- 1Circadian Light Research Center, Stoneham, MA, United States
- 2Department of Structural and Cellular Biology, Tulane University School of Medicine, New Orleans, LA, United States
- 3School of Psychological Sciences, Turner Institute for Brain and Mental Health, Monash University, Melbourne, VIC, Australia
- 4Department of Neuroscience, Rockefeller Neuroscience Institute, West Virginia University, Morgantown, WV, United States
For over a hundred years, the lighting industry has primarily been driven by illumination aesthetics, energy efficiency and product cost with little consideration of the effects of light on health. The recent widespread replacement of traditional light sources by blue-enriched LED lights has heightened concerns about the disruption of the blue-sensitive human circadian system by these LED lights and their impact on the multiple health disorders linked to circadian disruption. Despite these health concerns, less than 0.5% of the lighting sold today modifies spectral content and intensity between day and night. We report that 248 scientists, with a total of 2,697 peer-reviewed publications on light and circadian clocks since 2008, reached consensus on 25 statements about the impact of light on circadian rhythms and health based on accumulated scientific evidence, including support for the widespread introduction of circadian lighting and warning labels on blue-enriched LED lights indicating they “maybe harmful if used at night”.
1 Introduction
The timing, duration, intensity and spectral composition of ocular light exposure have a profound effect on circadian clocks and rhythmic physiological processes. For over 10,000 generations of Homo sapiens, the contrast between bright daylight (10,000–100,000 lux) and nocturnal darkness (0.0001–0.1 lux) robustly entrained the human circadian timing system to the Earth’s 24-h rotation (Moore-Ede et al., 1982). However, for less than four generations over the past century, the natural 24-h cycle of daylight and darkness at night has been replaced in the developed world by electric light. Approximately 90% of our time is now spent indoors (Kleipeis et al., 2001) under electric light, that is, typically 100 times dimmer during the day than natural daylight and 100 times brighter after dusk than even the brightest moonlight.
The circadian disruption of the timing of physiological and biochemical processes that occurs in the absence of robust entrainment by light has been extensively studied over the past 50 years (Moore-Ede et al., 1982; Fishbein et al., 2021). By 2007, there was sufficient evidence for the World Health Organization (WHO) International Agency for Research on Cancer (IARC) (Straif et al., 2007) to classify night shift work with circadian disruption as a probable (group 2 A) human carcinogen based on human epidemiological studies and research with animal models. Since then, a large number of studies have linked circadian disruption caused by inadequate exposure to light during daytime hours and exposure to electric light at night to a wide range of health disorders, including obesity, diabetes, heart disease, reproductive and psychiatric disorders and certain endocrine-sensitive cancers, such as breast cancer (Bedrosian et al., 2016; Blask et al., 2011; Fishbein et al., 2021; Kim et al., 2023; Rüger and Scheer 2009; Stevens et al., 2013; Straif et al., 2007).
The circadian clocks that regulate the timing of physiological processes, with molecular mechanisms defined by the 2017 Nobel Prize in Physiology or Medicine winners Jeffrey Hall, Michael Rosbash and Michael Young (Van Laake et al., 2018), are uniquely sensitive to the blue content of visible light. This is because the melanopic intrinsically photosensitive retinal ganglion cells (ipRGCs) that entrain circadian clocks to the environmental light dark cycle have a peak sensitivity to ∼480 nm blue light (Bailes and Lucas 2013). In light-adapted people with similar normal prior daytime light exposure, and then studied under seven different LED & fluorescent lights with different spectral power distributions, all delivering the same 540 lux desktop light intensity, the full-width half maximum (FWHM) melatonin suppression was 440–495 nm and the peak spectral sensitivity was 477 nm (i.e., ∼480 nm blue) (Moore-Ede et al., 2020). Further evidence for how much more potent ∼480 nm blue light is in shifting circadian clocks than the rest of the 380–780 nm visible light spectrum is provided by the comparable amplitude phase response curves produced by a 12 μW/cm2 monochromatic 480 nm blue light source and a 3,000 μW/cm2 polychromatic white light source (Rüger et al., 2013).
Since 2013, electric lighting has been transformed by the introduction of highly energy efficient LED (light-emitting diode) lights and the progressive regulatory-enforced phase outs of other less efficient light sources, including incandescent, halogen, CCFL, and fluorescent lights (California Assembly Bill 2022; Directorate-General for Energy 2018; U.S. Department of Energy 2022). However, most LED lights optimize efficacy by utilizing a blue chip, which increases the relative percentage of 440–495 nm blue content in the white light spectrum compared to other sources of light (Zielinska-Dabkowska 2018).
The obvious solution is to provide blue-rich light during the daytime to help entrain circadian rhythms and blue-depleted light during the evening hours to protect against circadian disruption. However, the $130 billion/year lighting industry driven by energy-efficiency incentives offers mostly unmodulated blue-chip LED light. Less than 0.5% (0.26/130 billion US dollars) of the lights sold today have any circadian supportive properties (Data Bridge Market Research 2023). Because of the importance of balancing energy efficiency and human health concerns, we sought to determine if a scientific consensus can be documented between currently active scientists, which would 1) clarify the core well-established findings of circadian lighting science, 2) provide direction to the lighting industry on the properties of the lights they should be manufacturing and marketing to promote health and wellbeing, 3) provide guidance to lighting consumers on the evidence-based characteristics of the lights they should be buying, and 4) provide the scientific community with guidance on unresolved questions and issues that should be prioritized in future scientific investigations.
2 Method
2.1 Survey population
A search of PubMed with the terms “circadian” + “light” identified 10,002 peer-reviewed scientific articles published between 1 April 2008 and 1 April 2022 by 29,455 unique authors. As shown in the flow chart in Figure 1, we identified all the authors out of this sample who had published four or more peer-reviewed articles, and we built an email list of 2,142 authors using their most recent identifiable email addresses on academic and publisher websites. Three email invitations to participate were sent to the email list at weekly intervals in August–September 2022. A total of 1,156 (54%) of these scientific authors received and opened an email invitation to participate in the Scientific Consensus Survey on Circadian Light, and 248 (21.5%) of the recipients completed the survey.
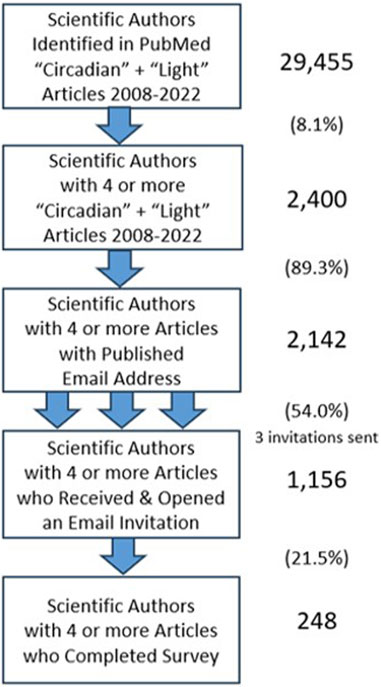
FIGURE 1. Process for the recruitment of survey respondents. For each step, number of authors and percentages (as compared to the previous step) are indicated.
The 248 respondents had published an average of 10.8 (median: 7; range: 4–68) peer-reviewed articles that met the “circadian” + “light” criterion in the 2008–2022 study period. In comparison, the 1894 authors who did not complete the survey or did not open the email had an average of 8.3 peer reviewed articles (median: 6, range: 4–87) (p < 0.001; Mann-Whitney Test). A total of 111 of the respondents (44.8%) were based in North America, 96 (38.7%) were based in Europe, 17 (6.9%) were based in South America, 12 (4.8%) were based in Australia/New Zealand, 10 (4.0%) were based in Asia, and the remaining 2 (0.8%) were based in Africa. The publications of each of the 248 respondents were reviewed to classify the respondents’ primary research models: human research (studies including human subjects and human-related reviews and translational research) and nonhuman research (studies including mammalian, other vertebrate species, invertebrates, plants and cellular/molecular systems). There were 103 researchers who had performed human research and 145 researchers who had not conducted any human research. Subgroup comparisons of the frequencies of the response options for each statement were conducted using chi-square tests.
2.2 Survey design
Forty statements were developed for testing by the authors of this article. Thirty were potential factual conclusions summarizing the scientific literature with the following response options: 1) Don’t Know, 2) No Evidence, 3) Limited Evidence, 4) Good Evidence or 5) Well-Established. Five were potential practical advice conclusions about lighting with the same set of response options. The five remaining statements were potential expert policy statements based on the scientific literature with the following response options: 1) Don’t Know, 2) Strongly Disagree, 3) Disagree, 4) Agree or 5) Strongly Agree.
2.3 Definition of consensus
In a large and diverse group of scientists addressing complex scientific questions, achieving unanimous agreement is not feasible or expected. In Delphi health policy consensus studies, where there are several rounds of feedback between participants enabling participants to change their minds and refine their answers based on new data and the judgments of their peers, 70%–75% agreement is often accepted as consensus (Barrios et al., 2021; de Raaff et al., 2017). In this single iteration survey, with no opportunity to revise answers based on feedback from other participants, we defined a consensus as when two-thirds of the respondents (66.7%) supported the statement. The responses “Good Evidence” and “Well-Established” were combined for evidence-based statements, and “Agree” and “Strongly Agree” were combined for expert policy statements. The “Don’t Know” option on each tested statement allowed scientists to abstain from the evaluation of the strength of evidence on specific topics with which they were not familiar and therefore to be excluded from the consensus calculation on those topics.
3 Results
Consensus was reached on 25 out of the 40 test statements, listed in Table 1, and Table 2 lists the test statements for which two-thirds (66.6%) consensus was not reached. The ranking of support for every scientific statement is displayed in the bar graph in Figure 2 (top), and the ranking of the expert opinions on practical applications in Figure 2 (bottom).
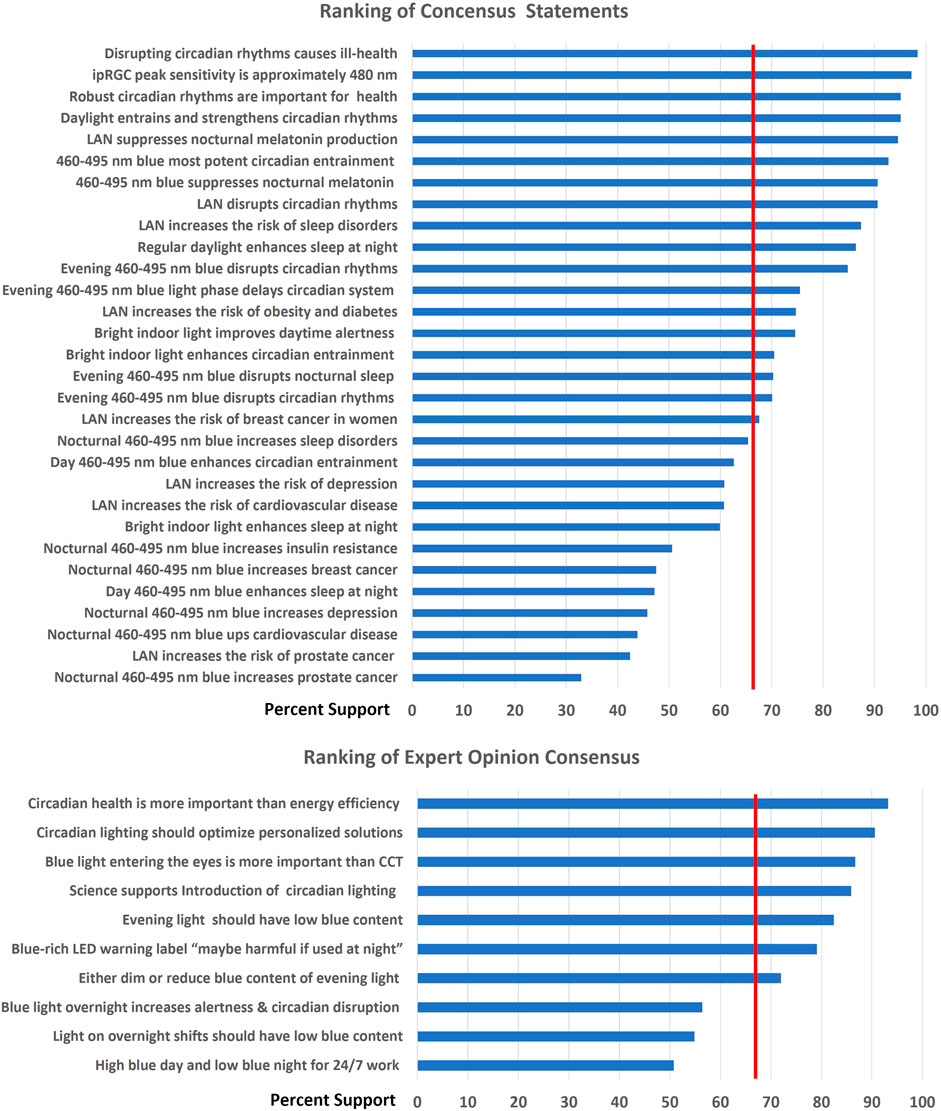
FIGURE 2. Ranking of level of support for each statement. Top: Scientific statements. Bottom: Opinions on practical applications. Statement support was based on the combination of the response options “well established” and “good evidence” (scientific statements), and on the combination of the response options “strongly agree” and “agree” (opinions). Vertical red line = 66.6% consensus level. LAN = Light at Night.
The first set of consensus statements related to the role of regular exposure to bright light during the day (daylight or bright indoor electric light) in enhancing the robust entrainment of circadian rhythms and maintaining health. There was strong consensus that robust circadian rhythms are important for health (95.1%) and that disrupting circadian rhythms can cause ill health (98.4%).
There was also consensus that increasing daytime light intensity indoors within the normal indoor intensity range of 50–500 lux enhances circadian entrainment and strengthens circadian rhythms (70.5%), improves daytime alertness and reduces sleepiness (74.7%). The majority (59.1%) of respondents indicated there was “good evidence” or it was “well established” that increasing indoor daytime light intensity enhances sleep at night, but this did not reach the two-thirds consensus level.
The second set of consensus statements related to the impact of electric light at night. There was strong consensus that increasing indoor light intensity at night increases the disruption of circadian rhythms (90.6%) and increases the suppression of nocturnal melatonin production (94.6%). There was also consensus that repetitive and prolonged exposure to light at night bright enough to cause circadian disruption increases the risk of breast cancer in women (67.6%), obesity and diabetes (74.7%), and sleep disorders (87.4%). The majority of respondents agreed that there was good support (“good evidence” or “well established”) that repetitive and prolonged exposure to light at night that was bright enough to cause circadian disruption increases cardiovascular disease (60.7%) and depression (60.8%), but these did not reach the two-thirds consensus level.
The third set of consensus statements related to the impact of the blue wavelengths in white illumination on the human circadian system. There was strong consensus that “the sensitivity peak of the ipRGC melanopic receptors in the human retina is approximately 480 nm in the blue part of the visible spectrum” (97.2%) and that “the most potent wavelengths for circadian entrainment are 460–495 nm blue light near to the sensitivity peak of the ipRGC melanopic receptors” (92.7%).
There was also consensus that “blue-enriched (460–495 nm) light in the evening (during the 3 hours before bedtime) disrupts nocturnal sleep more (70.3%), phase delays the circadian system more (75.5%) and disrupts circadian rhythms more (70.1%) than blue-depleted light at the same intensity. There was also strong consensus that exposure to 460–495 nm blue light at night suppresses melatonin production (90.6%) and disrupts circadian rhythms” (84.9%).
The fourth set of consensus statements related to the practical application of circadian science to lighting. There was consensus that “light used in the evening (during the 3 hours before bedtime) should have as little blue content as practically possible” (82.5%) and that “the risk of circadian disruption during the 3 hours before bedtime can be reduced either by 1) dimming indoor lighting which may compromise the ability to perform visual work tasks, or 2) reducing the blue content of indoor lighting maintained at the intensity required for visual tasks” (72.0%).
The participants were also asked their expert opinion about the implications of circadian science for the design and implementation of lighting. There was consensus that “the blue content of light entering the eyes is much more important in determining circadian health outcomes than the correlated color temperature (CCT) of the light source” (86.7%) and “increasing the energy efficiency of lights is desirable, but not if it increases the risks of causing circadian disruption and serious illness (93.2%).
Summarizing their expert opinion, there was consensus that “there is now sufficient evidence to support the widespread introduction of circadian lighting that adjusts light intensity and blue content across day and night to maintain robust circadian entrainment and health (85.9%), and “LED lights with high 460–495 nm blue content should carry the warning label “maybe harmful if used at night” (79.1%). They also reached consensus that “there is significant variation in individual sensitivity to light, therefore circadian lighting should be optimized where possible using personalized solutions” (90.6%).
Comparisons between the response frequencies of the two subgroups—respondents whose research was all or partly human research and respondents who did not do any human research—showed that there was overall good agreement between the two subgroups, with significant differences being found in the evaluation of only four of the forty statements. Three of these statements:
• Increasing indoor light intensity at night increases the suppression of nocturnal melatonin production
• Exposure to 460–495 nm blue light at night suppresses melatonin production
• There is significant variation in individual sensitivity to light; therefore, circadian lighting should be optimized where possible using personalized solutions
had a higher consensus score in the human research subgroup than in the nonhuman research subgroup (but reached consensus in both subgroups).
One statement:
• Repetitive and prolonged exposure to light at night bright enough to cause circadian disruption increases the risk of cardiovascular disease
obtained slightly more support (and reached the consensus criterion in the nonhuman research subgroup).
4 Discussion
A consensus survey, such as this, serves a different purpose than a literature review or a meta-analysis. It asked 248 scientists who have had significant research and publication experience in circadian rhythms and light interactions over the past 15 years on what can be considered established science in this field, and where there are still open questions that will require additional research to resolve. Their answers were informed by the published literature and meta-analyses and their own awareness of yet to be published research. We made it clear in the title of the survey “Scientific Consensus on Circadian Light”, and in the email invitations, that we were examining where a consensus could be reached on core principles of circadian rhythms and light that could be communicated to non-scientists in the lighting industry. All active scientists who have published four or more peer-reviewed articles identified in a PubMed search with the terms “circadian” + “light” since 2008, when the WHO IARC carcinogenic finding was first reported (Straif et al., 2007), and for whom we were able to identify an email address, were invited to participate in the survey. There was no preselection of potential participants other than by these criteria. However, scientists who have changed employment so their email address was out of date, who were not corresponding authors with their email address published in their articles, or who were not sufficiently familiar with our English language survey may be underrepresented in the sample.
The responses to the factual survey questions were, as expected, consistent with publications and reviews of the scientific literature, meta-analyses and scientific panel reports. For example, the survey consensus that repetitive and prolonged exposure to light at night bright enough to cause circadian disruption increases the risk of obesity and diabetes is supported by reviews (Fishbein et al., 2021; Rüger and Scheer 2009), meta-analyses (Lai et al., 2020) and scientific panels (Stevens et al., 2013). Similarly, the survey consensus that light at night bright enough to cause circadian disruption increases the risk of breast cancer in women is consistent with reviews (Blask et al., 2011), meta-analyses (He et al., 2015; Urbano et al., 2021) and scientific panels (IARC Working Group on the Evaluation of Carcinogenic Risks to Humans 2010; U.S. National Toxicology Program 2021).
The participants were also asked for their expert opinions on matters of health policy related to circadian health and light exposure, especially concerning the blue wavelength content of light. Blue-enriched LED lights have gained dominance in the lighting market because they can provide 100–200 lumens per watt of energy-efficient lighting compared to 15 lumens/watt for incandescent and up to 90 lumens/watt for fluorescent lights. This breakthrough invention in lighting using gallium nitride (GaN) crystals was recognized by the 2014 Nobel Prize for Physics awarded to three Japanese scientists, Isamu Akasaki, Hiroshi Amano and Shuji Nakamura (Gibney 2014). Blue-enriched LEDs have grown from less than 1% market share in 2013 to greater than 80% market share in 2022, aided by government policies and utility rebates that incentivize the replacement of traditional lighting with LEDs to achieve energy savings, and further promoted by regulatory bans on incandescent, halogen and fluorescent lighting (U.S. Department of Energy 2022; California Assembly Bill 2023; Directorate-General for Energy 2018).
Exposure to blue-rich light in the evening and night hours raises significant health issues because there is a more than five-fold variation in nocturnal melatonin suppression between different LED light sources at the same lux intensity depending on the 440–495 nm μW/cm2 blue irradiance of each LED light source (Moore-Ede 2021). Even small amounts of blue irradiance in visible light during nocturnal hours can cause circadian disruption and increase the risk of health disorders (Rahman et al., 2011; Vethe et al., 2021; Vethe et al., 2022; Balserak et al., 2022; Cain et al., 2020; Schöllhorn et al., 2023).
Consistent with this, the survey respondents agreed that blue-enriched (460–495 nm) light in the evening (during the 3 hours before bedtime) disrupts nocturnal sleep, phase delays the circadian system and disrupts circadian rhythms more than blue-depleted light at the same intensity. They also reached consensus that light used in the evening (during the 3 hours before bedtime) should have as little blue content as practically possible, and that “LED lights with high 460–495 nm blue content should carry the warning label “maybe harmful if used at night”. The survey question focused specifically on blue-rich LED lighting, because this kind of lighting currently predominates, with incandescent, halogen and fluorescent lights now largely banned.
The drive to reduce energy consumption by increasing the electrical efficiency of lighting prompted new rules which significantly increase the minimum allowable lumens/watt of general service lamps. Effective 1 August 2023, the US Department of Energy regulations require a minimum of 45 lumens/watt which effectively bans all incandescent and halogen lamps (U.S. Department of Energy 2022). And new rules proposed in 2023 to take effect in 2029 increase the minimum to 120 lumens per watt which will effectively exclude all lamps except for blue-enriched LEDs (U.S. Department of Energy 2023). However, the scientific consensus in our survey was that increasing the energy efficiency of lights is desirable, but not if it increases the risks of causing circadian disruption and serious illness. One of the problems is that the “lumens per watt” metric used by the DOE to assess energy efficiency focuses on light wavelengths between 500 and 600 nm, with only 7% of the lumens power reflecting biologically active <500 nm blue wavelengths, so these biologically important watts of energy are considered “inefficient” when lumens per watt is calculated.
The consensus study also agreed that the blue content of light entering the eyes is much more important in determining circadian health outcomes than the correlated color temperature (CCT) of the light source. CCT has sometimes been used as a proxy for biological effectiveness (Stevens et al., 2013) because low CCT lights tend to have less blue content than high CCT lights. However, CCT has been shown to be an unreliable predictor of the biological potency of light (Esposito and Houser 2022).
A secondary goal of this survey was to help guide future scientific investigation. Failure to reach two-thirds consensus on 15 of the tested statements indicates that more definitive research is required on these topics, but does not necessarily mean they are not factually correct. Another possible reason for not reaching consensus is that individual scientists may have a lack of familiarity with the recent literature on a specific issue since PubMed citations with the terms “circadian” + “light” currently exceed 1,000/year.
For eight of these statements, the majority (50.6%–65.4%) of respondents ranked them as “good evidence/well established”, but a sufficient fraction of respondents ranked them as having “limited evidence” to bring the overall consensus below the two-thirds (66.6%) support criterion. For example, the current scientific controversy on whether to use blue-rich light on overnight work shifts is reflected in the three expert opinion statements supported by 50.8%–56.4% of respondents. Blue-rich light does increase alertness in short studies of overnight shifts (Sletten et al., 2021), but can also cause circadian disruption (Stenvres et al., 2019) and increased errors (Griepentrog et al., 2018) especially when used on a longer-term basis.
Similarly, while there was consensus that repetitive and prolonged exposure to light at night increases the risk of sleep disorders, obesity, diabetes, and breast cancer in women, consensus was not reached (42%) regarding an increased risk for prostate cancer in men, or for an increased risk for cardiovascular disease (61%) and depression (61%).
There was consensus that nocturnal exposure to 460–495 nm blue light suppresses melatonin production, and phase shifts and disrupts circadian rhythms (70.1%–75.5%), but there was not consensus that blue light at night increases the risk for specific diseases, because of the limited number of direct studies linking blue-rich light to a specific disease. However, for the practical recommendations, the respondents appeared comfortable with considering a two-step argument: 1) Blue light is most potent at disrupting circadian rhythms, and 2) Circadian disruption is associated with multiple health disorders and diseases.
Regarding daytime indoor lighting, there was consensus that increasing light intensity can enhance circadian entrainment and strengthen circadian rhythms as well as improve daytime alertness, but consensus was not reached on whether increasing indoor light intensity enhances sleep at night.
In this survey, potential Type 1 errors (falsely identifying a statement as well established) included a) Respondents not sufficiently familiar with the relevant scientific articles on the specific subject, and b) 248 respondents not representative of the 2,400 scientific authors with 4 or more publications on “circadian” + “light” since 2008. Potential Type 2 errors (falsely labelling a statement as not well established) included a) Respondents not sufficiently familiar with the relevant scientific articles on the specific subject, b) 248 respondents not representative of the 2,400 scientific authors with 4 or more publications on “circadian” + “light” since 2008, and c) Respondents not labelling a scientific conclusion as well-established when they are applying for funding to study that subject. Advances in spectral engineering have enabled the development of blue-depleted LED lights for nocturnal use and ∼480 nm blue-enriched LED lights for daytime without significantly compromising color or energy efficiency (David et al., 2019; Moore-Ede 2021). This means there is little excuse not to use lighting, which modifies blue content and intensity over the day-night cycle. Recent demonstrations of significant returns on investments in circadian lighting, such as a 43% reduction in elderly falls and reduced depression and agitation in nursing homes (Grant et al., 2022; Figueiro et al., 2014) and improvement of sleep and reduction of delirium in intensive care patients (Engwall et al., 2017; Simons et al., 2016), will help transition circadian lighting from a “nice to have” to a “must have”.
The 248 scientists participating in this Consensus Survey, who each had published multiple peer-reviewed articles addressing the interactions between light and the circadian system, reached a clear consensus that “there is now sufficient evidence to support the widespread introduction of circadian lighting that adjusts light intensity and blue content across day and night to maintain robust circadian entrainment and health”. This conclusion was supported by consensus on 24 other specific statements about the disruptive effects of light at night, and the lack of adequate light during the day, on circadian rhythms and health. With 99.5% of currently manufactured lights only designed to illuminate visual tasks without any features designed to support circadian rhythms, this is an important message that it is critical to also use lighting to support human health and wellbeing.
Data availability statement
The raw data supporting the conclusion of this article will be made available by the authors, without undue reservation.
Author contributions
MM-E: Conceptualization, Funding acquisition, Supervision, Writing–original draft, Writing–review and editing, Methodology. DB: Methodology, Writing–review and editing, Conceptualization. SC: Conceptualization, Methodology, Writing–review and editing. AH: Conceptualization, Methodology, Writing–review and editing, Formal Analysis. RN: Conceptualization, Methodology, Writing–review and editing.
Funding
The authors declare financial support was received for the research, authorship, and/or publication of this article. Fees for using the QuestionPro survey website and partial costs of compiling the email database were funded by Korrus, Inc. The funder had no role in the design of the study; in the collection, analyses, or interpretation of data; in the writing of the manuscript; or in the decision to publish the results.
Acknowledgments
We thank R. Guttkuhn for assistance with the analysis of the PubMed database, P. Jones, M. Laungani, and A. Godkhindi for assistance with the graphical design of the online consensus survey, and B. Harrison for helpful discussions.
Conflict of interest
SC is an inventor on a provisional patent on a circadian lighting system (WO2021102504A1) and is co-Founder and co-Director of Circadian Health Innovations, a circadian wearables company. DB, RN, and SC were consensus survey participants who met the qualification standard. MM-E and AH are inventors on patents US9827440B2, CN105265025B, EP2982224B1, JP6391669B2, CA2908659A1, KR101986700B1, and AU2018241062A1 and patent application PCT/US 2019/041728 that disclose circadian optimized spectrally engineered light sources, including day and night LEDs. Neither MM-E nor AH participated in the consensus survey. From 2010 to April 2022, MM-E was CEO of Circadian Zirclight Inc., a company developing, patenting and selling spectrally engineered circadian lighting, whose IP (including the above patents) and technology were acquired by Korrus in April 2022. The survey was conceived, developed and conducted in the summer of 2022 after the completion of the IP acquisition. MM-E received compensation from Korrus and holds equity in the CIRCADIAN companies which hold a minor (<1%) equity interest in Korrus.
The author (SC) declared that they were an editorial board member of Frontiers at the time of submission. This had no impact on the peer review process and the final decision.
Publisher’s note
All claims expressed in this article are solely those of the authors and do not necessarily represent those of their affiliated organizations, or those of the publisher, the editors and the reviewers. Any product that may be evaluated in this article, or claim that may be made by its manufacturer, is not guaranteed or endorsed by the publisher.
References
Bailes, H. J., and Lucas, R. J. (2013). Human melanopsin forms a pigment maximally sensitive to blue light (λmax ≈ 479 nm) supporting activation of Gq/11 and Gi/o signalling cascades. Proc. Biol. Sci. 280, 20122987. doi:10.1098/rspb.2012.2987
Balserak, B., Hermann, R., Hernandez, T. L., Buhimschi, C., and Park, C. (2022). Evening blue-light exposure, maternal glucose, and infant birthweight. Ann. N. Y. Acad. Sci. 1515 (1), 276–284. doi:10.1111/nyas.14852
Barrios, M., Guilera, G., Nuño, L., and Gómez-Benito, J. (2021). Consensus in the delphi method: what makes a decision change? Technol. Forecast. Soc. Change 163, 120484. doi:10.1016/j.techfore.2020.120484
Bedrosian, T. A., Fonken, L. K., and Nelson, R. J. (2016). Endocrine effects of circadian disruption. Annu. Rev. Physiol. 78, 109–131. doi:10.1146/annurev-physiol-021115-105102
Blask, D. E., Hill, S. M., Dauchy, R. T., Xiang, S., Yuan, L., Duplessis, T., et al. (2011). Circadian regulation of molecular, dietary, and metabolic signaling mechanisms of human breast cancer growth by the nocturnal melatonin signal and the consequences of its disruption by light at night. J. Pineal Res. 51 (3), 259–269. doi:10.1111/j.1600-079X.2011.00888.x
Cain, S. W., McGlashan, E. M., Vidafar, P., Mustafovska, J., Curran, S., Wang, X., et al. (2020). Evening home lighting adversely impacts the circadian system and sleep. Sci. Rep. 10, 19110. doi:10.1038/s41598-020-75622-4
California Assembly Bill (2022). Fluorescent lamps: sale and distribution: prohibition. Available at: https://legiscan.com/CA/text/AB2208/2021 (Accessed May 30, 2023).
Data Bridge Market Research (2022). Global circadian rhythm lighting market – industry trends and forecast to 2030. Available at: https://www.databridgemarketresearch.com/reports/global-circadian-rhythm-lighting-market (Accessed May 26, 2023).
David, A., Sahlhoff, D., and Wisser, M. (2019). Human perception of light chromaticity: short-wavelength effects in spectra with low circadian stimulation, and broader implications for general LED sources. Opt. Express 27 (22), 31553. doi:10.1364/OE.27.031553
de Raaff, C. A. L., Gorter-Stam, M. A. W., de Vries, N., Sinha, A. C., Bonjer, H. J., Chung, F., et al. (2017). Perioperative management of obstructive sleep apnea in bariatric surgery: A consensus guideline. Surg. Obes. Relat. Dis. 13, 1095–1109. doi:10.1016/j.soard.2017.03.022
Directorate-General for Energy (2018). New lightbulb rules will enable household energy savings and help reduce greenhouse gas emissions. Brussels, Belgium: European Commission.
Engwall, M., Fridh, I., Jutengren, G., Bergbom, I., Sterner, A., and Lindahl, B. (2017). The effect of cycled lighting in the intensive care unit on sleep, activity and physiological parameters: A pilot study. Intensive Crit. Care Nurs. 41, 26–32. doi:10.1016/j.iccn.2017.01.009
Esposito, T., and Houser, K. (2022). Correlated color temperature is not a suitable proxy for the biological potency of light. Sci. Rep. 12, 20223. doi:10.1038/s41598-022-21755-7
Figueiro, M. G., Plitnick, B. A., Lok, A., Jones, G. E., Higgins, P., Hornick, T. R., et al. (2014). Tailored lighting intervention improves measures of sleep, depression, and agitation in persons with Alzheimer's disease and related dementia living in long-term care facilities. Clin. Interv. Aging 9, 1527–1537. doi:10.2147/CIA.S68557
Fishbein, A. B., Knutson, K. L., and Zee, P. C. (2021). Circadian disruption and human health. J. Clin. Invest. 131 (19), e3148286. doi:10.1172/JCI148286
Gibney, E. (2014). Nobel for blue LED that revolutionized lighting. Nature 514, 152–153. doi:10.1038/514152a
Grant, L. K., St Hilaire, M. A., Heller, J. P., Heller, R. A., Lockley, S. W., and Rahman, S. A. (2022). Impact of upgraded lighting on falls in care home residents. J. Am. Med. Dir. Assoc. 23 (10), 1698–1704.e2. doi:10.1016/j.jamda.2022.06.013
Griepentrog, J. E., Labiner, H. E., Gunn, S. R., and Rosengart, M. R. (2018). Bright environmental light improves the sleepiness of nightshift ICU nurses. Crit. Care 22 (1), 295. doi:10.1186/s13054-018-2233-4
He, C., Anand, S. T., Ebell, M. H., Vena, J. E., and Robb, S. W. (2015). Circadian disrupting exposures and breast cancer risk: A meta-analysis. Int. Arch. Occup. Environ. Health 88 (5), 533–547. doi:10.1007/s00420-014-0986-x
IARC Working Group on the Evaluation of Carcinogenic Risks to Humans (2010). “Painting, firefighting, and shiftwork,” in IARC monographs on the evaluation of carcinogenic risks in humans (Lyon, France: WHO, IARC), 563–764.
Kim, M., Vu, T. H., Maas, M. B., Braun, R. I., Wolf, M. S., Roenneberg, T., et al. (2023). Light at night in older age is associated with obesity, diabetes, and hypertension. Sleep 46 (3), zsac130. doi:10.1093/sleep/zsac130
Klepeis, N. E., Nelson, W. C., Ott, W. R., Robinson, J. P., Tsang, A. M., Switzer, P., et al. (2001). The national human activity pattern survey (nhaps): A resource for assessing exposure to environmental pollutants. J. Expo. Anal. Environ. Epidemiol. 11 (3), 231–252. doi:10.1038/sj.jea.7500165
Lai, Y. L., Sarkar, C., Ni, M. Y., Gallacher, J., and Webster, C. (2020). Exposure to light at night (LAN) and risk of obesity: A systematic review and meta-analysis of observational studies. Environ. Res. 187, 109637. doi:10.1016/j.envres.2020.109637
Moore Ede, M., Sulzman, F. M., and Fuller, C. A. (1982). The clocks that time us: Physiology of the circadian timing system. Cambridge, MA, USA: Harvard University Press.
Moore-Ede, M., Heitmann, A., and Guttkuhn, R. (2020). Circadian potency spectrum with extended exposure to polychromatic white LED light under workplace conditions. J. Biol. Rhythms 35 (4), 405–415. doi:10.1177/0748730420923164
Moore-Ede, M. (2021). LEDs must spectrally balance illumination, circadian health, productivity, and energy efficiency. Pennsylvania: LED Magazine. Available at: https://www.ledsmagazine.com/lighting-health-wellbeing/article/14199941/ideal-led-lighting-must-balance-multiple-objectives-magazine. (Accessed June 3, 2023)
Rahman, S. A., Marcu, S., Shapiro, C. M., Brown, T. J., and Casper, R. F. (2011). Spectral modulation attenuates molecular, endocrine, and neurobehavioral disruption induced by nocturnal light exposure. Am. J. Physiol. Endocrinol. Metab. 300 (3), 518–527. doi:10.1152/ajpendo.00597.2010
Rüger, M., and Scheer, F. (2009). Effects of circadian disruption on the cardiometabolic system. Rev. Endocr. Metab. Disord. 10 (4), 245–260. doi:10.1007/s11154-009-9122-8
Rüger, M., St Hilaire, M. A., Brainard, G. C., Khalsa, S. B. S., Kronauer, R. E., Czeisler, C. A., et al. (2013). Human phase response curve to a single 6.5 h pulse of short-wavelength light: blue light phase response curve. J. Physiol. 591, 353–363. doi:10.1113/jphysiol.2012.239046
Schöllhorn, I., Stefani, O., Robert, J., Lucas, R. J., Spitschan, M., Slawik, H. C., et al. (2023). Melanopic irradiance defines the impact of evening display light on sleep latency, melatonin and alertness. Commun. Biol. 6 (1), 228. doi:10.1038/s42003-023-04598-4
Simons, K. S., Laheij, R. J. F., van den Boogaard, M., Moviat, M. A., Paling, A. J., Polderman, F. N., et al. (2016). Dynamic light application therapy to reduce the incidence and duration of delirium in intensive-care patients: A randomised controlled trial. Lancet Respir. Med. 4 (3), 194–202. doi:10.1016/S2213-2600(16)00025-4
Sletten, T. L., Raman, B., Magee, M., Ferguson, S. A., Kennaway, D. J., Grunstein, R. R., et al. (2021). A blue-enriched, increased intensity light intervention to improve alertness and performance in rotating night shift workers in an operational setting. Nat. Sci. Sleep. 13, 647–657. doi:10.2147/NSS.S287097
Stenvres, D. J., Scheer, F. A. J. L., Schrauwen, P., la Fleur, S. E., and Kalsbeek, A. (2019). Circadian clocks and insulin resistance. Nat. Rev. Endocrinol. 15 (2), 75–89. doi:10.1038/s41574-018-0122-1
Stevens, R. G., Brainard, G. C., Blask, D. E., Lockley, S. W., and Motta, M. E. (2013). Adverse health effects of nighttime lighting: comments on American Medical Association policy statement. Am. J. Prev. Med. 45 (3), 343–346. doi:10.1016/j.amepre.2013.04.011
Straif, K., Baan, R., Grosse, Y., Secretan, B., Ghissassi, F. E. I., Bouvard, V., et al. (2007). Carcinogenicity of shift-work, painting, and fire-fighting. Lancet Oncol. 8 (12), 1065–1066. doi:10.1016/S1470-2045(07)70373-X
Urbano, T., Vincenti, M., Wise, L. A., and Filippini, T. (2021). Light at night and risk of breast cancer: A systematic review and dose–response meta-analysis. Int. J. Health Geogr. 20, 44. doi:10.1186/s12942-021-00297-7
U.S. Department of Energy (2022). Energy conservation program: definitions for general service lamps (final rule 87FR27461). Fed. Regist. 87 (89).
U.S. Department of Energy (2023). Energy conservation program: energy conservation standards for general service lamps: notice of proposed rulemaking and announcement of public meeting. Available at: https://www.federalregister.gov/documents/2023/01/11/2022-28072/energy-conservation-program-energy-conservation-standards-for-general-service-lamps (Accessed June 2, 2023).
U.S. National Toxicology Program (2021). NTP cancer hazard assessment report on night shift work and light at night. Research Triangle Park, NC, USA: U.S Department of Health and Human Services, National Institute of Environmental Health Sciences. Available at: https://ntp.niehs.nih.gov/ntp/results/pubs/cancer_assessment/lanfinal20210400_508.pdf.
Van Laake, L. W., Luscher, T. F., and Young, M. E. (2018). The circadian clock in cardiovascular regulation and disease: lessons from the Nobel prize in Physiology or Medicine 2017. Eur. Heart J. 39, 2326–2329. doi:10.1093/eurheartj/ehx775
Vethe, D., Drews, H. J., Scott, J., Engstrøm, M., Heglum, H. S. M., Grønli, J., et al. (2022). Evening light environments can be designed to consolidate and increase the duration of REM-sleep. Sci. Rep. 12, 8719. doi:10.1038/s41598-022-12408-w
Vethe, D., Scott, J., Engstrøm, M., Salvesen, Ø., Sand, T., Olsen, A., et al. (2021). The evening light environment in hospitals can be designed to produce less disruptive effects on the circadian system and improve sleep. Sleep 44 (3), zsaa194–12. doi:10.1093/sleep/zsaa194
Keywords: circadian disruption, light, LED, blue light, ipRGC, obesity, diabetes, breast cancer
Citation: Moore-Ede M, Blask DE, Cain SW, Heitmann A and Nelson RJ (2023) Lights should support circadian rhythms: evidence-based scientific consensus. Front. Photonics 4:1272934. doi: 10.3389/fphot.2023.1272934
Received: 04 August 2023; Accepted: 20 September 2023;
Published: 04 October 2023.
Edited by:
Michael Smolensky, The University of Texas at Austin, United StatesReviewed by:
Philip Lewis, University of Cologne, GermanySubhabrata Das, JPMorgan Chase & Co, United States
Copyright © 2023 Moore-Ede, Blask, Cain, Heitmann and Nelson. This is an open-access article distributed under the terms of the Creative Commons Attribution License (CC BY). The use, distribution or reproduction in other forums is permitted, provided the original author(s) and the copyright owner(s) are credited and that the original publication in this journal is cited, in accordance with accepted academic practice. No use, distribution or reproduction is permitted which does not comply with these terms.
*Correspondence: Martin Moore-Ede, mme@circadian.com