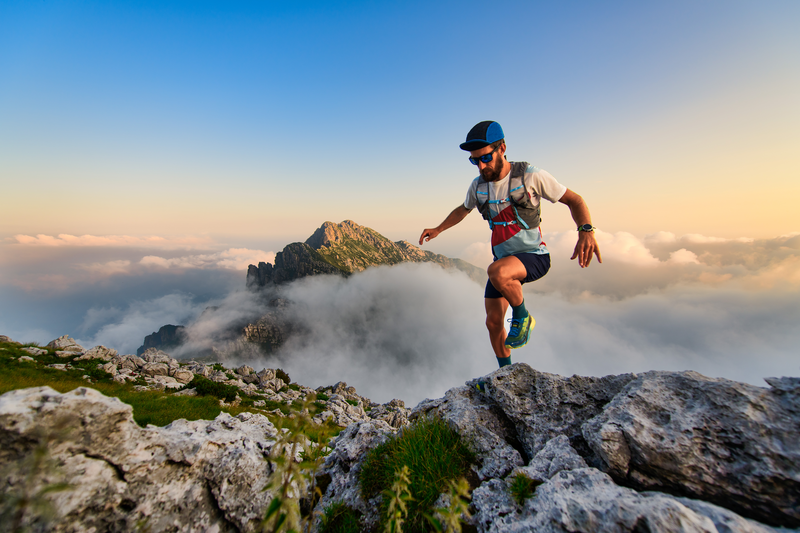
94% of researchers rate our articles as excellent or good
Learn more about the work of our research integrity team to safeguard the quality of each article we publish.
Find out more
MINI REVIEW article
Front. Photonics , 19 June 2024
Sec. Biophotonics
Volume 5 - 2024 | https://doi.org/10.3389/fphot.2024.1423280
In injury, fibroblasts are key for disintegrating the fibrin clot, secreting collagen proteins, and constructing extracellular matrix Altered fibroblast function is among the common characteristics of delayed wound healing and development of chronic wounds. Thus, treatment methods for effective wound healing require agents that affect the complexed wound milieu, including fibroblast cells. Diabetes mellitus (DM) is a common cause of impaired fibroblast cell function, delayed wound healing, development of chronic ulcers, and limb amputation. Photobiomodulation (PBM) promotes fibroblast cell proliferation, migration, release of growth factors and wound healing. Yet, its use is limited largely due to an incomplete understanding of its underlying mechanisms at the molecular and cellular level. In this review, we summarise the involvement of fibroblast cells in wound healing, and the effect of PBM on fibroblast cell function and diabetic wound healing. PubMed, Google Scholar, and Research Gate were used to find pertinent academic articles.
The global morbidity rate of diabetes mellitus (DM) is increasing, and by 2030 it is estimated to increase from approximately 5%–8% (Liao et al., 2022). Several complications develop as a result of DM, accompanied with high treatment costs, disability, and lowered life expectancy of the affected individual. Diabetic foot ulcers (DFUs) are a critical marker for high mortality in diabetic patients, and is characterised by infection, persistent wound formation, and destruction of the deep tissue. The development of diabetic ulcers is largely due to neuropathy and peripheral arterial disease, and the risk of developing chronic wounds in diabetic patients is reported to have increased to approximately 25% (Jais, 2023). Literature elucidates that in DM, dermal fibroblast activity is reduced, and is closely related to the reduced wound healing process (Evangelatov et al., 2022). Treatment for diabetic ulcers is a challenge, frequently resulting in minor or major limb amputation which increases the mortality rate within 5 years to as high as 39%–68% (Petersen et al., 2020; Liao et al., 2022; Liu et al., 2022; Jais, 2023).
The notion that dermal fibroblasts comprise numerous separate subpopulations is a significant development in the field of skin pathophysiology and provides a fresh viewpoint that could lead to the development of innovative wound remedies (Des Jardins-Park et al., 2018). This review discusses the involvement of fibroblast cells in wound healing, and PBM as a treatment technique for fibroblast cell regeneration and survival in diabetic wounds. A search was conducted online using terms like “diabetic wound healing,” “fibroblast cell,” “fibroblast cell in wound healing,” “diabetes mellitus,” “effect of photobiomodulation,” and “laser therapy in diabetic wound healing” on PubMed, Google Scholar, and Research Gate. The heterogeneity of healthy fibroblast cells is well explained elsewhere (Liu et al., 2022).
During the skin wound healing process, the granulation tissue is basically composed of ECM, inflammatory cells, small blood vessels, and fibroblast and myofibroblast cells. Myofibroblast cells characteristically differ from normal fibroblasts due to their increased ability to synthesize large amounts of ECM proteins and tighten the wound. They express alpha-smooth muscle actin (α-SMA), an isoform of actin, and is found in the majority of connective tissues of healing wounds (Evangelatov et al., 2022). Fibroblasts are mesenchymal-derived cells, and are widely found in most tissues. This cell type express vimentin, but neither desmin nor α-SMA are expressed, and under normal circumstances, fibroblasts are essential for controlling the turnover of ECM. Fibroblasts are activated in injured tissue, and undergo differentiation into myofibroblasts (Figure 1) that shrink the wound and release ECM proteins to aid in the healing process (Li and Wang, 2011). Fibroblasts possess a variety of functions including creation of the basic tissue and organ framework, and are among the critical cell types for effective wound healing (Dick et al., 2023). Fibroblast cells are responsible for coordinating the entire wound healing process by producing a range of regulatory molecules and interacting with other cell populations involved in the healing mechanism. Injury provokes the release of cytokines, resulting from platelet degranulation, which sets off an inflammatory response. During this time, tumour necrosis factor-alpha (TNF-α), inducible nitric oxide synthase (iNOS), interleukin-1 (IL-1), interleukin-6 (IL-6), interleukin-12 (IL-12), and other pro-inflammatory mediators are further elevated, thereby promoting inflammation and fibroblast cell recruitment and activation (Francesca et al., 2022).
Although fibroblast cells are essential for an acute wounds’ ability to heal, they can also cause fibrosis and excessive scarring, and may prevent a lesion from healing as in the case of ulcerative and diabetic wounds (Samuel et al., 2023). Dysfunctional fibroblast cells is a critical factor in the development of chronic wounds, and abnormal deposition of ECM and collagen often cause permanent organ dysfunction. Research has demonstrated that fibroblasts from chronic wounds have aberrant characteristics, such as reduced proliferation, early senescence, and changed cytokine release patterns, in addition to aberrant MMP and tissue inhibitor of metalloproteinases (TIMP) activity (Wall et al., 2008; Li and Wang, 2011). Furthermore, it has been demonstrated that hyperglycaemia reduces migration, proliferation, and production of collagen, and enhances apoptosis of fibroblast cells (Xuan et al., 2014). Buranasin et al. (Buranasin et al., 2018) elucidated that hyperglycaemia affects fibroblast cell proliferation and migration, elevates inflammation, and hinders the wound healing process.
Delayed and postponed wound healing in DM is largely due to dysregulated differentiation of fibroblasts to myofibroblasts, disordered action of myofibroblasts, and insufficient deposition of ECM (Wan et al., 2021). Dysfunctional myofibroblasts can cause postponed or suspended wound repair process mostly due to failure in ECM reconstruction (Cialdai et al., 2022). One of the key pathogenic influences in diabetic complications is oxidative stress due to high concentrations of advanced glycation end products (AGEs). Increased oxidative stress is a vital mechanism that triggers cellular defects and accelerated tissue damage, mostly via the increased reactive oxygen species (ROS) and activated receptor for AGEs (RAGE). During the wound repair process, several pro-inflammatory cytokines and other signalling pathways, including transforming growth factor-beta (TGFβ)/Smad, similarly activate fibroblast cells and cellular fibrosis, and, increased expression of MMPs, TGF, Angiotensin II (Ang II), and TNF-α are associated with fibrous tissue remodelling (Zheng et al., 2019). High blood glucose activates the advancement of tissue fibrosis, and during diabetic wound healing is linked to reduced and malfunction of myofibroblasts (Deb et al., 2017). To demonstrate the effect of hyperglycaemia on fibroblasts, Haas et al. (Haas et al., 2021) noted a decreased expression of fibrosis-related genes and myofibroblasts in diabetic wounds. Lerman et al. (Lerman et al., 2003) found a significant reduction in fibroblast cell migration, transformed production of matrix metalloproteinase 9 (MMP-9), and a huge reduction in the production of vascular endothelial growth factor (VEGF) from diabetic mice. In addition, Zheng et al. (Zheng et al., 2019) showed that hyperglycaemia increases the expression of MMP-9, a protein that regulates the pathological remodelling processes involving prolonged inflammation and fibrosis, and intracellular domain of Notch receptor in fibroblasts. Recent studies have shown that hyperglycaemia reduces fibroblast proliferation and migration, and these findings clearly point out the failure of fibroblasts to hastily migrate into the wound milieu, reconstruct the ECM, and effectively promote angiogenesis in DM (Wall et al., 2008; Deng et al., 2021).
There are several therapeutic methods that classically focuses on offloading, maintaining a moist environment, and preventing infection and formation of biofilm. Growth factor and cell-based therapies have been used to target and improve the performance of fibroblasts (Berger et al., 2021). Recently, attempts have been made to transform the wound bed into an environment where maximum wound healing may be successfully obtained. In clinical practice, cells including fibroblasts have all been utilised to promote wound healing (You and Han, 2014). Also, nanotechnology-based therapies for chronic diabetic wounds have emerged, with numerous designed nanotechnologies exhibiting distinct qualities and diverse applications for wound repair beeing put forth (Hamdan et al., 2017). Furthermore, treatment approaches based on stem cells have been suggested, and their potential to enhance both the speed and calibre of wound healing as well as skin regeneration has been demonstrated (Nourian et al., 2019). However, these options are not satisfactory, and many patients still require amputation, with approximately 30% of patients with chronic diabetic wounds undergoing amputation (Deng et al., 2021). In recent years, the understanding of fibroblast cell behaviour in wound healing has led to discoveries of novel therapeutic techniques including photobiomodulation (PBM) (Des Jardins-Park et al., 2018).
Recently, the application of PBM therapy in medicine has gained a lot of interest. PBM induces a photobiomodulatory effect on tissue and cells to modulate cellular behaviour and enhance tissue repair. Previously known as low level laser/light therapy (LLLT), PBM is able to induce cellular proliferation, migration, and differentiation without causing tissue or cell’s temperature to rise. It is a non-invasive treatment technique effective for pain relief, reduction of inflammation, and enhanced wound healing (Dompe et al., 2020). It is believed that the mitochondria and gated ion channels for light and heat are the likely location for the primary effects of PBM, resulting in enhanced production of ATP and alteration of ROS, leading to downstream stimulation of transcription factors for cell proliferation, migration, and survival (Figure 2). This mechanism involves mitochondrial cytochrome c oxidase (CoX), a membrane protein having a binuclear copper (CuA) and a heme centre (a3-CuB). These proteins facilitate the transportation of electrons from CoX to oxygen in the electron transport chain. It is suggested that CoX represents the primary photoacceptor for the red and near infrared (NIR) light spectrum in mammalian cells (Farivar et al., 2014). The mechanisms of PBM and the mitochondrial redox signalling is well explained elsewhere (Hamblin, 2018). Different from pharmaceutical agents, PBM is described by parameters including wavelength, power output, energy density, frequency, and time of irradiation. It is believed that a reduced dosage may result in an insignificant cellular response, and an excessive dosage may completely impede positive cellular responses and induce apoptosis (Arndt–Schulz law). However, these cell responses have also been reported in some irradiated tumour cells, raising fear that PBM may enhance growth of tumours, as well as highlighting the importance of understanding the effects of PBM at the cell level for proper interpretation of treatment protocols in cancer patients (Tam et al., 2020).
Various transcription factors including nuclear factor kappa B (NF-κB) are regulated with the status of cellular redox to activate several intracellular signalling pathways for nucleic acid and protein synthesis, progression of cell cycle, and activation of enzymes. This process stimulates cell differentiation, increases cell proliferation and migration, and release of cytokines and growth factors. PBM modifies cellular transcription factors and gene expression resulting in various phenotypic cellular variations (Khalkhal et al., 2020). Different researchers have elucidated on the advantages of PBM in advancing human fibroblast cell proliferation and survival when used at appropriate parameters (Almeida-Lopes et al., 2001; Rani et al., 2005).
The equations should be inserted in editable format from the equation editor. Several studies have demonstrated the effect of PBM on fibroblast cell proliferation and migration in diabetic wound healing. In vitro, PBM has demonstrated a significant increase in the proliferation rate of human fibroblasts (Kreisler et al., 2002). When Khadra et al. (Khadra et al., 2005), used PBM using a diode laser at a fluence of 1.5 and 3 J/cm2 at a wavelength of 830 nm, they noted a significant improvement in human fibroblast cell attachment and proliferation on titanium discs. Using a diode laser at a wavelength of 670 nm, 780 nm, 692 nm, and 786 nm at a fluence of 2 J/cm2, Almeida-Lopes et al. (Almeida-Lopes et al., 2001) noted a significant increase in human fibroblast proliferation. Jere et al. (Jere et al., 2021) noted a significant increase in fibroblast cell migration when they used PBM at a wavelength of 660 nm and energy density of 5 J/cm2 on diabetic wounded and hypoxic diabetic wounded human fibroblasts. Furthermore, when Rajendran et al. (Rajendran et al., 2021) used PBM at a wavelength of 660 nm (fluence of 5 J/cm2, and power density of 11.2 mW/cm2), or 830 nm (fluence of 5 J/cm2, and power density of 10.3 mW/cm2), they observed enhanced migration in irradiated diabetic wounded fibroblast cells. Giannakopoulos et al. (Giannakopoulos et al., 2023) noted an increased activation of cellular migration and proliferation when they tried to investigate the role of red light at a wavelength of 661 nm on 3T3 fibroblast cell proliferation and migration. Similarly, Zhao et al. (Zhao et al., 2022) aimed to assess and relate the outcomes of 630 nm and 810 nm PBM on the proliferation of mouse fibroblast cells (L929) in wound healing in vitro and in vivo. They noted that both 630 nm and 810 nm irradiation significantly stimulated cell proliferation and migration. In another study, Oyebode and Houreld, (Oyebode and Houreld, 2022), noted a significantly increased human fibroblast cell proliferation and migration when they investigated the potential of PBM at 830 nm in accelerating cell proliferation and migration.
The differentiation of fibroblasts to myofibroblasts is regulated by different growth factors and the stiffness of the ECM (Sassoli et al., 2016). When Oyebode and Houreld, (Oyebode and Houreld, 2021), used diode lasers at a wavelength of 830 nm and 660 nm at a fluence of 5 J/cm2 on diabetic human fibroblast cells they reported successful initiation of fibroblast cell differentiation into myofibroblasts at a wavelength of 660 nm than 830 nm. However, they noted better results at a wavelength of 660 nm than at a wavelength of 830 nm. It should be noted that this was in vitro, and most studies make use of NIR as it has a deeper penetration depth than red light. Myofibroblasts demonstrate the competence to release ECM proteins and adopt a contractile phenotype to regulate tissue remodelling and wound contraction. Lau et al. (Lau et al., 2015) assessed the effects of near infrared (808 nm) PBM on diabetic wounds in rats at a fluence of 5 J/cm2 with power densities of 0.1 W/cm2, 0.2 W/cm2 and 0.3 W/cm2 and exposure time of 50 s, 25 s and 17 s, respectively. They noted that wound contraction was optimised with a power density of 0.1 W/cm2, and that PBM was able to enhance epithelialisation and collagen fibre synthesis, indicating effective transformation of fibroblasts to myofibroblasts. When Sassoli et al. (Sassoli et al., 2016) assessed NIH/3T3 fibroblast cells cultured in medium with reduced serum and additional TGF-β1 that were irradiated with a 635 nm diode laser at a fluence of 0.3 J/cm2, they noted that PBM inhibited TGF-β1-induced fibroblast differentiation to myofibroblast. They observed a significant reduction in the formation of stress fibres, α-SMA and expression of type-1 collagen, indicating that PBM at a lower fluence may negatively affected the differentiation of fibroblasts to myofibroblasts, critical for the reduction of fibrosis.
The wound healing process is largely regulated by mitogenic mediators including cytokines and growth factors that are produced by occupant and infiltrating cells within the wound milieu (Chen et al., 2014). The dermal fibroblast cells are critical for reconstructing the injured tissue, and produce essential growth factors and cytokine that contribute to the wound healing process. According to Rahbar et al. (Rahbar et al., 2020) dysfunctional fibroblast cell proliferation and communication within the dermis is the major cause of disrupted wound healing in DM. In DM the production of the mitogenic factors and their downstream cellular signalling that promote fibroblast cell activity is altered, further diminishing the wound healing process (Huang et al., 2018). Several studies have shown that PBM induces impaired diabetic fibroblasts for effective and enhanced diabetic wound healing. Rahbar et al. (Rahbar et al., 2020) demonstrated that PBM at a wavelength of 632.8 nm and a fluence of 0.5 J/cm2 stimulates the release of 7 growth factor/cytokines [eotaxin-3, brain-derived neurotrophic factor (BDNF), fibroblast growth factor 6 and 7 (FGF-6 and -7), fractalkine, fit-3ligand, and granulocyte chemotactic protein (GCP-2)] mainly involved in cell-to-cell communication and cell proliferation in diabetic fibroblast cells. Literature shows that PBM induces therapeutic benefits and increases the healing of diabetic wounds via the release of growth factors including FGFs (Kasowanjete et al., 2022). Karkada et al. (Karkada et al., 2023) showed that PBM stimulates the release of IL-6 and bFGF in human skin fibroblasts (HSFs) cultured in high glucose concentration medium. In addition, Khoo et al. (Khoo et al., 2014) observed a significant increase in the expression of FGF, platelet-derived growth factor (PDGF) and VEGF in irradiated diabetic fibroblast cells with a single dose of PBM at a wavelength of 810 nm and a fluence of 1 J/cm2. This suggests that PBM may play a critical role in diabetic wound healing by stimulating fibroblasts and the release of growth factors. Literature shows that PBM enhances wound healing, particularly diabetic wounds, and its effect in cellular function as a result of growth factor and cytokine production (Oyebode and Houreld, 2021). Research on the impact of repeated exposures to PBM on the responses of injured skin fibroblasts shows that appropriate energy density or fluence and exposure frequency can enhance the responses of injured fibroblasts and encourage cell migration and proliferation by promoting mitochondrial activity and preserving viability without adding to the stress or harm to the injured cells. The results show that the stimulatory effect is determined by the cumulative effect of lower dosages, while multiple exposure at larger concentrations produces an inhibitory effect that causes more damage (Rocha Júnior et al., 2007).
During the wound healing process, fibroblast cells play a key role in all the phases of wound healing, and crosstalk with other types of cells via the production of chemokines, growth factors, MMPs, and construction of ECM. In an effort to close and remodel the wounded tissue, fibroblasts differentiate into contractile myofibroblasts, which are responsible for increased production and construction of ECM, mainly aimed at reestablishing the normal function of the tissue after injury. Fibroblast dysfunction, therefore, is a distinctive attribute of impaired wound healing and the development of chronic non-healing wounds. For this reason, most therapeutic techniques target the regulation and progression of fibroblast cell performance. Diabetic wounds are problematic to heal, become chronic, and may necessitate limb amputation. However, the molecular and cellular modifications that lead to this deficiency is not completely known. PBM is a novel treatment technique that is able to induce and enhance tissue repair, and research shows that it compensates most impairments in diabetic fibroblast cells. Could this non-invasive and cheap treatment technique with limited side effects be effective in mitigating fibroblast dysfunction in diabetic wound healing? Due to controversy surrounding the use PBM, more research may be critical to understand its effect, at a cellular and molecular level, and the optimal dosage of PBM in diabetic wound healing.
SJ: Conceptualization, Writing–original draft, Writing–review and editing. NH: Writing–review and editing.
The author(s) declare that no financial support was received for the research, authorship, and/or publication of this article. This work was supported by the South African Research Chairs Initiative of the Department of Science and Technology and National Research Foundation of South Africa (Grant No 98337), as well as grants received from the University of Johannesburg (URC), the African Laser Centre (ALC, Grant No HLHA25X), and the Council for Scientific and Industrial Research (CSIR)-National Laser Centre (NLC) Laser Rental Pool Program.
The authors declare that the research was conducted in the absence of any commercial or financial relationships that could be construed as a potential conflict of interest.
All claims expressed in this article are solely those of the authors and do not necessarily represent those of their affiliated organizations, or those of the publisher, the editors and the reviewers. Any product that may be evaluated in this article, or claim that may be made by its manufacturer, is not guaranteed or endorsed by the publisher.
Almeida-Lopes, L., Rigau, J., Amaro Zângaro, R., Guidugli-Neto, J., and Marques Jaeger, M. M. (2001). Comparison of the low level laser therapy effects on cultured human gingival fibroblasts proliferation using different irradiance and same fluence. Lasers Surg. Med. 29, 179–184. doi:10.1002/lsm.1107
Berger, A. G., Chou, J. J., and Hammond, P. T. (2021). Approaches to modulate the chronic wound environment using localized nucleic acid delivery. Adv. Wound Care (New Rochelle) 10 (9), 503–528. doi:10.1089/wound.2020.1167
Buranasin, P., Mizutani, K., Iwasaki, K., Pawaputanon Na Mahasarakham, C., Kido, D., Takeda, K., et al. (2018). High glucose-induced oxidative stress impairs proliferation and migration of human gingival fibroblasts. PLoS One 13 (8), e0201855. doi:10.1371/journal.pone.0201855
Chen, J. C., Lin, B. B., Hu, H. W., Jin, W. Y., Zhang, F. B., et al. (2014). NGF accelerates cutaneous wound healing by promoting the migration of dermal fibroblasts via the PI3K/Akt-Rac1-JNK and ERK pathways. BioMed Res. Int. 2014, 1–13. doi:10.1155/2014/547187
Cialdai, F., Risaliti, C., and Monici, M. (2022). Role of fibroblasts in wound healing and tissue remodeling on Earth and in space. Front. Bioeng. Biotechnol. 10, 958381. doi:10.3389/fbioe.2022.958381
Deb, D. K., Bao, R., and Li, Y. C. (2017). Critical role of the cAMP–PKA pathway in hyperglycemia–induced epigenetic activation of fibrogenic program in the kidney. FASEB J. 31 (5), 2065–2075. doi:10.1096/fj.201601116R
Deng, C. C., Hu, Y. F., Zhu, D. H., Cheng, Q., Gu, J. J., Feng, Q. L., et al. (2021). Single-cell RNA-seq reveals fibroblast heterogeneity and increased mesenchymal fibroblasts in human fibrotic skin diseases. Nat. Commun. 12, 3709. doi:10.1038/s41467-021-24110-y
Des Jardins-Park, H. E., Foster, D. S., and Longaker, M. T. (2018). Fibroblasts and wound healing: an update. Regen. Med. 13 (5), 491–495. doi:10.2217/rme-2018-0073
Dick, M. K., Miao, J. H., and Limaiem, F. (2023). “Histology, fibroblast,” in StatPearls (Treasure Island (FL): StatPearls Publishing). Available at: https://www.ncbi.nlm.nih.gov/books/NBK541065/ (Accessed May 1, 2023).
Dompe, C., Moncrieff, L., Matys, J., Grzech-Leśniak, K., Kocherova, I., Bryja, A., et al. (2020). Photobiomodulation-underlying mechanism and clinical applications. J. Clin. Med. 9 (6), 1724. doi:10.3390/jcm9061724
Evangelatov, A., Georgiev, G., Arabadjiev, B., Pankov, S., Krastev, P., Momchilova, A., et al. (2022). Hyperglycemia attenuates fibroblast contractility via suppression of TβRII receptor modulated α-smooth muscle actin expression. Biotechnol. Biotechnol. Equip. 36 (1), 35–44. doi:10.1080/13102818.2022.2041486
Farivar, S., Malekshahabi, T., and Shiari, R. (2014). Biological effects of low level laser therapy. J. Lasers Med. Sci. 5 (2), 58–62.
Francesca, C., Chiara, R., and Monica, M. (2022). Role of fibroblasts in wound healing and tissue remodeling on Earth and in space. Front. Bioeng. Biotechnol. 10, 958381. doi:10.3389/fbioe.2022.958381
Giannakopoulos, E., Katopodi, A., Rallis, M., Politopoulos, K., and Alexandratou, E. (2023). The effects of low power laser light at 661 nm on wound healing in a scratch assay fibroblast model. Lasers Med. Sci. 38 (27), 27. doi:10.1007/s10103-022-03670-5
Haas, M. R., Nguyen, D. V., and Shook, B. A. (2021). Recovery of altered diabetic myofibroblast heterogeneity and gene expression are associated with CD301b+ macrophages. Biomedicines 9 (12), 1752. doi:10.3390/biomedicines9121752
Hamblin, M. R. (2018). Mechanisms and mitochondrial redox signaling in photobiomodulation. Photochem Photobiol. 94 (2), 199–212. doi:10.1111/php.12864
Hamdan, S., Pastar, I., Drakulich, S., Dikici, E., Tomic-Canic, M., Deo, S., et al. (2017). Nanotechnology-driven therapeutic interventions in wound healing: potential uses and applications. ACS Cent. Sci. 3 (3), 163–175. doi:10.1021/acscentsci.6b00371
Huang, X., Liu, G., Guo, J., and Su, Z. (2018). The PI3K/AKT pathway in obesity and type 2 diabetes. Int. J. Biol. Sci. 14, 1483–1496. doi:10.7150/ijbs.27173
Jais, S. (2023). Various types of wounds that diabetic patients can develop: a narrative review. Clin. Pathol. 16, 2632010X231205366. doi:10.1177/2632010X231205366
Jere, S. W., Houreld, N. N., and Abrahamse, H. (2021). Effect of photobiomodulation on cellular migration and survival in diabetic and hypoxic diabetic wounded fibroblast cells. Lasers Med. Sci. 36 (2), 365–374. doi:10.1007/s10103-020-03041-y
Karkada, G., Maiya, G. A., Houreld, N. N., Arany, P., Rao Kg, M., Adiga, S., et al. (2023). Effect of photobiomodulation therapy on inflammatory cytokines in healing dynamics of diabetic wounds: a systematic review of preclinical studies. Arch. Physiol. Biochem. 129 (3), 663–670. doi:10.1080/13813455.2020.1861025
Kasowanjete, P., Houreld, N. N., and Abrahamse, H. (2022). The effect of photomodulation on fibroblast growth factor and the Ras/MAPK signalling pathway: a review. J. Wound Care 31 (10), 832–845. doi:10.12968/jowc.2022.31.10.832
Khadra, M., Lyngstadaas, S. P., Haanæs, H. R., and Mustafa, K. (2005). Effect of laser therapy on attachment, proliferation and differentiation of human osteoblast-like cells cultured on titanium implant material. Biomaterials 26, 3503–3509. doi:10.1016/j.biomaterials.2004.09.033
Khalkhal, E., Razzaghi, M., Rostami-Nejad, M., Rezaei-Tavirani, M., Heidari Beigvand, H., and Rezaei Tavirani, M. (2020). Evaluation of laser effects on the human body after laser therapy. J. Lasers Med. Sci. 11 (1), 91–97. doi:10.15171/jlms.2020.15
Khoo, N. K., Shokrgozar, M. A., Kashani, I. R., Amanzadeh, A., Mostafavi, E., Sanati, H., et al. (2014). In vitro therapeutic effects of low level laser at mRNA level on the release of skin growth factors from fibroblasts in diabetic mice. Avicenna J. Med. Biotechnol. 6 (2), 113–118.
Kreisler, M., Christoffers, A. B., Al-Haj, H., Willershausen, B., and d'Hoedt, B. (2002). Low level 809-nm diode laser-induced in vitro stimulation of the proliferation of human gingival fibroblasts. Lasers Surg. Med. 30, 365–369. doi:10.1002/lsm.10060
Lau, P., Bidin, N., Krishnan, G., AnaybBaleg, S. M., Sum, M. B. M., Bakhtiar, H., et al. (2015). Photobiostimulation effect on diabetic wound at different power density of near infrared laser. J. Photochem Photobiol. B 151, 201–207. doi:10.1016/j.jphotobiol.2015.08.009
Lerman, O. Z., Galiano, R. D., Armour, M., Levine, J. P., and Gurtner, G. C. (2003). Cellular dysfunction in the diabetic fibroblast: impairment in migration, vascular endothelial growth factor production, and response to hypoxia. Am. J. Pathol. 162 (1), 303–312. doi:10.1016/S0002-9440(10)63821-7
Li, B., and Wang, J. H. (2011). Fibroblasts and myofibroblasts in wound healing: force generation and measurement. J. Tissue Viability 20 (4), 108–120. doi:10.1016/j.jtv.2009.11.004
Liao, X., Li, S. H., El Akkawi, M. M., Fu, X., Liu, H., and Huang, Y. (2022). Surgical amputation for patients with diabetic foot ulcers: a Chinese expert panel consensus treatment guide. Front. Surg. 9, 1003339. doi:10.3389/fsurg.2022.1003339
Liu, Y., Liu, Y., He, W., Mu, X., Wu, X., Deng, J., et al. (2022). Fibroblasts: immunomodulatory factors in refractory diabetic wound healing. Front. Immunol. 13, 918223. doi:10.3389/fimmu.2022.918223
Nourian, D. A., Mirahmadi, B. F., Chehelgerdi, M., and Raeisi Dehkordi, S. (2019). Skin tissue engineering: wound healing based on stem-cell-based therapeutic strategies. Stem Cell Res. Ther. 10, 111. doi:10.1186/s13287-019-1212-2
Oyebode, O. A., and Houreld, N. N. (2021). “A comparison between photobiomodulation at 830 nm and 660 nm on differentiation in diabetic human skin fibroblast cells,” in SAIP2021 Proceedings, 1–6.
Oyebode, O. A., and Houreld, N. N. (2022). Photobiomodulation at 830 nm stimulates migration, survival and proliferation of fibroblast cells. Diabetes Metab. Syndr. Obes. 15, 2885–2900. doi:10.2147/DMSO.S374649
Petersen, B. J., Bus, S. A., Rothenberg, G. M., Linders, D. R., Lavery, L. A., and Armstrong, D. G. (2020). Recurrence rates suggest delayed identification of plantar ulceration for patients in diabetic foot remission. BMJ Open Diabetes Res. Care 8 (1), e001697. doi:10.1136/bmjdrc–2020–001697
Rahbar, L. E., Fadaei, F. F., Lotfinia, M., Zare, F., Mohammadi Tofigh, A., Abrishami, S., et al. (2020). Photobiomodulation therapy improves the growth factor and cytokine secretory profile in human type 2 diabetic fibroblasts. J. Photochem Photobiol. B 210, 111962. doi:10.1016/j.jphotobiol.2020.111962
Rajendran, N. K., Houreld, N. N., and Abrahamse, H. (2021). Photobiomodulation reduces oxidative stress in diabetic wounded fibroblast cells by inhibiting the FOXO1 signaling pathway. J. Cell Commun. Signal 15 (2), 195–206. doi:10.1007/s12079-020-00588-x
Rani, P. K., Raman, R., Agarwal, S., Paul, P., Satagopan, U., Margabandhu, G., et al. (2005). Diabetic retinopathy screening model for rural population: awareness and screening methodology. Rural. Remote Health 5, 350. doi:10.22605/RRH350
Rocha Júnior, A. M., Vieira, B. J., Andrade, L. C. F., and Aarestrup, F. M. (2007). Effects of low-level laser therapy on the progress of wound healing in humans: the contribution of in vitro and in vivo experimental studies. J. Vasc. Bras. 6 (3), 257–265. doi:10.1590/S1677-54492007000300009
Samuel, K., Sonja, B., Ruiji, G., Dai, R., Knoedler, L., Kauke-Navarro, M., et al. (2023). Fibroblasts – the cellular choreographers of wound healing. Front. Immunol. 14, 1233800. doi:10.3389/fimmu.2023.1233800
Sassoli, C., Chellini, F., Squecco, R., Tani, A., Idrizaj, E., Nosi, D., et al. (2016). Low intensity 635 nm diode laser irradiation inhibits fibroblast-myofibroblast transition reducing TRPC1 channel expression/activity: new perspectives for tissue fibrosis treatment. Lasers Surg. Med. 48 (3), 318–332. doi:10.1002/lsm.22441
Tam, S. Y., Tam, V. C. W., Ramkumar, S., Khaw, M. L., Law, H. K. W., and Lee, S. W. Y. (2020). Review on the cellular mechanisms of low-level laser therapy use in oncology. Front. Oncol. 10, 1255. doi:10.3389/fonc.2020.01255
Wall, I. B., Moseley, R., Baird, D. M., Kipling, D., Giles, P., Laffafian, I., et al. (2008). Fibroblast dysfunction is a key factor in the non-healing of chronic venous leg ulcers. J. Invest. Dermatol 128 (10), 2526–2540. doi:10.1038/jid.2008.114
Wan, R., Weissman, J. P., Grundman, K., Lang, L., Grybowski, D. J., and Galiano, R. D. (2021). Diabetic wound healing: the impact of diabetes on myofibroblast activity and its potential therapeutic treatments. Wound Repair Regen. 29 (4), 573–581. doi:10.1111/wrr.12954
Xuan, Y. H., Huang, B. B., Tian, H. S., Chi, L. S., Duan, Y. M., Wang, X., et al. (2014). High-glucose inhibits human fibroblast cell migration in wound healing via repression of bFGF-regulating JNK phosphorylation. PLoS One 9 (9), e108182. doi:10.1371/journal.pone.0108182
You, H. J., and Han, S. K. (2014). Cell therapy for wound healing. J. Korean Med. Sci. 29 (3), 311–319. doi:10.3346/jkms.2014.29.3.311
Zhao, H., Ji, T., Sun, T., Liu, H., Liu, Y., Chen, D., et al. (2022). Comparative study on Photobiomodulation between 630 nm and 810 nm LED in diabetic wound healing both in vitro and in vivo. J. Innov. Opt. Health Sci. 15 (02), 2250010. doi:10.1142/S1793545822500109
Keywords: fibroblasts, diabetes, fibroblast dysfunction, wound healing, photobiomodulation, diabetic wound
Citation: Jere SW and Houreld NN (2024) Photobiomodulation (PBM): a therapeutic technique targeting fibroblast cell regeneration and survival in diabetic wounds. Front. Photonics 5:1423280. doi: 10.3389/fphot.2024.1423280
Received: 25 April 2024; Accepted: 04 June 2024;
Published: 19 June 2024.
Edited by:
Shuo Chen, Northeastern University, ChinaReviewed by:
Hélio Plapler, Federal University of São Paulo, BrazilCopyright © 2024 Jere and Houreld. This is an open-access article distributed under the terms of the Creative Commons Attribution License (CC BY). The use, distribution or reproduction in other forums is permitted, provided the original author(s) and the copyright owner(s) are credited and that the original publication in this journal is cited, in accordance with accepted academic practice. No use, distribution or reproduction is permitted which does not comply with these terms.
*Correspondence: Sandy Winfield Jere, c2FuZHlqQHVqLmFjLnph
†ORCID: Sandy Winfield Jere, orcid.org/0000-0001-5470-3762; Nicolette Nadene Houreld, orcid.org/0000-0002-1515-8260
Disclaimer: All claims expressed in this article are solely those of the authors and do not necessarily represent those of their affiliated organizations, or those of the publisher, the editors and the reviewers. Any product that may be evaluated in this article or claim that may be made by its manufacturer is not guaranteed or endorsed by the publisher.
Research integrity at Frontiers
Learn more about the work of our research integrity team to safeguard the quality of each article we publish.