Plasmonics has been a flourishing field since the late ‘80s of the last century. Given the number of outstanding developments even without resorting to metrics–it is straightforward to address the 2 decades crossing the new millennium as the golden era of Plasmonics. The unique capabilities of plasmonic nanostructures to collect, direct and enhance light at length scales much below the operating wavelength granted them the name “antennas for light”. These features were crucial for the vast deployment of Plasmonics to a variety of tasks, spanning from light harvesting (Atwater and Albert 2010) to molecular sensing (Homola 2008; Saha et al., 2012), bio-imaging (Meola et al., 2018; Bocková et al., 2019; Wu et al., 2019) and plasmon-enhanced spectroscopy (Zhang et al., 2013, Ding et al., 2016). Another recently growing field is that of plasmon-enhanced catalysis, which could be of crucial importance for hydrogen synthesis (Ezendam et al., 2022) with significant consequences for sustainability. Despite its indisputable key role in basic and applied research, the disruptive fallout of Plasmonics in life and society is still mainly restricted to medical diagnostic tools, as demonstrated by the antigenic lateral flow test employed to detect SARS-CoV-2, which is massively employed during this last pandemic. The first clinical pilot study of a device for prostate cancer treatment, carried out by Prof. Halas using photothermal ablation via gold nanoshells (Rastinehad et al., 2019), represents another major landmark in nanomedicine.
The main hindrances to technological application of classical metal-based Plasmonics are the sizeable ohmic losses at visible wavelengths and the non-trivial integration in semiconductor-based technology. With the aim of targeting a higher technological readiness level, and thanks to the recent advancement in nanofabrication, semiconductor-based Plasmonics has rapidly emerged (Taliercio and Biagioni 2019). Indeed, heavily-doped semiconductors display a sizeable plasma frequency, which can be tuned chemically, optically, or electrically over a broad spectral range. These platforms are extremely appealing for their facile integration in low-cost, mass-fabricated devices, but their operation is yet limited to the mid-infrared range.
Yet, Plasmonics endures among the liveliest branches in the field of Photonics, thanks to the extreme light confinement achievable and the ultrafast dynamics of the underlying processes. A major technological task still remains, i.e., the improvement of reliability and reproducibility in plasmonic-based devices and techniques (i.e., plasmon-enhanced Raman spectroscopy, sensing). This will require a major effort in nanofabrication in the coming years to control sub-nm features in metal nanostructures. In this way, unprecedented localization of light will be achieved, enabling the investigation a whole new physics, such as Quantum Plasmonics, as pointed out in the previous Grand Challenge by Prof. Giannini (Giannini 2020). Accessing the quantum regime will be a crucial task in Plasmonics in the coming years. In fact, by concurrently exploiting the ultrafast relaxation dynamics of localized surface plasmons, which are on the timescale of tens of femtoseconds, ultra-high-speed and low-consumption logic operations may be within reach (Rybka et al., 2016; Li et al., 2022) (see top-left panel in Figure 1).
Although single molecule sensitivity has been already reported using plasmonic sensors (Ament et al., 2012; Zijlstra Paulo and Orrit 2012), such challenging task can be facilitated by accessing the quantum regime and, hence, overcoming the fundamental limits imposed by the shot noise (Lee et al., 2021). In plasmonic sensing a crucial role can be also played by the nonlinear optical effects efficiently generated in the strongly localized hot spots of nanoantennas (Mesch et al., 2016). Indeed, along with a nonlinear response of the sensor one should expect a further boost in the sensitivity when resorting to even order nonlinear effects, such as second harmonic generation (Ghirardini et al., 2018), since in metals they stem from the surface where also functional binding is attained (see top-center panel in Figure 1).
As a matter of fact, plasmon-enhanced nonlinear optics deserves a whole separate chapter. In fact, besides the promising applications to sensing, plasmonic metasurfaces still withstand the competition with all-dielectric platforms (Kuznetsov et al., 2016) when coming to nonlinear and active light management in meta-optics. This is possible thanks to the intense light-matter interaction and the facile wavefront control of the generated harmonic signals, which can be shaped by tuning the geometry of the individual plasmonic meta-atoms (Li Zhang and Zentgraf 2017). This entails a whole range of suggestive applications, spanning from nonlinear image encoding and holography (Keren-Zur Avayu et al., 2015; Walter et al., 2017) to frequency up- and down-conversion. In particular, plasmonic metasurfaces were the first to be successfully applied for the generation of terahertz radiation by means of optical rectification (Keren-Zur Tal et al., 2019). Exploiting the strong light confinement in plasmonic nanogaps in hybrid molecular-plasmonic systems, it was also recently demonstrated in a back-to-back publication by Chen et al. (Chen et al., 2021) and Xomalis et al. (Xomalis et al., 2021) that infrared radiation can be detected at room temperature by up-conversion in the near-infrared, where classical detectors can be employed (see top-right panel in Figure 1).
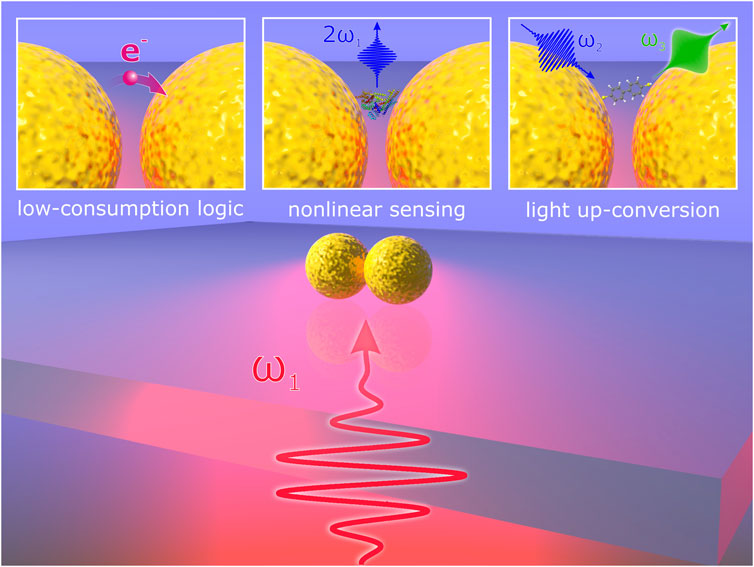
FIGURE 1. Current and future developments in Plasmonics enabled by ultrafast pulsed excitation and sub-nm gaps. Top-left panel: controlled and ultrafast single electron flux for ultrafast low-consumption logic operations. Top-center panel: enhanced nonlinear plasmonic sensing of single proteins and molecules. Top-right panel: light up-conversion by molecular-plasmonic cavity hybrid effect for room-temperature infrared light detection and generation.
In light of these recent achievements and promising new directions, it is clear that the field of Plasmonics is still subject to a sturdy drive and will have a major role in the broader field of Photonics in the coming years if not decades.
Author contributions
The author confirms being the sole contributor of this work and has approved it for publication.
Conflict of interest
The author declares that the research was conducted in the absence of any commercial or financial relationships that could be construed as a potential conflict of interest.
Publisher’s note
All claims expressed in this article are solely those of the authors and do not necessarily represent those of their affiliated organizations, or those of the publisher, the editors and the reviewers. Any product that may be evaluated in this article, or claim that may be made by its manufacturer, is not guaranteed or endorsed by the publisher.
References
Ament, Irene, Prasad, Janak, Henkel, Andreas, Schmachtel, Sebastian, and Sönnichsen, Carsten (2012). Single unlabeled protein detection on individual plasmonic nanoparticles. Nano Lett. 12, 1092–1095. doi:10.1021/nl204496g
Atwater, Harry A., and Albert, Polman. (2010). “Plasmonics for improved photovoltaic devices,” in Materials for sustainable energy (UK: Co-Published with Macmillan Publishers Ltd), 1–11. doi:10.1142/9789814317665_0001
Bocková, Markéta, Slabý, Jiřı́, Špringer, Tomáš, and Homola, Jiřı́ (2019). Advances in surface plasmon resonance imaging and microscopy and their biological applications. Annu. Rev. Anal. Chem. Palo. Alto. Calif. 12, 151–176. doi:10.1146/annurev-anchem-061318-115106
Chen, Wen, Roelli, Philippe, Hu, Huatian, Verlekar, Sachin, Priya Amirtharaj, Sakthi, Barreda, Angela I., et al. (2021). Continuous-wave frequency upconversion with a molecular optomechanical nanocavity. Science 374, 1264–1267. doi:10.1126/science.abk3106
Ding, Song-Yuan, Yi, Jun, Li, Jian-Feng, Ren, Bin, Wu, De-Yin, Panneerselvam, Rajapandiyan, et al. (2016). Nanostructure-based plasmon-enhanced Raman spectroscopy for surface analysis of materials. Nat. Rev. Mater. 1, 16021. doi:10.1038/natrevmats.2016.21
Ezendam, Simone, Herran, Matias, Nan, Lin, Gruber, Christoph, Kang, Yicui, Gröbmeyer, Franz, et al. (2022). Hybrid plasmonic nanomaterials for hydrogen generation and carbon dioxide reduction. ACS Energy Lett. 7, 778–815. doi:10.1021/acsenergylett.1c02241
Ghirardini, Lavinia, Monticelli, Marco, Petti, Daniela, Biagioni, Paolo, Duò, Lamberto, Pellegrini, Giovanni, et al. (2018). Plasmon-enhanced second harmonic sensing. J. Phys. Chem. C 122, 11475–11481. doi:10.1021/acs.jpcc.8b03148
Giannini, Vincenzo (2020). Plasmonics: Future and challenges. Front. Phot. 1, 1–2. doi:10.3389/fphot.2020.621510
Homola, Jiřı́ (2008). Surface plasmon resonance sensors for detection of chemical and biological species. Chem. Rev. 108, 462–493. doi:10.1021/cr068107d
Keren-Zur, Shay, Avayu, Ori, Michaeli, Lior, and Ellenbogen, Tal (2015). Nonlinear beam shaping with plasmonic metasurfaces. ACS Photonics 3, 117–123. doi:10.1021/acsphotonics.5b00528
Keren-Zur, Shay Mai Tal, Fleischer, Sharly, Mittleman, Daniel M., and Ellenbogen, Tal (2019). Generation of spatiotemporally tailored terahertz wavepackets by nonlinear metasurfaces. Nat. Commun. 10, 1778. doi:10.1038/s41467-019-09811-9
Kuznetsov, Arseniy I., Miroshnichenko, Andrey E., Brongersma, Mark L., Kivshar, Yuri S., and Luk'yanchuk, Boris (2016). Optically resonant dielectric nanostructures. Science 354. doi:10.1126/science.aag2472
Lee, Changhyoup, Benjamin, Lawrie, Pooser, Raphael, Lee, Kwang-Geol, Rockstuhl, Carsten, and Tame, Mark (2021). Quantum plasmonic sensors. Chem. Rev. 121, 4743–4804. doi:10.1021/acs.chemrev.0c01028
Li, Guixin, Zhang, Shuang, and Zentgraf, Thomas (2017). Nonlinear photonic metasurfaces. Nat. Rev. Mater. 2, 17010. doi:10.1038/natrevmats.2017.10
Li, Wancong, Zhou, Qiang, Zhang, Pu, and Chen, Xue-Wen (2022). Direct electro plasmonic and optic modulation via a nanoscopic electron reservoir. Phys. Rev. Lett. 128, 217401. doi:10.1103/physrevlett.128.217401
Meola, Antonio, Rao, Jianghong, Chaudhary, Navjot, Sharma, Mayur, and Chang, Steven D. (2018). Gold nanoparticles for brain tumor imaging: A systematic review. Front. Neurol. 9, 328. doi:10.3389/fneur.2018.00328
Mesch, Martin, Metzger, Bernd, Hentschel, Mario, and Giessen, Harald (2016). Nonlinear plasmonic sensing. Nano Lett. 16, 3155–3159. doi:10.1021/acs.nanolett.6b00478
Rastinehad, Ardeshir R., Anastos, Harry, Wajswol, Ethan, Jared, S., Winoker, John P. Sfakianos, Doppalapudi, Sai K., et al. (2019). Gold nanoshell-localized photothermal ablation of prostate tumors in a clinical pilot device study. Proc. Natl. Acad. Sci. U. S. A. 116, 18590–18596. doi:10.1073/pnas.1906929116,
Rybka, Tobias, Ludwig, Markus, Schmalz, Michael F., Knittel, Vanessa, Brida, Daniele, and Leitenstorfer, Alfred (2016). Sub-cycle optical phase control of nanotunnelling in the single-electron regime. Nat. Photonics 10, 667–670. doi:10.1038/nphoton.2016.174
Saha, Krishnendu, Agasti, Sarit S., Kim, Chaekyu, Li, Xiaoning, and Rotello, Vincent M. (2012). Gold nanoparticles in chemical and biological sensing. Chem. Rev. 112, 2739–2779. doi:10.1021/cr2001178
Taliercio, Thierry, and Biagioni, Paolo (2019). Semiconductor infrared plasmonics. Nanophotonics 8, 949–990. doi:10.1515/nanoph-2019-0077
Walter, Felicitas, Li, Guixin, Meier, Cedrik, Zhang, Shuang, and Zentgraf, Thomas (2017). Ultrathin nonlinear metasurface for optical image encoding. Nano Lett. 17, 3171–3175. doi:10.1021/acs.nanolett.7b00676
Wu, Yue, Ali, Moustafa R. K., Chen, Kuangcai, Fang, Ning, and El-Sayed, Mostafa A. (2019). Gold nanoparticles in biological optical imaging. Nano Today 24, 120–140. doi:10.1016/j.nantod.2018.12.006
Xomalis, Angelos, Zheng, Xuezhi, Chikkaraddy, Rohit, Koczor-Benda, Zsuzsanna, Miele, Ermanno, Rosta, Edina, et al. (2021). Detecting mid-infrared light by molecular frequency upconversion in dual-wavelength nanoantennas. Science 374, 1268–1271. doi:10.1126/science.abk2593
Zhang, R., Zhang, Y., Dong, Z. C., Jiang, S., Zhang, C., Chen, L. G., et al. (2013). Chemical mapping of a single molecule by plasmon-enhanced Raman scattering. Nature 498, 82–86. doi:10.1038/nature12151
Keywords: plasmonics, nano-optics, nanoantennas, sensing, biomedicine, nonlinear optics, quantum optics, enhanced Raman scattering
Citation: Celebrano M (2022) Plasmonics: The future is ultrafast and ultrasmall. Front. Photonics 3:969233. doi: 10.3389/fphot.2022.969233
Received: 14 June 2022; Accepted: 18 July 2022;
Published: 17 August 2022.
Edited and reviewed by:
Marco Peccianti, University of Sussex, United KingdomCopyright © 2022 Celebrano. This is an open-access article distributed under the terms of the Creative Commons Attribution License (CC BY). The use, distribution or reproduction in other forums is permitted, provided the original author(s) and the copyright owner(s) are credited and that the original publication in this journal is cited, in accordance with accepted academic practice. No use, distribution or reproduction is permitted which does not comply with these terms.
*Correspondence: Michele Celebrano, bWljaGVsZS5jZWxlYnJhbm9AcG9saW1pLml0