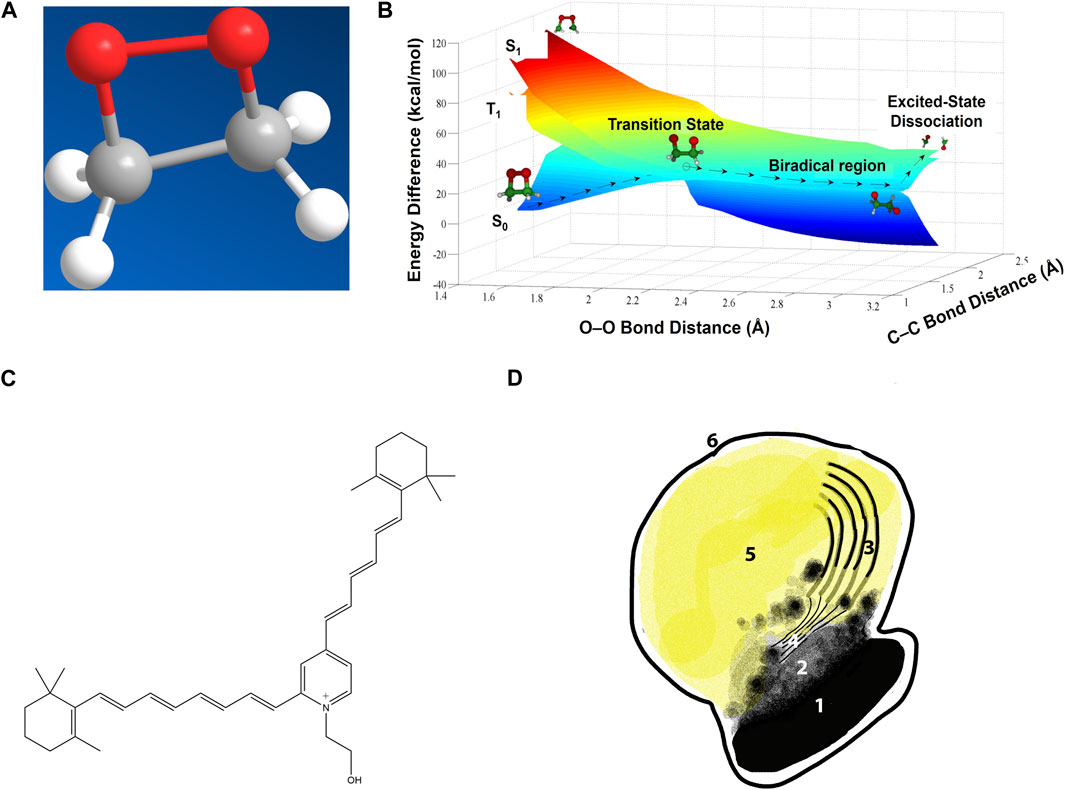
Figure 1. Chemiexcitation and the lipofuscin underlying macular degeneration. (A) A dioxetane, a strained and high-energy cyclic peroxide. Adapted from (Brash and Goncalves, 2023). (B) Potential energy surfaces for dioxetane cleavage. Heat-induced vibration twists the two Os apart at an increasingly large angle, stretching the O–O bond and increasing the dioxetane’s ground-state energy S0 until it matches the energy of an excited triplet state T1. This match allows easy intersystem crossing to T1 along the transition state seam. Further stretching lowers the ground-state energy and cleaves the C–C bond. The reaction product with two carbonyls is then born already in the excited state. (Dark blue region on right is the underside of the ground state surface which, on the left, is seen from the top.) Adapted from (Farahani et al., 2013), copyright by the American Chemical Society (2013). (C) Structure of the lipofuscin bis-retinoid A2E. (D) A melanolipofuscin granule. 1, polymeric melanin within the melanosome; 2, smaller melanin units after leaking out; 3, remnants of the original disc membranes; 4, thin lamellar membranes (TLM’s); 5, homogeneous lipofuscin-like material; 6, limiting membrane of the melanolipofuscin granule. Adapted from (Lyu et al., 2023).
In melanocytes, the upstream process creating the dioxetane is that UV activates nitric oxide synthase and NADPH oxidase for hours (by unknown signaling pathways), generating the radicals ⋅NO and O2⋅- which rapidly combine to form peroxynitrite (ONOO−), which oxidizes melanin to a radical. The enzymes horseradish peroxidase and monoamine oxidase can substitute for peroxynitrite (Premi et al., 2015; Gonçalves et al., 2023). Melanin-like biomolecules like the neurotransmitters serotonin and dopamine are also subject to chemiexcitation (Gonçalves et al., 2023).
Evidence indicates that ambient O2 forms a dioxetane ring on the melanin radical. In the chemiexcitation step, the ring cleaves, leaving one of the two excited carbonyls in the triplet-state, at a UV-like energy higher than in bioluminescence. The long-lived triplet state can generate phosphorescence, transfer its energy to DNA or ambient O2 by Dexter electron exchange (exchange of a high energy electron for a low energy ground state one), or initiate a redox reaction such as electron or H abstraction (Epe et al., 1992; Brash and Goncalves, 2023). Unlike photoexcitation, chemiexcitation does not rely on the absorption spectrum of the initial molecule, carbonyl-containing product, or acceptor, allowing a wider range of acceptors, analogous to a photosensitizer’s energy transfer (Cantrell et al., 2001).
3 Melanin and neurotransmitters as chemiexcitation targets
Melanin’s role as a protective pigment in the skin stems from its exotic structure (Meredith and Sarna, 2006; Hong and Simon, 2007; Simon and Peles, 2010; Mostert et al., 2018). Its variety of subunits have different oxidation states and thus a range of energy separation between the highest occupied molecular orbital (HOMO) and the lowest unoccupied molecular orbital (LUMO). As a result, melanin absorbs light from UV to visible and possibly infrared (Kohl et al., 2019). Moreover, its energy is dissipated as heat, which in a solid is the quantized vibrations termed phonons. Melanin’s electron→phonon coupling is so strong that energy from excited electrons dissipates ≥106 fold faster as heat than by emitting light (Crippa and Viappiani, 1990). It also has highly mobile pi electrons that are easily ejected, due to a high fraction of semiquinones—stable radicals capable of 1-electron transfer. New semiquinone radicals are generated by low energy visible light because excited quinones abstract an electron from nearby hydroquinones (Felix et al., 1979). Melanin’s quinones have a very low energy LUMO, which therefore readily accepts electrons. The HOMO is only slightly lower than the LUMO, so little energy is needed for an electron to be excited (Pullman and Pullman, 1961) or ejected completely (Chedekel et al., 1978). The unpaired electrons of two nearby semiquinones can couple through the pi clouds (Blois, 1971); even ambient heat (approximately 0.01 eV) can flip the spin of one of the radicals so they have the same direction, putting the radical pair into a triplet state (Najder-Kozdrowska et al., 2010). The ease of electron excitation, a property shared with serotonin and dopamine, entails an ease of oxidation that facilitates the free radical or singlet oxygen reaction that creates the dioxetane, thereby facilitating chemiexcitation.
Melanin has served many useful purposes over the course of evolution. Yet this Swiss Army knife is double-edged because chemiexcitation can lead to CPDs and electron or H abstraction. In addition, melanin extensively binds Fe, Cu, and Zn ions (Hong and Simon, 2007), metals facilitating Fenton-like reactions in which H2O2 formed from O2⋅- abstracts an electron from the metal to make ⋅OH radicals. Other ancient indoles and catecholamines with similar properties—like serotonin, melatonin, and dopamine—will face a similar duality. Why would brains rely on such hazardous molecules? Evolution apparently arrived at this point because the useful low excitation energies evolved in the long ago low-O2 world (Azmitia, 2010). Yet, the switch to a high O2 atmosphere 2 billion years ago, with accompanying risk of oxidation, was not followed by an evolutionary search for alternative solutions. This stasis follows from the principle of antagonistic pleiotropy, that evolution cannot select against events that are harmful after the age of reproduction (Williams, 1957).
Because the retina contains melanin, assumed to reduce light scatter in the eye, it seemed that its chemiexcitation by inflammation-induced ⋅NO and O2⋅- might underlie the malady of macular degeneration (Brash et al., 2018). Yet the plot thickened.
4 Photoreceptor turnover and macular degeneration
The leading cause of vision loss in industrialized countries is age-related macular degeneration (AMD). This deterioration of central vision is concurrent with the accumulation of droplets of lipid and protein called lipofuscin in the retina, damaging cells. Effective treatments to reverse AMD are not available and it remains unclear how healthy eyes prevent this accumulation.
The starting point of this disease is the photoreceptor (Kaufmann and Han, 2024), a specialized cell in the eye that convert light into signals sent to the brain. The outer portion of these cells contain stacks of membranes called discs that are filled with rhodopsin, the molecule that captures light and converts cis-retinal to all-trans-retinal, resulting in an electrical signal. Once exposed to light, discs are shed and undergo recycling that restores all-trans-retinal to cis for reuse by newly formed discs. This process repeats daily throughout a person’s life.
But a fraction of the recycling goes awry. Occasional reaction of all-trans-retinal with the disc membrane’s phosphatidylethanolamine produces N-retinylidene-phosphatidylethanolamine, which then reacts with remaining all-trans-retinal to form bis-retinoids such as N-retinyl-N-retinylidene ethanolamine (usually abbreviated A2E) (Figure 1C). In A2E, the two retinoids are fused via a charged pyridinium ring (Parish et al., 1998). When photoreceptor discs are shed for recycling, they are phagocytosed by the underlying retinal pigment epithelium (RPE), a layer of cells that also contain melanosomes. Bis-retinoids cannot be digested by lysosomal enzymes in the RPE’s phagosomes, resulting in membrane-limited melanolipofuscin granules in the RPE of aged human eyes, Stargardt disease patients, and Stargardt model mice (Figure 1D) (reviewed in (Lyu et al., 2023)). The accumulation of melanolipofuscin granules, sometimes termed “melanolysosomes,” reflects onset and progression of AMD more closely than lipofuscin accumulation alone (Feeney-Burns et al., 1984).
5 Quantum chemistry in the eye
Albino Stargardt mice accumulate lipofuscin faster and develop retinal degeneration earlier than pigmented strains, suggesting that melanin is beneficial (Schraermeyer and Heimann, 1999; Wu et al., 2010; Radu et al., 2011; Taubitz et al., 2018). The known decrease in RPE melanin with age (Bonilha, 2008) would then diminish protection. This conclusion is supported by a gene therapy experiment injecting the gene for tyrosinase, the main synthetic enzyme for melanin, into the retina of albino mice. The treatment induced melanosomes and removed lipofuscin (Lyu et al., 2023).
Pharmacologically, the lipofuscin component can be degraded by intravitreal injection with generators of oxygen radicals such as superoxide (O2⋅-), peroxyl (OO⋅), and hydroperoxyl (HOO⋅), in both monkeys and Stargardt mice (Lyu et al., 2023). These treatments include: Remofuscin plus ambient or blue light (Fang et al., 2022); riboflavin (Schraermeyer et al., 2019); and Visudyne plus red light (Schraermeyer et al., 2019). Blue light plus lipofuscin generates superoxide and degrades lipofuscin, but is toxic (Gaillard et al., 2004; Ueda et al., 2016). The enzymes horseradish peroxidase and manganese peroxidase also degrade lipofuscin (Wu et al., 2011; Moody et al., 2018). Remofuscin rescues existing retinal degeneration in the Stargardt mouse model. Melanolipofuscin granules are also degraded by the nitric oxide (⋅NO) generators SIN-1 (Figures 2A,B) and isosorbide dinitrate (Lyu et al., 2023). These results are conventionally taken to indicate a direct free radical pathway for bis-retinoid degradation during phagocytosis of the disc outer segments, inasmuch as RPE phagocytosis generates oxygen radicals, hydrogen peroxide (H2O2), and the resulting hazardous Fenton-type reaction. However, these mechanisms should be operative in albinos lacking melanin; perhaps more efficiently because ocular melanin scavenges radicals (Sarna, 1992). An alternative comes from the fact that this same list of radicals and enzymes have another activity—chemiexcitation of melanin.
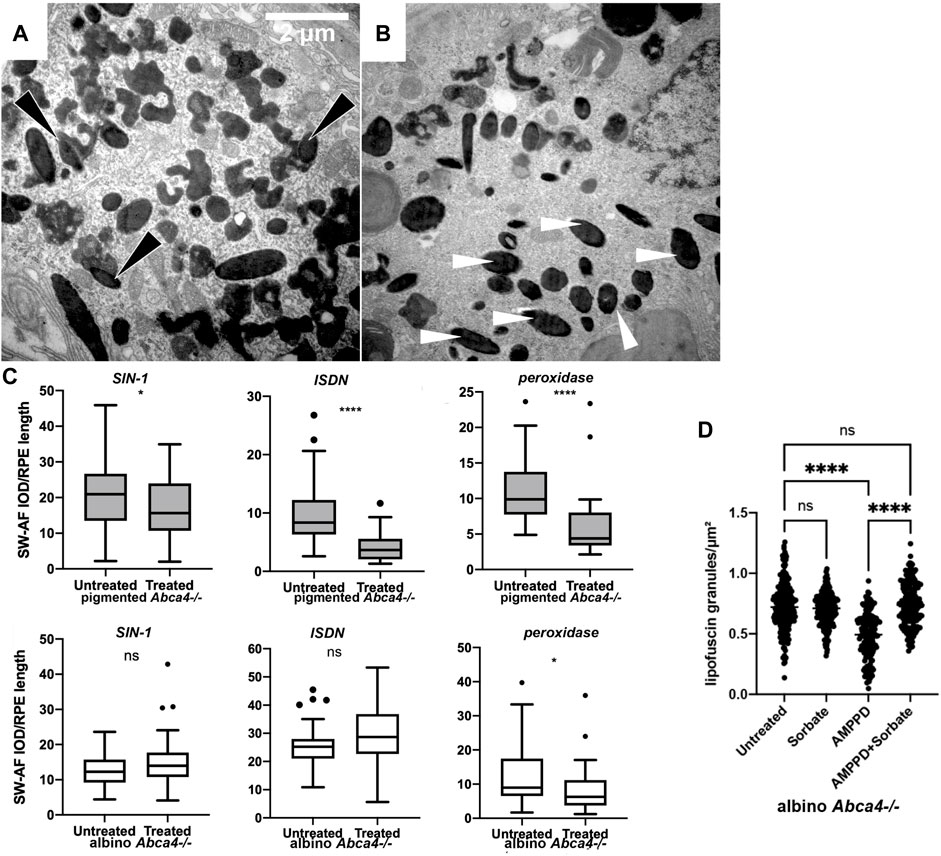
Figure 2. Removal of retinal lipofuscin by chemiexcitation and melanin. (A,B) Injecting a generator of superoxide plus nitric oxide stimulates lipofuscin removal from the RPE of pigmented Abca4−/− Stargardt model mice but not in albino. SIN-1, a generator of superoxide O2⋅- and ⋅NO, reduced the amount of lipofuscin present in the RPE cells of pigmented Abca4−/− mice 48 h later. (A) Pigmented mouse, untreated. Melanin granules (dark opacity) fused with lipofuscin (light opacity), forming a melanolipofuscin granule (black arrowheads). (B) Pigmented mouse, contralateral eye treated with SIN-1. Melanin granules occur more frequently as single melanosomes (white arrowheads) that are not associated with lipofuscin. (C) Melanin-dependent lipofuscin removal by a generator of superoxide, nitric oxide, and peroxynitrite (SIN-1); by a generator of nitric oxide alone (ISDN); or by peroxidase. Murine eye flatmounts were treated with the indicated reagent and lipofuscin autofluorescence was scored. These reagents can each initiate chemiexcitation in tubo (Adam and Cilento, 1982; Premi et al., 2015). Y axis quantifies lipofuscin autofluorescence per RPE length. (D) Treating murine eye flatmounts with the dioxetane-containing molecule AMPPD, which generates excited electronic states autonomously, removes lipofuscin granules from the RPE even in the absence of melanin. Autofluorescent lipofuscin granules in the retinal pigment epithelium were reduced by half in 2 days. This reduction was inhibited by the triplet-state quencher sorbate.
The possibility that lipofuscin removal was due to melanin chemiexcitation—biology’s use of quantum medicine—was tested experimentally in three ways (Lyu et al., 2023). First, in albino mice the radical-generators and peroxidase were ineffective (Figure 2C). Second, a reagent that undergoes chemiexcitation on its own, without melanin, was effective (Figure 2D). That reagent, AMPPD (3-(2′-spiroadamantyl)-4-methoxy-4-(3″-phosphoryloxy)-phenyl-1,2-dioxetane), contains a dioxetane stabilized by a protecting group that prevents the molecular twisting (Bronstein et al., 1989). This protecting group is removed by alkaline phosphatase, an enzyme present in the RPE (Korte et al., 1991). Third, AMPPD’s removal of lipofuscin was blocked by a specific quencher of excited triplet states, sorbate (Velosa et al., 2007) (Figure 2D). These results suggest that the combination of these events could be the eye’s normal lipofuscin-disposal mechanism in youth. As a melanin-suicide mechanism, it is consistent with the known depletion of retinal melanin with age.
A direct in vivo test of the role of chemiexcitation in youthful AMD prevention or drug-induced remediation is to use triplet state quenchers that cross the blood-retina barrier. Lipofuscin would accumulate earlier and faster. Several biomolecules that are effective triplet state quenchers (Angelé-Martínez et al., 2022) do cross the blood-brain barrier; these or their analogs include resveratrol, lutein, vitamin E, and rapamycin (which contains a sorbate-like moiety) (Meng et al., 2021).
6 Converting physics to chemistry
A triplet-excited molecule eventually dissipates its energy in one of several ways (Brash and Goncalves, 2023). Light, heat, and cis-trans isomerization are relatively autonomous. Because an electronically excited molecule resembles a diradical, it can instead initiate chemistry: split between two Cs to make two radicals, add to a C=C double bond, or abstract an H from another molecule. In a purely physical reaction, it can transfer its energy to a ground-state singlet acceptor, rendering the acceptor a triplet; the acceptor now has the same options just described. Alternatively, the triplet-excited molecule can transfer its energy to ambient O2, exciting it to very reactive singlet oxygen. Singlet oxygen can initiate chemistry, forming a peroxide, peroxyl radical, or dioxetane (Di Mascio et al., 2019). Which of these pathways destroys the lipofuscin?
In the case of a bis-retinoid like A2E, these options provide a limited menu of potential routes depending on melanin. For clarity, we will use the * convention for each excited state and the superscript 1 or 3 to denote a singlet or triplet state. The permanent positive charge on the A2E pyridinium ring will be omitted for simplicity.
6.1 Melanin chemiexcitation
The energy of chemiexcited 3melanin* is approximately 70 kcal/mol (Premi et al., 2015), so it could transfer its energy to molecules having lower energy levels. A2E has a singlet state 1A2E* of approximately 66 kcal/mol, excitable by even blue light (Lamb et al., 2001). A2E undergoes negligible intersystem crossing to the triplet state when photoexcited (Cantrell et al., 2001), but it does accept triplet energy from a triplet-energy donor having energy higher than its own of <33 kcal/mol (Ragauskaite et al., 2001). Therefore chemiexciting melanin can access A2E’s triplet state whereas photoexciting A2E cannot. Subsequent events can be:
• Energy in the excited A2E reversing the bis-linkage or otherwise cleaving A2E. Laboratory synthesis of bis-retinoids involves two sigmatropic rearrangements (Parish et al., 1998), which can be mediated by electron excitation and are sometimes reversible. The pyridinium ring is fairly stable, yet violet light is known to degrade A2E and degradation is blocked by vitamin E (Ueda et al., 2016), which is both a radical scavenger and a triplet-state quencher (Angelé-Martínez et al., 2022).
• Energy in the excited A2E isomerizing one of its double bonds, perhaps making it degradable or facilitating macrophage engulfment.
• 1A2E* abstracting an electron or H from a ground state A2E, making A2E peroxide or peroxyl radical, respectively:
R indicates the rest of the molecule. Steps 1.2c and 1.2d initiate chain reactions via R· and the hydroxyl radical. Because 3melanin* can abstract an electron from A2E, it could drive reaction 1.2a directly. If its hydroquinones donate H, 3melanin* would also drive reaction 2. The initial abstractions require electronic excitation because ground-state A2E does not readily donate electrons (its reduction potential is −700 to −900 mV) nor does it react with O2⋅-, hydroperoxide, or CO3−⋅ (Broniec et al., 2005).
The peroxide or peroxyl radical would then add a single O to one or more double bonds in A2E, particularly adjacent to the β-ionone ring (Gaillard et al., 2004). Cyclization of the peroxyl radical would create a dioxetane on a conjugated double bond, also leading to cleavage (Ukai et al., 1994).
• 3Melanin* can instead transfer its UVB-like energy to ambient O2, creating singlet oxygen, 1O2* (Szewczyk et al., 2016). Photoexcited A2E does not, due to its inefficient crossing to the triplet state (Kanofsky et al., 2003). 1O2* can diffuse appreciable distances and cross membranes (Skovsen et al., 2005), which would allow it to reach lipofuscin. It can add to a conjugated double bond to create a new dioxetane and triplet carbonyl (Ukai et al., 1994), potentially propagating the process spatially in a 1O2*–dioxetane chain reaction. Upon reaching a target:
The 1O2* can split polyenes (such as in A2E), leaving a single O on each product (Stratton et al., 1993; Ukai et al., 1994). There are preferred cleavage sites. Depending on the cleavage site, this split can leave highly reactive and toxic aldehydes or less toxic ketones (Ukai et al., 1994; Ueda et al., 2016).
1O2* can isomerize the A2E polyene (Foote et al., 1970), perhaps making it degradable or facilitating macrophage engulfment.
6.2 Melanin as a scaffold
Melanin contains stable radicals and binds metals, riboflavin, and electron-donating amine drugs (Kozik et al., 1990; Meredith and Sarna, 2006; Karlsson and Lindquist, 2016)}. Therefore:
• A melanolipofuscin granule would juxtapose the reactants in the radical reactions above. For example, melanin binds A2E (Dontsov et al., 2013) and binds tightly to another pyridinium-containing molecule that induces Parkinson’s (D'Amato et al., 1986).
• Unexcited melanin could enhance those radical reactions by its bound transition metals serving as the electron donor for making A2E⋅−, or its semiquinones serving as H donors to make A2EH⋅.
• Melanin’s bound metals can participate in Fenton-like electron transfer reactions.
• When riboflavin is excited by violet or blue light, the O2⋅- and 1O2* it generates (Jung et al., 2007; Cardoso et al., 2012) would be adjacent to the lipofuscin target. Indeed, melanin itself generates O2⋅- upon light exposure (Meredith and Sarna, 2006).
7 Discussion—clinical implications
One route to prevention would be to restore melanin by intravitreal injections of melanin-nanoparticles (Kaufmann and Han, 2024), supplanting the current practice of intravitreal injections to prevent blood vessel proliferation in advanced AMD. Yet the eye’s immune privilege means that any infection during injection is serious. A permanent approach would be a single introduction of the tyrosinase gene to re-activate melanin synthesis (Lyu et al., 2023). An alternative circumventing the need for melanin would be a drug directly generating the high-energy excited states without melanin, in analogy to AMPPD. The ideal drug would traverse the blood-retina barrier, obviating intravitreal injection, and minimize triplet-state side-reactions by targeting melanolipofuscin granules. That drug would be the first example of quantum biology entering the clinic.
Author contributions
DB: Conceptualization, Funding acquisition, Writing–original draft, Writing–review and editing. EG: Funding acquisition, Writing–original draft, Writing–review and editing.
Funding
The author(s) declare that financial support was received for the research, authorship, and/or publication of this article. The authors’ research and the writing of this mini-review were supported by grants from the National Institute of Arthritis and Musculoskeletal and Skin Diseases (5R01AR070851 to DB) and the National Science Foundation (#1726931 to EG).
Conflict of interest
The authors declare that the research was conducted in the absence of any commercial or financial relationships that could be construed as a potential conflict of interest.
Publisher’s note
All claims expressed in this article are solely those of the authors and do not necessarily represent those of their affiliated organizations, or those of the publisher, the editors and the reviewers. Any product that may be evaluated in this article, or claim that may be made by its manufacturer, is not guaranteed or endorsed by the publisher.
Abbreviations
AMD, age-related macular degeneration; AMPPD, (3-(2′-spiroadamantyl)-4-methoxy-4-(3″-phosphoryloxy)-phenyl-1,2-dioxetane); A2E, (2-[2,6-dimethyl-8-(2,6,6-trimethyl-1-cyclohexen-1-yl)-1E,3E,5E, 7Eoctatetraenyl]-1- (2-hydroxyethyl)-4-[4-methyl-6 (2,6,6-trimethyl-1-cyclohexen-1-yl)-1E,3E,5E,7E- hexatrienyl]-pyridinium); CPD, cyclobutane pyrimidine dimer; dCPD, dark cyclobutane pyrimidine dimer; HOMO, highest occupied molecular orbital; LUMO, lowest unoccupied molecular orbital; RPE, retinal pigment epithelium.
References
Adam, W., and Cilento, G. (1982). Chemical and biological generation of excited states. New York: Academic Press.
Angelé-Martínez, C., Goncalves, L. C. P., Premi, S., Augusto, F. A., Palmatier, M. A., Amar, S. K., et al. (2022). Triplet-energy quenching functions of antioxidant molecules. Antioxidants 11 (2), 357–376. doi:10.3390/antiox11020357
Azmitia, E. C. (2010). “Evolution of serotonin: sunlight to suicide,” in Handbook of behavioral neurobiology of serotonin. Editors C. Muller, and B. Jacobs (Amsterdam: Elsevier), 3–22.
Blois, M. S. (1971). “Physical studies of the melanins,” in Biology of normal and abnormal melanocytes. Editors T. Kawamura, T. B. Fitzpatrick, and M. Seiji (Baltimore: University Park Press), 125–139.
Bonilha, V. L. (2008). Age and disease-related structural changes in the retinal pigment epithelium. Clin. Ophthalmol. 2 (2), 413–424. doi:10.2147/opth.s2151
Brash, D. E. (2015). UV signature mutations. Photochem Photobiol. 91 (1), 15–26. doi:10.1111/php.12377
Brash, D. E., and Goncalves, L. C. P. (2023). Chemiexcitation: mammalian photochemistry in the dark. Photochem. Photobiol. 99, 251–276. doi:10.1111/PHP.13781
Brash, D. E., Goncalves, L. C. P., and Bechara, E. J. H.Group, E.-S. M. W. (2018). Chemiexcitation and its implications for disease. Trends Mol. Med. 24 (6), 527–541. doi:10.1016/j.molmed.2018.04.004
Broniec, A., Pawlak, A., Sarna, T., Wielgus, A., Roberts, J. E., Land, E. J., et al. (2005). Spectroscopic properties and reactivity of free radical forms of A2E. Free Radic. Biol. Med. 38 (8), 1037–1046. doi:10.1016/j.freeradbiomed.2004.12.023
Bronstein, I., Edwards, B., and Voyta, J. C. (1989). 1,2-Dioxetanes: novel chemiluminescent enzyme substrates. Applications to immunoassays. J. Bioluminesc Chemiluminesc 4, 99–111. doi:10.1002/bio.1170040116
Cantrell, A., McGarvey, D. J., Roberts, J., Sarna, T., and Truscott, T. G. (2001). Photochemical studies of A2-E. J. Photochem Photobiol. B 64 (2-3), 162–165. doi:10.1016/s1011-1344(01)00224-x
Cardoso, D. R., Libardi, S. H., and Skibsted, L. H. (2012). Riboflavin as a photosensitizer. Effects on human health and food quality. Food & Funct. 3 (5), 487–502. doi:10.1039/c2fo10246c
Chedekel, M. R., Smith, S. K., Post, P. W., Pokora, A., and Vessell, D. L. (1978). Photodestruction of pheomelanin: role of oxygen. Proc. Natl. Acad. Sci. U.S.A. 75, 5395–5399. doi:10.1073/pnas.75.11.5395
Crippa, P. R., and Viappiani, C. (1990). Photoacoustic studies of non-radiative relaxation of excited states in melanin. Eur. Biophys. J. 17 (6), 299–305. doi:10.1007/BF00258378
D'Amato, R. J., Lipman, Z. P., and Snyder, S. H. (1986). Selectivity of the parkinsonian neurotoxin MPTP: toxic metabolite MPP+ binds to neuromelanin. Science 231 (4741), 987–989. doi:10.1126/science.3080808
Delinasios, G. J., Karbaschi, M., Cooke, M. S., and Young, A. R. (2018). Vitamin E inhibits the UVAI induction of "light" and "dark" cyclobutane pyrimidine dimers, and oxidatively generated DNA damage, in keratinocytes. Sci. Rep. 8 (1), 423. doi:10.1038/s41598-017-18924-4
Di Mascio, P., Martinez, G. R., Miyamoto, S., Ronsein, G. E., Medeiros, M. H. G., and Cadet, J. (2019). Singlet molecular oxygen reactions with nucleic acids, lipids, and proteins. Chem. Rev. 119 (3), 2043–2086. doi:10.1021/acs.chemrev.8b00554
Dontsov, A. E., Koromyslova, A. D., and Sakina, N. L. (2013). Lipofuscin component A2E does not reduce antioxidant activity of DOPA-melanin. Bull. Exp. Biol. Med. 154 (5), 624–627. doi:10.1007/s10517-013-2015-6
Epe, B., Müller, E., Adam, W., and Saha-Möller, C. R. (1992). Photochemical DNA modifications induced by 1, 2-dioxetanes. Chemico-biological Interact. 85 (2-3), 265–281. doi:10.1016/0009-2797(92)90067-u
Fajuyigbe, D., Douki, T., van Dijk, A., Sarkany, R. P. E., and Young, A. R. (2021). Dark cyclobutane pyrimidine dimers are formed in the epidermis of Fitzpatrick skin types I/II and VI in vivo after exposure to solar-simulated radiation. Pigment. Cell Melanoma Res. 34 (3), 575–584. doi:10.1111/pcmr.12956
Fang, Y., Taubitz, T., Tschulakow, A. V., Heiduschka, P., Szewczyk, G., Burnet, M., et al. (2022). Removal of RPE lipofuscin results in rescue from retinal degeneration in a mouse model of advanced Stargardt disease: role of reactive oxygen species. Free Radic. Biol. Med. 182, 132–149. doi:10.1016/j.freeradbiomed.2022.02.025
Farahani, P., Roca-Sanjuán, D., Felipe Zapata, F., and Lindh, R. (2013). Revisiting the nonadiabatic process in 1,2-dioxetane. J. Chem. Theory Comput. 9, 5404–5411. doi:10.1021/ct4007844
Feeney-Burns, L., Hilderbrand, E. S., and Eldridge, S. (1984). Aging human RPE: morphometric analysis of macular, equatorial, and peripheral cells. Invest Ophthalmol. Vis. Sci. 25 (2), 195–200.
Felix, C. C., Hyde, J. S., and Sealy, R. C. (1979). Photoreactions of melanin: a new transient species and evidence for triplet state involvement. Biochem. Biophys. Res. Commun. 88 (2), 456–461. doi:10.1016/0006-291x(79)92070-9
Foote, C. S., Chang, Y. C., and Denny, R. W. (1970). Chemistry of singlet oxygen. XI. Cis-trans isomerization of carotenoids by single oxygen and a probable quenching mechanism. J. Am. Chem. Soc. 92 (17), 5218–5219. doi:10.1021/ja00720a037
Gaillard, E. R., Avalle, L. B., Keller, L. M., Wang, Z., Reszka, K. J., and Dillon, J. P. (2004). A mechanistic study of the photooxidation of A2E, a component of human retinal lipofuscin. Exp. Eye Res. 79 (3), 313–319. doi:10.1016/j.exer.2004.05.005
Gonçalves, L. C. P., Angelé-Martinez, C., Premi, S., Palmatier, M. A., Prado, F. M., Di Mascio, P., et al. (2023). Chemiexcited neurotransmitters and hormones create DNA photoproducts in the dark. ACS Chem. Biol. 18, 484–493. doi:10.1021/acschembio.2c00787
Hong, L., and Simon, J. D. (2007). Current understanding of the binding sites, capacity, affinity, and biological significance of metals in melanin. J. Phys. Chem. B 111 (28), 7938–7947. doi:10.1021/jp071439h
Jung, M. Y., Oh, Y. S., Kim, D. K., Kim, H. J., and Min, D. B. (2007). Photoinduced generation of 2,3-butanedione from riboflavin. J. Agric. Food Chem. 55 (1), 170–174. doi:10.1021/jf061999y
Kanofsky, J. R., Sima, P. D., and Richter, C. (2003). Singlet-oxygen generation from A2E. Photochem Photobiol. 77 (3), 235–242. doi:10.1562/0031-8655(2003)077<0235:sogfa>2.0.co;2
Karlsson, O., and Lindquist, N. G. (2016). Melanin and neuromelanin binding of drugs and chemicals: toxicological implications. Arch. Toxicol. 90 (8), 1883–1891. doi:10.1007/s00204-016-1757-0
Kaufmann, M., and Han, Z. (2024). RPE melanin and its influence on the progression of AMD. Ageing Res. Rev. 99, 102358. doi:10.1016/j.arr.2024.102358
Kohl, F. R., Grieco, C., and Kohler, B. (2019). Ultrafast spectral hole burning reveals the distinct chromophores in eumelanin and their common photoresponse. Chem. Sci. 11 (5), 1248–1259. doi:10.1039/c9sc04527a
Korte, G. E., Rappa, E., and Andracchi, S. (1991). Localization of alkaline phosphatase on basolateral plasma membrane of normal and regenerating retinal pigment epithelium. A cytochemical study in rabbits. Invest. Ophthalmol. Vis. Sci. 32, 3187–3197.
Kozik, A., Korytowski, W., Sarna, T., and Bloom, A. S. (1990). Interactions of flavins with melanin. Biophys. Chem. 38 (1-2), 39–48. doi:10.1016/0301-4622(90)80038-9
Lamb, L. E., Ye, T., Haralampus-Grynaviski, N. M., Williams, T. R., Pawlak, A., Sarna, T., et al. (2001). Primary photophysical properties of A2E in solution. J. Phys. Chem. B 105, 11507–11512. doi:10.1021/jp0123177
Lawrence, K. P., Douki, T., Sarkany, R. P. E., Acker, S., Herzog, B., and Young, A. R. (2018). The UV/visible radiation boundary region (385-405 nm) damages skin cells and induces "dark" cyclobutane pyrimidine dimers in human skin in vivo. Sci. Rep. 8 (1), 12722. doi:10.1038/s41598-018-30738-6
Lyu, Y., Tschulakow, A. V., Wang, K., Brash, D. E., and Schraermeyer, U. (2023). Chemiexcitation and melanin in photoreceptor disc turnover and prevention of macular degeneration. Proc. Natl. Acad. Sci. U. S. A. 120 (20), e2216935120. doi:10.1073/pnas.2216935120
Meng, F., Xi, Y., Huang, J., and Ayers, P. W. (2021). A curated diverse molecular database of blood-brain barrier permeability with chemical descriptors. Sci. Data 8 (1), 289. doi:10.1038/s41597-021-01069-5
Meredith, P., and Sarna, T. (2006). The physical and chemical properties of eumelanin. Pigment. Cell Res. 19 (6), 572–594. PCR345 [pii]. doi:10.1111/j.1600-0749.2006.00345.x
Moody, K. J., Tinklepaugh, J., Obert, E., Grohn, K., DeRosa, J. R., Lumen, E., et al. (2018). Recombinant manganese peroxidase reduces A2E burden in age-related and Stargardt's macular degeneration models. Rejuvenation Res. 21 (6), 560–571. doi:10.1089/rej.2018.2146
Mostert, A. B., Rienecker, S. B., Noble, C., Hanson, G. R., and Meredith, P. (2018). The photoreactive free radical in eumelanin. Sci. Adv. 4 (3), eaaq1293. doi:10.1126/sciadv.aaq1293
Najder-Kozdrowska, L., Pilawa, B., Buszman, E., Więckowski, A. B., Şwiătkowska, L., Wrzeşniok, D., et al. (2010). Triplet states in DOPA-melanin and in its complexes with kanamycin and copper Cu(II) ions. Acta Phys. Pol. A 118, 613–618. doi:10.12693/aphyspola.118.613
Parish, C. A., Hashimoto, M., Nakanishi, K., Dillon, J., and Sparrow, J. (1998). Isolation and one-step preparation of A2E and iso-A2E, fluorophores from human retinal pigment epithelium. Proc. Natl. Acad. Sci. U. S. A. 95 (25), 14609–14613. doi:10.1073/pnas.95.25.14609
Premi, S., Wallisch, S., Mano, C. M., Weiner, A. B., Bacchiocchi, A., Wakamatsu, K., et al. (2015). Chemiexcitation of melanin derivatives induces DNA photoproducts long after UV exposure. Science 347 (6224), 842–847. doi:10.1126/science.1256022
Pullman, A., and Pullman, B. (1961). The band structure of melanins. Biochim. Biophys. Acta 54, 384–385. doi:10.1016/0006-3002(61)90389-4
Radu, R. A., Hu, J., Yuan, Q., Welch, D. L., Makshanoff, J., Lloyd, M., et al. (2011). Complement system dysregulation and inflammation in the retinal pigment epithelium of a mouse model for Stargardt macular degeneration. J. Biol. Chem. 286 (21), 18593–18601. doi:10.1074/jbc.M110.191866
Ragauskaite, L., Heckathorn, R. C., and Gaillard, E. R. (2001). Environmental effects on the photochemistry of A2-E, a component of human retinal lipofuscin¶. Photochem Photobiol. 74 (3), 483–488. doi:10.1562/0031-8655(2001)074<0483:eeotpo>2.0.co;2
Sarna, T. (1992). New trends in photobiology. J. Photochem Photobiol. B 12 (3), 215–258. doi:10.1016/1011-1344(92)85027-r
Schraermeyer, U., Fang, Y., Taubitz, T., and Tschulakow, A. (2019a). Degradation of lipofuscin in Stargardt mice can be enhanced by the superoxide generator riboflavin - a hypothesis for melanolipofuscin formation. Invest. Ophthalmol. Vis. Sci. 60, 1912.
Schraermeyer, U., and Heimann, K. (1999). Current understanding on the role of retinal pigment epithelium and its pigmentation. Pigment. Cell Res. 12 (4), 219–236. doi:10.1111/j.1600-0749.1999.tb00755.x
Schraermeyer, U. B., Senn-Bilfinger, J., Sturm, E., and Hanauer, G. (2019b). Methods for the determination of compounds or compositions for the treatment of lipofuscin related diseases and compounds or compositions. Germany patent application.
Schreier, W. J., Gilch, P., and Zinth, W. (2015). Early events of DNA photodamage. Annu. Rev. Phys. Chem. 66 (1), 497–519. doi:10.1146/annurev-physchem-040214-121821
Simon, J. D., and Peles, D. N. (2010). The red and the black. Acc. Chem. Res. 43 (11), 1452–1460. doi:10.1021/ar100079y
Skovsen, E., Snyder, J. W., Lambert, J. D. C., and Ogilby, P. R. (2005). Lifetime and diffusion of singlet oxygen in a cell. J. Phys. Chem. B Lett. 109, 8570–8573. doi:10.1021/jp051163i
Stratton, S. P., Schaefer, W. H., and Liebler, D. C. (1993). Isolation and identification of singlet oxygen oxidation products of.beta.-carotene. Chem. Res. Toxicol. 6 (4), 542–547. doi:10.1021/tx00034a024
Szewczyk, G., Zadlo, A., Sarna, M., Ito, S., Wakamatsu, K., and Sarna, T. (2016). Aerobic photoreactivity of synthetic eumelanins and pheomelanins: generation of singlet oxygen and superoxide anion. Pigment. Cell Melanoma Res. 29 (6), 669–678. doi:10.1111/pcmr.12514
Taubitz, T., Tschulakow, A. V., Tikhonovich, M., Illing, B., Fang, Y., Biesemeier, A., et al. (2018). Ultrastructural alterations in the retinal pigment epithelium and photoreceptors of a Stargardt patient and three Stargardt mouse models: indication for the central role of RPE melanin in oxidative stress. PeerJ 6, e5215. doi:10.7717/peerj.5215
Turro, N. J., and Kraeutler, B. (1980). Magnetic field and magnetic isotope effects in organic photochemical reactions. A novel probe of reaction mechanisms and a method for enrichment of magnetic isotopes. Acc. Che. Res. 13, 369–377. doi:10.1021/ar50154a005
Ueda, K., Zhao, J., Kim, H. J., and Sparrow, J. R. (2016). Photodegradation of retinal bisretinoids in mouse models and implications for macular degeneration. Proc. Natl. Acad. Sci. U. S. A. 113 (25), 6904–6909. doi:10.1073/pnas.1524774113
Ukai, N., Lu, Y., Etoh, H., Yagi, A., Ina, K., Oshima, S., et al. (1994). Photosensitized oxygenation of lycopene. Biosci. Biotech. Biochem. 58, 1718–1719. doi:10.1271/bbb.58.1718
Velosa, A. C., Baader, W. J., Stevani, C. V., Mano, C. M., and Bechara, E. J. H. (2007). 1,3-Diene probes for detection of triplet carbonyls in biological systems. Chem. Res. Toxicol. 20 (8), 1162–1169. doi:10.1021/tx700074n
Williams, G. C. (1957). Pleiotropy, natural selection, and the evolution of senescence. Evolution 11, 398–411. doi:10.2307/2406060
Wilson, T., and Hastings, J. W. (2013). Bioluminescence: living lights, lights for living. Cambridge, MA: Harvard Univ. Press.
Woodward, J. R. (2002). Radical pairs in solution. Progr. React. Kinet. Mech. 27, 165–207. doi:10.3184/007967402103165388
Wu, L., Nagasaki, T., and Sparrow, J. R. (2010). Photoreceptor cell degeneration in Abcr (-/-) mice. Adv. Exp. Med. Biol. 664, 533–539. doi:10.1007/978-1-4419-1399-9_61
Keywords: chemiexcitation, dioxetane, triplet state, photoreceptor, retinal pigment epithelium, melanin, lipofuscin, macular degeneration
Citation: Brash DE and Gaillard ER (2024) Chemiexcitation in preventing macular degeneration. Front. Photonics 5:1451857. doi: 10.3389/fphot.2024.1451857
Received: 19 June 2024; Accepted: 16 July 2024;
Published: 21 August 2024.
Edited by:
Marcelo Victor Pires de Sousa, D’Or Institute for Research and Education (IDOR), BrazilReviewed by:
Yanchun Wei, Huaiyin Institute of Technology, ChinaCopyright © 2024 Brash and Gaillard. This is an open-access article distributed under the terms of the Creative Commons Attribution License (CC BY). The use, distribution or reproduction in other forums is permitted, provided the original author(s) and the copyright owner(s) are credited and that the original publication in this journal is cited, in accordance with accepted academic practice. No use, distribution or reproduction is permitted which does not comply with these terms.
*Correspondence: Douglas E. Brash, douglas.brash@yale.edu