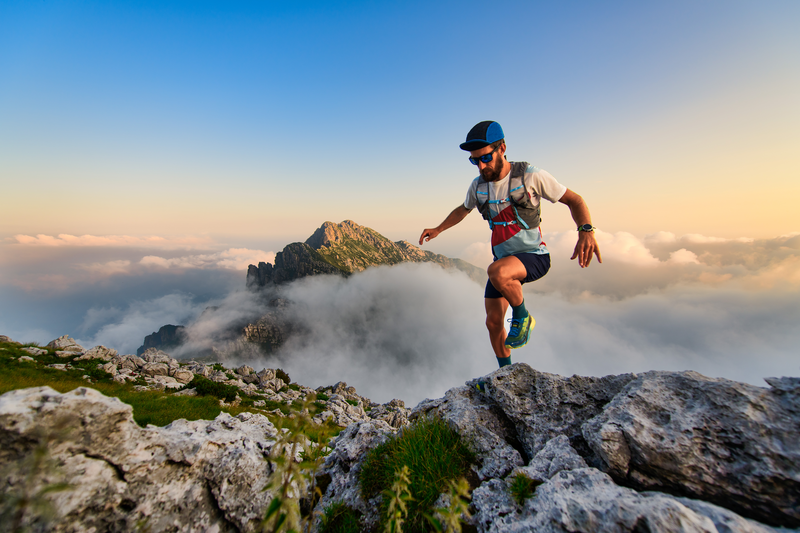
94% of researchers rate our articles as excellent or good
Learn more about the work of our research integrity team to safeguard the quality of each article we publish.
Find out more
REVIEW article
Front. Photonics , 14 October 2022
Sec. Biophotonics
Volume 3 - 2022 | https://doi.org/10.3389/fphot.2022.1015661
This article is part of the Research Topic Translational Clinical Intraluminal Imaging and Optical Sensing View all 5 articles
Cerebral aneurysms are an abnormal ballooning of blood vessels which have the potential to rupture and cause hemorrhagic stroke. The diagnosis, treatment, and monitoring of cerebral aneurysms is highly dependant on high resolution imaging. As an imaging modality capable of cross-sectional resolution down to 10 μm, intraluminal optical coherence tomography (OCT) has great potential in improving care for cerebral aneurysms. The ability to assess the blood vessel microanatomy in vivo may be able to predict aneurysm growth and rupture. During treatment, intraluminal OCT may aid in assessment of treatment efficacy and complication avoidance, such as via visualization of in-stent thrombosis, stent wall apposition, and the fate of covered branch vessels. This technology can also be used in post-treatment monitoring, to assess for aneurysmal remnants or for endothelialisation and healing over the diseased segments. The goal of this clinically focused narrative review is to provide an overview of the previous applications of intraluminal OCT in cerebral aneurysms and future prospects of applying this technology to improve care in patients with cerebral aneurysms, including a specific neurovascular OCT catheter, doppler OCT for high resolution blood flow assessment, and further research endeavors.
Rupture of cerebral aneurysms can lead to hemorrhagic stroke and significant morbidity and/or mortality (Macdonald and Schweizer, 2017). These vascular abnormalities are found in approximately 3.2% of the population (Vlak et al., 2011). Once found, the risk of rupture based on factors such as aneurysm size, morphology, and wall structure, in relation to patient age and comorbiditie,s can be calculated and weighed against the risks of treatment (Vlak et al., 2011; Macdonald and Schweizer, 2017; Zhao et al., 2018). If treatment is indicated, the current gold standard is endovascular coiling, which has been shown to lead to lower rates of morbidity and mortality, albeit with higher aneurysm recurrence rates, compared to microsurgical clipping (Molyneux et al., 2015; Thompson et al., 2015).
During coiling, following vascular access, catheters are navigated to the aneurysm, where detachable metallic coils are placed inside, which promote thrombosis within the aneurysm. Aneurysmal thrombosis leads to obliteration of blood flow into the aneurysm, removing the risk of rupture. Endothelialisation then occurs over the neck of the aneurysm, leading to restoration of the normal cerebral anatomy. As an adjunct to coiling, or as a stand-alone endovascular treatment, stenting with a metallic mesh tube over the neck of the aneurysm can also be performed (Thompson et al., 2015; Zhao et al., 2018). Specifically, flow-diverting stents, which have a higher surface area coverage and lower porosity compared to traditional stents, can be utilized to divert blood flow away from the aneurysm to also promote intra-aneurysmal thrombosis. The recurrence rate of aneurysms following endovascular treatment can be up to 20%, with a number of these patients requiring re-treatment (Raymond et al., 2003; Molyneux et al., 2015; Thompson et al., 2015; Zhou et al., 2017). The diagnosis, monitoring, treatment, and monitoring of cerebral aneurysms is highly dependant on high resolution imaging. Current imaging modalities utilized include catheter angiography, CT and CT angiography, and MRI and MR angiography. As an imaging modality that has a spatial resolution of 10 microns, the highest resolution currently available (Tearney et al., 1979; Pasarikovski et al., 2020a; Anagnostakou et al., 2021), intraluminal optical coherence tomography (OCT) has great potential in improving care for cerebral aneurysms. First developed in 1991, OCT is a light-based imaging modality that utilizes low-coherence interferometry to produce images based on the differential light reflection or backscattering from biological tissue (Huang et al., 1979). OCT at times has been described as an “optical biopsy,” due to the capability to provide microanatomical details of the imaged tissue without the requirement for tissue excision (Tearney et al., 1979). This technology was first applied to the retinal surface and inside coronary vessels in 1991 (Huang et al., 1979), and subsequently the Food and Drug Administration (FDA) approved the use of intraluminal OCT for the diagnosis and treatment of cardiovascular disease in 2010 (Tearney et al., 2012). In the cardiovascular environment, this technology has allowed for the high-resolution imaging of atherosclerotic plaques or thrombus, vessel wall imaging, stent evaluation, and detailed assessment of coronary aneurysms (Tearney et al., 2012; Dionne et al., 2018; Shimamura et al., 2021). It is unparalleled ability to visualize the intraluminal environment and blood vessel microanatomy on a near histological level has led to widespread adoption in the field of interventional cardiology (Tearney et al., 2012; Yonetsu et al., 2013).
As such, the utility of intraluminal OCT has also garnered clinical and research interest in the field of interventional neuroradiology. Similar to interventional cardiology, applications of intraluminal OCT in the cerebrovasculature can include assessment of atherosclerosis, aneurysms, stenting, or following thrombectomy (Pasarikovski et al., 2020a; Anagnostakou et al., 2021). As a disease that leads to significant morbidity and mortality, cerebral aneurysms as a disease deserves specific attention. The goal of this narrative review was to assess the previous uses of intraluminal OCT in cerebral aneurysms, with a view towards future progress. This review will discuss the device description and the technical aspects of image acquisition, and then the different aspects of aneurysm care where intraluminal OCT may be enlightening. This includes imaging of the vessel wall to better understand the aneurysm and risk of rupture, imaging during aneurysm treatment to prevent adverse outcomes, or imaging during aneurysm follow-up to guide further therapies.
Currently, there is no intraluminal OCT device approved for use in the cerebrovasculature, and the commercially available coronary intraluminal OCT devices are utilized “off-label” for clinical or research purposes (Table 1). There are several intraluminal OCT catheters to choose from, including the Dragonfly (St. Jude Medical, Minneapolis, MN), Novasight Hybrid System Fastview catheter with the Lunawave system (Terumo Corporation, Tokyo, JP), and the Vis-Rx Micro-Imaging Catheter with the Gentuity HF-OCT Imaging System (Gentuity LCC, Sudbury, MA).
At our institution, we utilize the Dragonfly catheter with the ILUMIEN OPTIS system (St. Jude Medical, Minneapolis, MN). The Dragonfly is a 2.7 French OCT imaging catheter that can be navigated to the region of interest co-axially through an access catheter or in monorail fashion over a 0.014" microwire. Radio-opaque markers on the catheter allow for confirmation of positioning using fluoroscopy. There is one marker that indicates the distal tip and another 2.3 cm proximally that indicates the starting position of imaging lens. There is a third marker 5.0 cm proximal to the imaging lens marker, and OCT images are acquired between the second and third radio-opaque markers. For image acquisition, the OCT catheter is placed within the parent vessel with the aneurysm between the second and third markers. A schematic of the catheter tip and example image is shown in Figure 1.
FIGURE 1. (A) Schematic of the Optical Coherence Tomography Catheter Tip, showing the optical element and lens to redirect the light radially outward, with the torque coil providing rotation allowing for the generation of cross sectional images of the artery wall. (B) An OCT image of a coronary artery, showing the catheter, wire and wire artifact (*), and layers of the blood vessel wall including Internal Elastic Lamina (IEL) and External Elastic Lamina (EEL). Scale bar = 1 mm. Reprinted with permission (Ughi and Adriaenssens, 2017).
As blood is a scattering medium in the near infra-red range that OCT utilizes, it must be displaced from the region of interest to capture an intraluminal OCT image. This can be performed with heparinized saline, contrast solution, or a mixture of both, and can be done via hand injection or auto-injection. However, this represents a limitation for intraluminal OCT in aneurysms. If blood is not fully cleared within the aneurysm, the portion of the aneurysm past the blood will be obscured. This may occur as flushing from the parent vessel may not be sufficient to expel the pooled blood from the aneurysm. On the other hand, excessive flushing may also increase the risk of intra-procedural rupture, as instances of aneurysmal rupture during contrast injection during catheter angiography have been previously reported (Klisch et al., 2003). Contrast or fluid overload with repeated flushing may also be an issue, leading to cardiorespiratory or renal issues (Tavakol et al., 2012; Rear et al., 2016). Ideally, OCT image acquisition should occur simultaneously during angiography image acquisition when a bolus of contrast is to be given, to decrease the amount of flushing required.
The catheter automatically detects blood clearance from the lumen and begins the automated motorized pullback (between the second and third radio-opaque markers) to capture OCT images at 100 frames per second, leading to a total of 540 cross-sectional images with a spatial resolution of 10–15 microns and a vessel wall depth of penetration of 3 mm. Image acquisition can be repeated multiple times, as the catheter itself maintains its position during this process. The field of view is 10 mm from the catheter’s lateral surface. This does represent a limitation in the application of intraluminal OCT for cerebral aneurysms, as the dome of aneurysms may not be seen. Additionally, the blood vessels of the intracranial vessels are also wider than the coronary vessels where intraluminal OCT is usually deployed. On the other hand, the neck of the aneurysm can be well-visualized, and this is where high resolution imaging may aide in assessing treatment success and complications.
In terms of safety and feasibility, intraluminal OCT has been performed successfully in 3D printed models, cadavers, animals, and humans (Table 2). The posterior circulation is more straightforward to navigate compared to the anterior circulation, mostly due to the tortuosity present in the carotid siphon. Intraluminal OCT catheter navigation for vertebral or basilar arteries in humans have been performed successfully without complication in 3 cases (Pasarikovski et al., 2020b; Li et al., 2022). For the anterior circulation, Lopes et al. first demonstrated the ability to navigate the OCT catheter past the internal carotid artery and imaged the middle and posterior cerebral arteries in a fresh frozen cadaver in 2012, visualizing important small perforating arteries which are not well appreciated on catheter angiography (Lopes and Johnson, 2012). In patients, there has been one reported case of an inability to negotiate the carotid siphon, leading to transient vasospasm and incomplete imaging of a deployed flow-diverting stent (Griessenauer et al., 2017). Overall, there have been no reported cases of neurologic complications following intraluminal OCT catheter navigation and image acquisition in twelve patients with intracerebral aneurysms (Table 2). However, as the use of OCT continues to expand, continued complication monitoring will be paramount, as it is often compared to non-invasive imaging modalities with minimal risk. Intraluminal OCT does require arterial access and intravascular catheter navigation to the aneurysm; therefore, it should only be done during times when cerebral angiograms are to be performed. It should be viewed as an adjunct to angiography to allow for high resolution image acquisition.
Notably, OCT can be compared to intravascular ultrasound (IVUS), as another intraluminal methodology of image acquisition. One key difference between the two are that OCT has 10x the resolution of IVUS but more limited tissue penetration (3 mm for OCT vs. up to 10 mm for IVUS, which decreases for frequencies greater than approximately 25 MHz), especially in the presence of red blood cells, red thrombus, lipids, or necrosis (Maehara et al., 2017; Nagaraja et al., 2020). OCT also requires blood clearance whereas IVUS does not, and IVUS has a longer image acquisition time than OCT (Maehara et al., 2017; Nagaraja et al., 2020). In the field of interventional cardiology, where both have been well studied, clinical circumstances where one is better than the other have been established (Tearney et al., 2012; Maehara et al., 2017; Nagaraja et al., 2020). On the other hand, IVUS experience in neuro-intervention (Macdonald and Schweizer, 2017) These vascular abnormalities are foervention and outside the scope of this review article. Intraluminal OCT for aneurysms may have more utility given its ability to obtain higher resolution imaging, but further studies are needed.
Cerebral aneurysms are thought to arise from congenital or acquired defects of the internal elastic lamina (IEL) or media, with further remodelling leading to the characteristic ballooning of the blood vessel wall (Frösen et al., 2019; Staarmann et al., 2019). These changes most commonly occur at bifurcation sites, where the blood flow is more turbulent and shear forces against the arterial wall are higher (Frösen et al., 2019). As such, it follows that the ability to assess the blood vessel microanatomy in vivo via intraluminal OCT may be able to predict aneurysm formation, growth, and rupture.
In 2011, Mathews et al. performed in vivo intraluminal OCT imaging of the internal carotid arteries in two pigs and in three patients with previously coiled cerebral aneurysms (Mathews et al., 2011). The blood vessel microanatomy, including the intima, internal elastic lamina (IEL), media, external elastic lamina (EEL), and adventitia, was well visualized and correlated with histological findings (Mathews et al., 2011). This has also been investigated with imaging of silicone phantom models based on angiographic images of patients’ aneurysms (Hoffmann et al., 2016). Hoffman et al. found the ability to visualize intimal thickening, dissections, and perforating arteries, as well as macro-anatomical details such as aneurysmal geometry and dimensions (Figure 2) (Hoffmann et al., 2016). However, they were unable to fully image large aneurysms due to the OCT imaging diameter of 10 mm, nor bottleneck aneurysms due to the overlapping tissue of the parent vessel and the aneurysm (Hoffmann et al., 2016). Additionally, as the saline/contrast flush is utilized for intraluminal clearing of the parent vessel, it is possible that blood is not fully cleared from the aneurysm to allow for image acquisition. Conversely, flushing may also increase the risk of intra-procedural rupture, as instances of aneurysmal rupture during contrast injection during catheter angiography have been previously reported (Klisch et al., 2003).
FIGURE 2. Schematic of Intraluminal OCT acquisition for aneurysms, with the catheter positioned in the parent vessel and the pullback length encompassing the area to be imaged. The corresponding cross-sectional image is illustrated as A-A. Reprinted with permission (Hoffmann et al., 2016).
From an extra-vascular perspective, OCT has been previously utilized to assess the microstructural composition of aneurysms in 16 patients undergoing microsurgical clipping, allowing for assessment of characteristics including wall thickness, calcification, residual tunica media, and atherosclerosis (Hartmann et al., 2019). However, these findings may be difficult to replicate from an intraluminal catheter, due to the imaging limitations of the catheter from the parent vessel. Navigation of the catheter into the aneurysm itself is likely dangerous due to the stiffness of the catheter and the length of catheter before the imaging lens.
Although an estimated 3.2% of people harbor intracranial aneurysms, not all aneurysms require treatment once discovered (Vlak et al., 2011). Treatment is only undertaken when it is felt that the risk of rupture outweigh the risks associated with treatment, but the prediction of rupture risk remains an inexact science. Rupture risk reflects a complex interplay of aneurysm factors (size, morphology, location, flow dynamics), patient factors (age, sex, comorbidities, genetics), and beyond (Cebral et al., 2011; Lv et al., 2019). Imaging findings including vessel wall enhancement on MRI (Lv et al., 2019; Staarmann et al., 2019) and computational flow modelling on CT or diagnostic angiogram (Cebral et al., 2011; Ishida et al., 2021) are recent advances in the field of aneurysmal rupture prediction. As suggested above, there is also potential in the use of intraluminal OCT in this realm, however the exact role has yet to be elucidated, and there is a lack of current studies assessing this. Further prospective research is required to determine whether OCT findings can be correlated with aneurysmal growth and rupture.
In addition, intraluminal OCT may also be utilized to further our understanding of the pathophysiology behind aneurysms without necessitating ex-vivo histological analysis. Griessenauer et al. imaged two traumatic aneurysms of the internal carotid artery and visualized disruption of the intima with preservation of the other layers, as well as thrombus formation and an intimal dissection not seen on standard angiography (Griessenauer et al., 2016). Li et al. imaged a dissecting aneurysm of the vertebral artery, albeit only following stent assisted coiling, so disease patho-anatomical details were obscured (Li et al., 2022). Additional intraluminal OCT imaging of non-saccular aneurysms, such as dissecting, fusiform, or blister aneurysms, may aid further understanding about these pathologies which commonly have worse outcomes and/or are more difficult to treat compared to saccular aneurysms (Barletta et al., 2019; Cagnazzo et al., 2020).
During aneurysm treatment, intraluminal OCT may be utilized to assess placement of stents and/or coils and the immediate effects of this. In 2012, Lopes et al. first demonstrated this by deploying a Pipeline Embolization Device (PED) on the basilar artery and utilized intraluminal OCT to visualize the relationship of the stent to the vessel wall, including strut apposition, and the branching vessels with stent struts across these orifices (Lopes and Johnson, 2012). Further work has been done in animal models to further assess this and infer the feasibility and utility of intraluminal OCT during aneurysm treatment, specifically immediately following stent deployment. In canine side-wall aneurysms, intraluminal OCT was able to quantify stent wall apposition as ‘device-wall distance’ with automatic detection of distance beyond 0.25 mm to facilitate screening for potential malapposition and acute thrombus formation (van der Marel et al., 2016). Thrombus formation is a rare but feared complication following stent deployment and can lead to acute ischemia or chronic in-stent stenosis (Zhou et al., 2017). If seen, it is commonly treated with intra-arterial or intravenous antithrombotic therapy (Zhou et al., 2017; Lin et al., 2018). In a swine model where flow diverting stents were deployed to cover a branch vessel of the internal carotid artery, intraluminal OCT allowed for the evaluation of acute thrombus formation on the stent struts and inside the jailed artery, as well as verification of lysis of thrombus following administration of antithrombotics (Iosif et al., 2016). The ability of OCT to detect and quantify stent-related thrombus has also been utilized in a preclinical environment to assess different types of stent coatings and antithrombotic regimens to reduce acute thrombus formation (Marosfoi et al., 2018; King et al., 2020), as seen in Figure 3.
FIGURE 3. Intraluminal OCT of different stent devices under different antiplatelet regimens (A–F), showing the ability to assess for clot formation (white arrow) and incomplete blood clearance (white *). Scale bar = 1 mm. Reprinted with permission (King et al., 2020).
In addition, stent strut apposition to the parent vessel wall can also be assessed with intraluminal OCT immediately following stent deployment, as seen in Figure 4 (Anagnostakou et al., 2021). Good wall apposition has been strongly associated with complete occlusion after flow-diverter therapy, but catheter angiography has been reported as suboptimal for the assessment of this (Rouchaud et al., 2016). With intraluminal OCT, poor apposition can be visualized and immediately corrected with balloon inflation (Iosif et al., 2016). King et al. placed flow diverting stents with balloon angioplasty in 40 aneurysms in rabbits and obtained OCT images prior to and following angioplasty, and found that malapposition of the flow diverter at the neck of the aneurysm which was present after angioplasty was associated with aneurysmal persistence at 30 days (King et al., 2018). They did report one case of malapposition caused by the balloon angioplasty leading to poor aneurysm occlusion, and posited that ubiquitous balloon angioplasty may actually have a negative effect on stent apposition, and thus intraluminal high resolution imaging may aid in the decision-making process following stent deployment (King et al., 2018).
FIGURE 4. High resolution CT imaging showing good flow diverting stent wall apposition (A), but intraluminal OCT (B,C) of the same stent revealing areas of poor wall apposition (white arrow). Scale bar = 1 mm. Reprinted with permission (Anagnostakou et al., 2021).
The ability of intraluminal OCT to detect and treat instances of thrombus formation and/or stent malapposition, which could otherwise lead to adverse outcomes, has also been explored in several human studies. Martínez-Galdámez et al. imaged a Pipeline Shield stent deployed to treat an unruptured posterior communicating artery aneurysm, and found areas of malapposition, particularly at the paraclinioid section of the ICA, which was not seen on catheter angiography, without evidence of acute thrombus (Martínez-Galdámez et al., 2019). However, as there was already contrast stasis within the aneurysm following deployment, a predictive marker for successful aneurysm occlusion (O’Kelly et al., 2010), balloon angioplasty was not performed (Martínez-Galdámez et al., 2019). Li et al. imaged two cases of stent-assisted coiling in vertebral artery aneurysms, in which Enterprise and Enterprise two stents were used, which have a lower metal coverage and higher porosity compared to flow diverting stents. They found that OCT was able to demonstrate good apposition of the stent struts and visualized loops of coils at the neck of the aneurysm without herniation into the parent vessel (Li et al., 2022). Notably, neither article reported any findings on OCT that required additional clinical action.
Although most of the research interest has been in imaging of flow diverting stents, intraluminal OCT is also possible for traditional stent assisted coiling, as demonstrated by Li et al. (Li et al., 2022), or even in cases of simple coiling. Theoretically, OCT could also be utilized to demonstrate the relationship of coil loops at the neck of the aneurysm, and possibly visualize any coil loop herniations into the parent artery not visible on angiography but may still have potential to cause distal embolization events (Luo et al., 2008).
Additionally, there is the potential to discover new predictors of aneurysm occlusion following coiling with better visualization of the relationship between the coil loops and the parent vessel, in addition to traditionally assessed markers such as packing volume or density (Kawanabe et al., 2001; Sluzewski et al., 2004). The Woven Endobridge (WEB) is another type of machinal detachable device which has been recently developed for the treatment for cerebral aneurysms, especially wide-necked aneurysms at arterial bifurcations (Harker et al., 2021; Mouchtouris et al., 2022). Intraluminal OCT has been applied to assess adequate WEB deployment within the aneurysm (no protrusion, good apposition), and has been shown to predict aneurysmal occlusion at 12 weeks follow-up in a rabbit model (Vardar et al., 2021). Overall, the expansion of applying intraluminal OCT into the assessment of aneurysmal treatments other than flow diversion is a gap in the literature that may be expanded upon.
Intraluminal OCT can also be used in post-treatment monitoring, in the assessment for aneurysmal remnants, for the fate of branching vessels, or for endothelialisation over the diseased segments or deployed stents. Iosif et al. covered branching arteries with a flow-diverting stent in four swine at 12 weeks, and readily visualized neointimal formation over stent struts and postulated that OCT may be utilized to assess when full coverage is achieved to guide antiplatelet regimen management (Iosif et al., 2016). The ability to analyze endothelialisation over the stent as well as over the ostium of covered vessels has also allowed for the use of OCT in comparing the effect of different antiplatelet regimens or different stent types on neointimal formation (Matsuda et al., 2019; Caroff et al., 2020). Intraluminal OCT application has also led to discovery that flow diversion may induce a drastic reduction of the covered branch ostium area, despite continued patency on catheter angiography (Caroff et al., 2020). Branching vessels covered by flow diverting stents may not always remain patent (Zhou et al., 2017), and improving our understanding about how and why this occurs can help with complication avoidance. Our own group recently performed OCT imaging on aneurysms in rabbits treated with flow diversion at 4 weeks, and again found the ability to characterize endothelialisation across the stent and patent areas across the neck of the aneurysm, with minimal thrombus formation within the aneurysm itself (Pasarikovski et al., 2021). This work shows that OCT can also improve our knowledge of the mechanisms behind aneurysmal healing following flow diversion. Additionally, intraluminal OCT has also been utilized to image in-stent thrombosis in an animal model, showing neointima on top of asymmetrical topography covering the stents, likely representing thrombus presence under the endothelization, furthering our understanding behind the causes of in-stent thrombosis (Monteiro et al., 2021).
Comparison of catheter angiography versus OCT in detecting residual aneurysms following flow diversion have also been performed in animal models. Fries et al. found that residual aneurysms at 28 days were significantly more often likely to be visible with OCT (78%) than with DSA (52%) after flow diversion in an animal model (Fries et al., 2020). However, as not all residual or recurrent aneurysmal remnants need to be treated (Madaelil et al., 2019), caution should be practiced when utilizing OCT to detect very small remnants and basing practice decisions on these particular OCT findings.
Studies in humans have also found that intraluminal OCT could also reliably visualize and measure endothelialisation on flow diverting stents, which can be utilized to assess aneurysmal healing and the need for continued antiplatelets to prevent thrombus formation. Griessenauer et al. found variability along the length of a long-standing implanted PED for an ICA aneurysm, with areas of bare stent, thin endothelium, or robust neointima (Griessenauer et al., 2017). Guerrero et al. reported one case flow diversion of a previously coiled PICA aneurysm, with OCT at 8 weeks demonstrating good wall apposition and concentric neointimal growth (Guerrero et al., 2018). In two stent assisted coiling cases, Li et al. also found variability in endothelial formation at 6 months, with one case of complete coverage by neointima and another with many struts prolapsed into the lumen and nonadherent to the vessel wall (Figure 5) (Li et al., 2022). They utilized these findings to guide management, as clopidogrel administration was stopped in the patient with a fully endothelialised stent (minimal risk of thrombus formation), while dual antiplatelet therapy was continued in the patient with a partially endothelialised stent (continued risk of thrombus formation) (Li et al., 2022). Yako et al. also performed intraluminal OCT in two stent-assisted coiling cases, at 1- and 2-year follow-up. In one case there was complete neointimal coverage of the stents over the aneurysmal neck in one case, and in the other there was only partial coverage of the stent struts with an irregular, thrombus-like matrix covering the coils loops at the aneurysmal neck (Yako et al., 2017). In the latter case, continued antiplatelet therapy and close follow-up to monitor for aneurysmal recurrence was performed based on the OCT findings (Yako et al., 2017). The length of antiplatelet therapy and choice of agents following flow diversion remains a contentious topic (Madaelil et al., 2019; Cagnazzo et al., 2020; Tonetti et al., 2020), and OCT may provide further insights on guiding antiplatelet therapy based on individualized high resolution imaging findings.
FIGURE 5. Cerebral angiography (A,B) showing the dissecting aneurysm (white arrows), with intraluminal OCT (C,D). The aneurysm (white arrow, white asterisk representing the far wall) is only partially imaged due to the limitations of OCT (yellow arrows). Following stent assisted coiling, angiography (E,F) shows obliteration of the aneurysm, and intraluminal OCT (G,H) demonstrates coil loops within the aneurysm and some areas of stent malapposition (white arrows). Scale bar = 1 mm. Reprinted with permission (Li et al., 2022).
The reasons behind aneurysmal persistence or recurrence following treatment may also be explored with intraluminal OCT, especially when traditional imaging techniques, including catheter angiography, fail to elucidate the issue. Neira et al. reported the use of OCT in a recanalized ICA aneurysm treated by stent assisted coiling to reveal malapposition of the stent and lack of neointimal growth at the aneurysm neck (Neira et al., 2021). This was then treated with an overlapping PED, with 1 year angiogram demonstrating aneurysmal healing and OCT good stent apposition and total endothelialisation over the aneurysm neck (Neira et al., 2021). Our own center has utilized intraluminal OCT similarly, in a case of a posterior communicating artery aneurysm which increased in size after 2 months despite flow diverting stent assisted coiling. Although there was no evidence of stent malapposition with high-resolution cone-beam (VASO) CT, OCT imaging demonstrated no stent endothelialisation over a patent portion of the aneurysm neck. This was treated with a second stent, leading to complete aneurysmal occlusion (Pasarikovski et al., 2020b). Although repeat flow diversion is generally felt to be safe and effective (Salem et al., 2022), there can still be associated complications, such as in-stent stenosis or closure of jailed branch vessels due to the increased metal coverage, or other risks associated with a repeat endovascular procedure. As such, intraluminal OCT may provide additional details about the microvascular environment to help guide additional therapies for aneurysmal recurrence or residual.
Clearly, intraluminal OCT has great potential in improving care for patients with cerebral aneurysms. The ability to provide high resolution micro-anatomical imaging of blood vessels and vascular abnormalities can add to our understanding of aneurysms, and possibly monitor or predict aneurysmal growth or rupture. During endovascular procedures, OCT can be utilized to assess the efficacy of treatment, providing clinicians with additional information that may guide the need for additional management. This could include the visualization of malapposition leading to balloon angioplasty or findings of acute thrombus requiring antithrombotic administration, findings which may not be readily apparent on catheter angiography. During follow-up, intraluminal OCT assessment of the endothelial healing over the neck of the aneurysm and/or the parent vessel may help guide antiplatelet regimen management or the necessity for closer follow-up. The reasons behind aneurysmal persistence or recurrence following treatment may also be explored with OCT, leading to individualized treatment plans based on micro- and macro-anatomical details not obtainable with other imaging modalities. In a preclinical realm, OCT can also be utilized as an assessment tool for the efficacy of aneurysm treatment, including different stent types, antiplatelet regimens, or in the development of novel devices. Areas for future direction include a neurovascular specific OCT catheter, doppler OCT, and additional clinical research, amongst others (Table 3).
One important future direction would be the continued development of a neurovascular specific OCT catheter. Improvements on currently available devices include better flexibility and navigability into the more tortuous cerebrovasculature (especially the anterior circulation) and a wider field of view to image larger vessels and aneurysms (Anagnostakou et al., 2021). Such a device is currently under development with promising results showing good maneuverability and the capability to rapidly acquire volumetric microscopy data at a 10-micron resolution level in swine vessels with and without deployed stents and in ex-vivo human atherosclerotic vessels (Ughi et al., 2020). Another area for catheter improvement could be a shorter “leading tip”. Currently, entry of the aneurysm itself with the OCT catheter is not possible due to the long length between the catheter tip and the imaging length, usually larger than the aneurysm size itself. A shorter “leading tip” could allow for placement of the OCT catheter within the aneurysm to better assess the wall structure of the aneurysm, though this must be weighed with the risks of aneurysm catheterization (e.g., rupture). Other areas for future development could include more rapid image acquisition, and improved mechanisms for blood clearing/image acquisition. Even small amounts of residual blood can adversely affect intraluminal OCT image acquisition, especially in situations where there may be flow stasis within the aneurysm, leading to incomplete visualization. Improved mechanisms for blood clearing or image acquisition, without the need to aggressively flush blood from the target vasculature, would be most welcome.
Another area for further advancement could be the development of doppler OCT, to assess blood flow velocity and provide volumetric flow measurements (Sun et al., 2012; Vuong et al., 2014). For example, we have previously utilized doppler OCT to assess a novel liquid embolic agent treatment in a phantom model of a cerebral aneurysm, showing changes in flow following obliteration of the aneurysm (Figure 6) (Dobashi et al., 2021). Hemodynamic patterns and flow patterns into a cerebral aneurysm have implicated to be predictive of aneurysm growth and rupture risk (Cebral et al., 2011; Ishida et al., 2021), and contrast stasis (or lack of flow into the aneurysm) immediately following treatment has been found to be predictive of aneurysm healing (O’Kelly et al., 2010). Intraluminal doppler OCT may be able to provide more detailed hemodynamic information regarding aneurysms and aneurysm treatment, however, this technology must first be validated against other flow-measuring modalities and explored further in phantom and animal models. Additionally, one significant barrier to doppler OCT is nonuniform rotational distortion (NURD), the distortion of the cross-sectional images as the transducer rotates in a nonuniform manner despite being coupled to a uniformly rotating motor, due to tortuosity in the blood vessels or friction with the catheter sheathe (Kawase et al., 2007; Ahsen et al., 2014). This can lead to artefact in the acquired images. Imaging processing techniques have allowed for minimization of NURD with intraluminal OCT (Kawase et al., 2007; Ahsen et al., 2014), however, techniques for NURD shift induced phase shift artifact removal for doppler OCT remain limited (Sun et al., 2012).
FIGURE 6. Doppler intraluminal OCT in a model aneurysm, with pre-treatment imaging in the systolic phase (A) and diastolic phase (B) showing blood flow in the parent artery (white arrowhead) and into the aneurysm (green arrowhead). Following embolization with a novel liquid embolic agent, intraluminal doppler OCT in the systolic phase (C) and diastolic phase (D) shows continued flow in the parent artery (white arrowhead) but complete cessation of flow into the aneurysm (green arrowhead). Scale bar = 1 mm. Reprinted with permission (Dobashi et al., 2021).
Lastly, much additional research is required in this fledgling field. In the realm of interventional cardiology, intraluminal OCT has become widely adopted clinically (Tearney et al., 2012; Dionne et al., 2018; Shimamura et al., 2021), and from a research perspective, there have already been multiple randomized controlled trials showing OCT efficacy in guiding management leading to improved clinical outcomes (Burzotta et al., 2014; Meneveau et al., 2016; Lee et al., 2020). Conversely, reports of intraluminal OCT use in human cerebral aneurysms are limited to case reports and case series. Nevertheless, the potential of OCT in improving aneurysm care has been demonstrated and should be continued to be explored.
JK and VY conceived and designed the article. JK, CP, YD, and JR collected and analyzed the data. JK and CP interpreted the data. JK prepared the draft manuscript with support from CP and YD. All authors critically revised the work and approved the final version for submission for publication.
The authors declare that the research was conducted in the absence of any commercial or financial relationships that could be construed as a potential conflict of interest.
All claims expressed in this article are solely those of the authors and do not necessarily represent those of their affiliated organizations, or those of the publisher, the editors and the reviewers. Any product that may be evaluated in this article, or claim that may be made by its manufacturer, is not guaranteed or endorsed by the publisher.
Ahsen, O. O., Lee, H. C., Giacomelli, M. G., Wang, Z., Liang, K., Tsai, T. H., et al. (2014). Correction of rotational distortion for catheter-based en face OCT and OCT angiography. Opt. Lett. 39 (20), 5973. doi:10.1364/OL.39.005973
Anagnostakou, V., Ughi, G. J., Puri, A. S., and Gounis, M. J. (2021). Optical coherence tomography for neurovascular disorders. Neuroscience 474, 134–144. doi:10.1016/j.neuroscience.2021.06.008
Barletta, E., Gaspar, R. M. L., Araújo, J. M., Neves, M. F., Aquino, J. B. de, and Belsuzarri, T. B. (2019). Nonsaccular aneurysms: A wide comparison between the four main types. Surg. Neurol. Int. 10 (1), 30. doi:10.4103/sni.sni_138_18
Burzotta, F., Leone, A. M., de Maria, G. L., Niccoli, G., Coluccia, V., Pirozzolo, G., et al. (2014). Fractional flow reserve or optical coherence tomography guidance to revascularize intermediate coronary stenosis using angioplasty (FORZA) trial: Study protocol for a randomized controlled trial. Trials 15 (1), 140. doi:10.1186/1745-6215-15-140
Cagnazzo, F., Lefevre, P. H., Derraz, I., Dargazanli, C., Gascou, G., di Carlo, D., et al. (2020). Flow-diversion treatment for unruptured nonsaccular intracranial aneurysms of the posterior and distal anterior circulation: A meta-analysis. AJNR. Am. J. Neuroradiol. 41 (1), 134–139. doi:10.3174/ajnr.A6352
Caroff, J., King, R. M., Ughi, G. J., Marosfoi, M., Langan, E. T., Raskett, C., et al. (2020). Longitudinal monitoring of flow-diverting stent tissue coverage after implant in a bifurcation model using neurovascular high-frequency optical coherence tomography. Neurosurg. 87 (6), 1311–1319. doi:10.1093/neuros/nyaa208
Cebral, J. R., Mut, F., Weir, J., and Putman, C. M. (2011). Association of hemodynamic characteristics and cerebral aneurysm rupture. AJNR. Am. J. Neuroradiol. 32 (2), 264–270. doi:10.3174/ajnr.A2274
Dionne, A., Ibrahim, R., Gebhard, C., Benovoy, M., Leye, M., Dery, J., et al. (2018). Difference between persistent aneurysm, regressed aneurysm, and coronary dilation in kawasaki disease: An optical coherence tomography study. Can. J. Cardiol. 34 (9), 1120–1128. doi:10.1016/j.cjca.2018.05.021
Dobashi, Y., Ku, J. C., Pasarikovski, C., Ramjist, J., Madden, J. D. W., Walus, K., et al. (2021). Dynamically tunable intravascular catheter delivery of hydrogels for endovascular embolization. MRS Adv. 6 (3), 66–71. doi:10.1557/s43580-021-00047-8
Fries, F., Maßmann, A., Tomori, T., Yilmaz, U., Kettner, M., Simgen, A., et al. (2020). Accuracy of optical coherence tomography imaging in assessing aneurysmal remnants after flow diversion. J. Neurointerv. Surg. 2, 1242–1246. neurintsurg-2020-016129. doi:10.1136/neurintsurg-2020-016129
Frösen, J., Cebral, J., Robertson, A. M., and Aoki, T. (2019). Flow-induced, inflammation-mediated arterial wall remodeling in the formation and progression of intracranial aneurysms. Neurosurg. Focus 47 (1), E21. doi:10.3171/2019.5.FOCUS19234
Griessenauer, C. J., Foreman, P. M., Deveikis, J. P., and Harrigan, M. R. (2016). Optical coherence tomography of traumatic aneurysms of the internal carotid artery: Report of 2 cases. J. Neurosurg. 124 (2), 305–309. doi:10.3171/2015.1.JNS142840
Griessenauer, C. J., Gupta, R., Shi, S., Alturki, A., Motiei-Langroudi, R., Adeeb, N., et al. (2017). Collar sign in incompletely occluded aneurysms after pipeline embolization: Evaluation with angiography and optical coherence tomography. AJNR. Am. J. Neuroradiol. 38 (2), 323–326. doi:10.3174/ajnr.A5010
Guerrero, B. P., Pacheco, C. D., Saied, A., Joshi, K., Rodriguez, C., Martinez-Galdamez, M., et al. (2018). First human evaluation of endothelial healing after a pipeline flex embolization device with Shield technology implanted in posterior circulation using optical coherence tomography. Neurointervention 13 (2), 129–132. doi:10.5469/neuroint.2018.01032
Harker, P., Regenhardt, R. W., Alotaibi, N. M., Vranic, J., Robertson, F. C., Dmytriw, A. A., et al. (2021). The woven EndoBridge device for ruptured intracranial aneurysms: International multicenter experience and updated meta-analysis. Neuroradiology 63 (11), 1891–1899. doi:10.1007/s00234-021-02727-6
Hartmann, K., Stein, K. P., Neyazi, B., and Sandalcioglu, I. E. (2019). Aneurysm architecture: First in vivo imaging of human cerebral aneurysms with extravascular optical coherence tomography. Cerebrovasc. Dis. 48 (1-2), 26–31. doi:10.1159/000502450
Hoffmann, T., Glaßer, S., Boese, A., Brandstadter, K., Kalinski, T., Beuing, O., et al. (2016). Experimental investigation of intravascular OCT for imaging of intracranial aneurysms. Int. J. Comput. Assist. Radiol. Surg. 11 (2), 231–241. doi:10.1007/s11548-015-1275-1
Huang, D., Swanson, E. A., Lin, C. P., Schuman, J. S., Stinson, W. G., Chang, W., et al. (1979). Optical coherence tomography. Science 254 (5035), 1178–1181. doi:10.1126/science.1957169
Iosif, C., Saleme, S., Ponsonnard, S., Carles, P., Silveira, E. P., Waihrich, E., et al. (2016). Intravascular optical coherence tomography for the evaluation of arterial bifurcations covered by flow diverters. J. Neurointerv. Surg. 8 (12), 1283–1287. doi:10.1136/neurintsurg-2015-012084
Ishida, F., Tsuji, M., Tanioka, S., Tanaka, K., Yoshimura, S., and Suzuki, H. (2021). Computational fluid dynamics for cerebral aneurysms in clinical settings. Acta Neurochir. Suppl. 132, 27–32. Published online 2021. doi:10.1007/978-3-030-63453-7_4
Kawanabe, Y., Sadato, A., Taki, W., and Hashimoto, N. (2001). Endovascular occlusion of intracranial aneurysms with guglielmi detachable coils: Correlation between coil packing density and coil compaction. Acta Neurochir. (Wien). 143 (5), 451–455. doi:10.1007/s007010170073
Kawase, Y., Suzuki, Y., Ikeno, F., Yoneyama, R., Hoshino, K., Ly, H. Q., et al. (2007). Comparison of nonuniform rotational distortion between mechanical IVUS and OCT using a phantom model. Ultrasound Med. Biol. 33 (1), 67–73. doi:10.1016/j.ultrasmedbio.2006.07.020
King, R. M., Brooks, O. W., Langan, E. T., Caroff, J., Clarencon, F., Tamura, T., et al. (2018). Communicating malapposition of flow diverters assessed with optical coherence tomography correlates with delayed aneurysm occlusion. J. Neurointerv. Surg. 10 (7), 693–697. doi:10.1136/neurintsurg-2017-013502
King, R. M., Langan, E. T., Ughi, G. J., Raskett, C. M., Puri, A. S., Henkes, H., et al. (2020). Acute thrombus burden on coated flow diverters assessed by high frequency optical coherence tomography. Cardiovasc. Interv. Radiol. 43 (8), 1218–1223. doi:10.1007/s00270-020-02482-w
Klisch, J., Weyerbrock, A., Spetzger, U., and Schumacher, M. (2003). Active bleeding from ruptured cerebral aneurysms during diagnostic angiography: Emergency treatment. AJNR. Am. J. Neuroradiol. 24 (10), 2062–2065.
Lee, S. Y., Kang, D. Y., Hong, S. J., Ahn, J. M., Ahn, C. M., Park, D. W., et al. (2020). Optical coherence tomography for coronary bioresorbable vascular scaffold implantation: A randomized controlled trial. Circ. Cardiovasc. Interv. 13 (1), e008383. doi:10.1161/CIRCINTERVENTIONS.119.008383
Li, L., Yongjie, M., Dmytriw, A. A., Jian, R., and Hongqi, Z. (2022). Evaluation of vessel wall apposition for stent-assisted coiling in treatment of vertebral artery aneurysms using optical coherence tomography. Clin. Neuroradiol. 24, 565–570. doi:10.1007/s00062-021-01070-w
Lin, L. M., Jiang, B., Campos, J. K., Beaty, N., Bender, M., Tamargo, R., et al. (2018). Abciximab (ReoPro) dosing strategy for the management of acute intraprocedural thromboembolic complications during pipeline flow diversion treatment of intracranial aneurysms. Interv. Neurol. 7 (5), 218–232. doi:10.1159/000486458
Lopes, D. K., and Johnson, A. K. (2012). Evaluation of cerebral artery perforators and the pipeline embolization device using optical coherence tomography. J. Neurointerv. Surg. 4 (4), 291–294. doi:10.1136/neurintsurg-2011-010102
Luo, C. B., Chang, F. C., Teng, M. M. H., Guo, W. Y., and Chang, C. Y. (2008). Stent management of coil herniation in embolization of internal carotid aneurysms. AJNR. Am. J. Neuroradiol. 29 (10), 1951–1955. doi:10.3174/ajnr.A1268
Lv, N., Karmonik, C., Chen, S., Wang, X., Fang, Y., Huang, Q., et al. (2019). Relationship between aneurysm wall enhancement in vessel wall magnetic resonance imaging and rupture risk of unruptured intracranial aneurysms. Neurosurg. 84 (6), E385–E391. doi:10.1093/neuros/nyy310
Macdonald, R. L., and Schweizer, T. A. (2017). Spontaneous subarachnoid haemorrhage. Lancet 389 (10069), 655–666. doi:10.1016/S0140-6736(16)30668-7
Madaelil, T. P., Grossberg, J. A., Howard, B. M., Cawley, C., Dion, J., Nogueira, R., et al. (2019). Aneurysm remnants after flow diversion: Clinical and angiographic outcomes. AJNR. Am. J. Neuroradiol. 40, 694–698. Published online March 7. doi:10.3174/ajnr.A6010
Maehara, A., Matsumura, M., Ali, Z. A., Mintz, G. S., and Stone, G. W. (2017). IVUS-guided versus OCT-guided coronary stent implantation. JACC Cardiovasc. Imaging 10 (12), 1487–1503. doi:10.1016/j.jcmg.2017.09.008
Marosfoi, M., Clarencon, F., Langan, E. T., King, R. M., Brooks, O. W., Tamura, T., et al. (2018). Acute thrombus formation on phosphorilcholine surface modified flow diverters. J. Neurointerv. Surg. 10 (4), 406–411. doi:10.1136/neurintsurg-2017-013175
Martínez-Galdámez, M., Escartín, J., Pabón, B., Diaz, C., Martin-Reyes, R., Hermosin, A., et al. (2019). Optical coherence tomography: Translation from 3D-printed vascular models of the anterior cerebral circulation to the first human images of implanted surface modified flow diverters. Interv. Neuroradiol. 25 (2), 150–156. doi:10.1177/1591019918808466
Mathews, M. S., Su, J., Heidari, E., Levy, E. I., Linskey, M. E., and Chen, Z. (2011). Neuroendovascular optical coherence tomography imaging and histological analysis. Neurosurgery 69 (2), 430–439. doi:10.1227/NEU.0b013e318212bcb4
Matsuda, Y., Jang, D. K., Chung, J., Wainwright, J. M., and Lopes, D. (2019). Preliminary outcomes of single antiplatelet therapy for surface-modified flow diverters in an animal model: Analysis of neointimal development and Thrombus formation using OCT. J. Neurointerv. Surg. 11 (1), 74–79. doi:10.1136/neurintsurg-2018-013935
Meneveau, N., Souteyrand, G., Motreff, P., Caussin, C., Amabile, N., Ohlmann, P., et al. (2016). Optical coherence tomography to optimize results of percutaneous coronary intervention in patients with non–ST-elevation acute coronary syndrome. Circulation 134 (13), 906–917. doi:10.1161/CIRCULATIONAHA.116.024393
Molyneux, A. J., Birks, J., Clarke, A., Sneade, M., and Kerr, R. S. C. (2015). The durability of endovascular coiling versus neurosurgical clipping of ruptured cerebral aneurysms: 18 year follow-up of the UK cohort of the international subarachnoid aneurysm trial (ISAT). Lancet 385 (9969), 691–697. doi:10.1016/S0140-6736(14)60975-2
Monteiro, A., Lopes, D. K., Aghaebrahim, A., and Hanel, R. (2021). Optical coherence tomography for elucidation of flow-diversion phenomena: The concept of endothelized mural thrombus behind reversible in-stent stenosis in flow-diverters. Interv. Neuroradiol. 27 (6), 774–780. doi:10.1177/15910199211003432
Mouchtouris, N., Hasan, D., Samaniego, E. A., Saiegh, F. A., Sweid, A., Abbas, R., et al. (2022). The woven EndoBridge (WEB) device: Feasibility, techniques, and outcomes after FDA approval. J. Neurosurg. 136 (5), 1266–1272. doi:10.3171/2021.5.JNS21889
Nagaraja, V., Kalra, A., and Puri, R. (2020). When to use intravascular ultrasound or optical coherence tomography during percutaneous coronary intervention? Cardiovasc. Diagn. Ther. 10 (5), 1429–1444. doi:10.21037/cdt-20-206
Neira, R. I., Yamada, K., Shirakawa, M., Uchida, K., and Yoshimura, S. (2021). Application of optical frequency domain imaging to recanalized unruptured internal carotid artery aneurysm treated by flow-diverting stent-assisted coiling. World Neurosurg. x. 146, 1–5. doi:10.1016/j.wneu.2020.09.037
O’Kelly, C. J., Krings, T., Fiorella, D., and Marotta, T. R. (2010). A novel grading scale for the angiographic assessment of intracranial aneurysms treated using flow diverting stents. Interv. Neuroradiol. 16 (2), 133–137. doi:10.1177/159101991001600204
Pasarikovski, C., Ku, J., Ramjist, J., Dobashi, Y., Priola, S., da Costa, L., et al. (2021). GP.3 examining aneurysmal healing after flow diversion treatment using endovascular optical coherence tomography. Can. J. Neurol. Sci. 48 (3), S12–S13. doi:10.1017/cjn.2021.259
Pasarikovski, C. R., da Costa, L., Pereira, V. M., Ramjist, J., Dobashi, Y., and Yang, V. X. D. (2020). Optical coherence tomography-guided flow diversion for aneurysmal treatment. Neurol. Clin. Pract. 10 (4), e30–e32. doi:10.1212/CPJ.0000000000000729
Pasarikovski, C. R., Ku, J. C., Priola, S. M., da Costa, L., and Yang, V. X. D. (2020). Endovascular optical coherence tomography imaging in cerebrovascular disease. J. Clin. Neurosci. 80, 30–37. doi:10.1016/j.jocn.2020.07.064
Raymond, J., Guilbert, F., Weill, A., Georganos, S. A., Juravsky, L., Lambert, A., et al. (2003). Long-term angiographic recurrences after selective endovascular treatment of aneurysms with detachable coils. Stroke 34 (6), 1398–1403. doi:10.1161/01.STR.0000073841.88563.E9
Rear, R., Bell, R. M., and Hausenloy, D. J. (2016). Contrast-induced nephropathy following angiography and cardiac interventions. Heart 102 (8), 638–648. doi:10.1136/heartjnl-2014-306962
Rouchaud, A., Ramana, C., Brinjikji, W., Ding, Y. H., Dai, D., Gunderson, T., et al. (2016). Wall apposition is a key factor for aneurysm occlusion after flow diversion: A histologic evaluation in 41 rabbits. AJNR. Am. J. Neuroradiol. 37 (11), 2087–2091. doi:10.3174/ajnr.A4848
Salem, M. M., Sweid, A., Kuhn, A. L., Dmytriw, A. A., Gomez-Paz, S., Maragkos, G. A., et al. (2022). Repeat flow diversion for cerebral aneurysms failing prior flow diversion: Safety and feasibility from multicenter experience. Stroke 53 (4), 1178–1189. doi:10.1161/STROKEAHA.120.033555
Shimamura, K., Kubo, T., and Akasaka, T. (2021). Evaluation of coronary plaques and atherosclerosis using optical coherence tomography. Expert Rev. cardiovasc. Ther. 19 (5), 379–386. doi:10.1080/14779072.2021.1914588
Sluzewski, M., van Rooij, W. J., Slob, M. J., Bescós, J. O., Slump, C. H., and Wijnalda, D. (2004). Relation between aneurysm volume, packing, and compaction in 145 cerebral aneurysms treated with coils. Radiology 231 (3), 653–658. doi:10.1148/radiol.2313030460
Staarmann, B., Smith, M., and Prestigiacomo, C. J. (2019). Shear stress and aneurysms: A review. Neurosurg. Focus 47 (1), E2. doi:10.3171/2019.4.FOCUS19225
Sun, C., Nolte, F., Cheng, K. H., Vuong, B., Lee, K. K. C., Standish, B. A., et al. (2012). In vivo feasibility of endovascular Doppler optical coherence tomography. Biomed. Opt. Express 3 (10), 2600–2610. doi:10.1364/BOE.3.002600
Tavakol, M., Ashraf, S., and Brener, S. J. (2012). Risks and complications of coronary angiography: A comprehensive review. Glob. J. Health Sci. 4 (1), 65–93. doi:10.5539/gjhs.v4n1p65
Tearney, G. J., Brezinski, M. E., Bouma, B. E., Boppart, S. A., Pitris, C., Southern, J. F., et al. (1979). In vivo endoscopic optical biopsy with optical coherence tomography. Science 276 (5321), 2037–2039. doi:10.1126/science.276.5321.2037
Tearney, G. J., Regar, E., Akasaka, T., Adriaenssens, T., Barlis, P., Bezerra, H. G., et al. (2012). Consensus standards for acquisition, measurement, and reporting of intravascular optical coherence tomography studies. J. Am. Coll. Cardiol. 59 (12), 1058–1072. doi:10.1016/j.jacc.2011.09.079
Thompson, B. G., Brown, R. D., Amin-Hanjani, S., Broderick, J. P., Cockroft, K. M., Connolly, E. S., et al. (2015). Guidelines for the management of patients with unruptured intracranial aneurysms: A guideline for healthcare professionals from the American heart association/American stroke association. Stroke 46 (8), 2368–2400. doi:10.1161/STR.0000000000000070
Tonetti, D. A., Jankowitz, B. T., and Gross, B. A. (2020). Antiplatelet therapy in flow diversion. Neurosurg. 86 (1), S47–S52. doi:10.1093/neuros/nyz391
Ughi, G. J., and Adriaenssens, T. (2017). “Intracoronary optical coherence tomography,” in Computing and visualization for intravascular imaging and computer-assisted stenting (Elsevier), 183–223. doi:10.1016/B978-0-12-811018-8.00008-4
Ughi, G. J., Marosfoi, M. G., King, R. M., Caroff, J., Peterson, L. M., Duncan, B. H., et al. (2020). A neurovascular high-frequency optical coherence tomography system enables in situ cerebrovascular volumetric microscopy. Nat. Commun. 11 (1), 3851. doi:10.1038/s41467-020-17702-7
van der Marel, K., Gounis, M. J., Weaver, J. P., de Korte, A. M., King, R. M., Arends, J. M., et al. (2016). Grading of regional apposition after flow-diverter treatment (GRAFT): A comparative evaluation of VasoCT and intravascular OCT. J. Neurointerv. Surg. 8 (8), 847–852. doi:10.1136/neurintsurg-2015-011843
Vardar, Z., King, R. M., Kraitem, A., Langan, E. T., Peterson, L. M., Duncan, B. H., et al. (2021). High-resolution image-guided WEB aneurysm embolization by high-frequency optical coherence tomography. J. Neurointerv. Surg. 13 (7), 669–673. doi:10.1136/neurintsurg-2020-016447
Vlak, M. H., Algra, A., Brandenburg, R., and Rinkel, G. J. (2011). Prevalence of unruptured intracranial aneurysms, with emphasis on sex, age, comorbidity, country, and time period: A systematic review and meta-analysis. Lancet Neurol. 10 (7), 626–636. doi:10.1016/S1474-4422(11)70109-0
Vuong, B., Genis, H., Wong, R., Ramjist, J., Jivraj, J., Farooq, H., et al. (2014). Evaluation of flow velocities after carotid artery stenting through split spectrum Doppler optical coherence tomography and computational fluid dynamics modeling. Biomed. Opt. Express 5 (12), 4405. doi:10.1364/BOE.5.004405
Yako, R., Matsumoto, H., Masuo, O., and Nakao, N. (2017). Observation of neointimal coverage around the aneurysm neck after stent-assisted coil embolization by optical frequency domain imaging: Technical case report. Oper. Neurosurg. Hagerst. 13 (2), 285–292. doi:10.1093/ons/opw018
Yonetsu, T., Bouma, B. E., Kato, K., Fujimoto, J. G., and Jang, I. K. (2013). Optical coherence tomography. Circ. J. 77 (8), 1933–1940. doi:10.1253/circj.CJ-13-0643.1
Zhao, J., Lin, H., Summers, R., Yang, M., Cousins, B. G., and Tsui, J. (2018). Current treatment strategies for intracranial aneurysms: An overview. Angiology 69 (1), 17–30. doi:10.1177/0003319717700503
Keywords: optical coherence tomography, intracerebral aneurysm, coiling, flow diverting stent, interventional neuroradiology
Citation: Ku JC, Pasarikovski CR, Dobashi Y, Ramjist J, Priola SM and Yang VXD (2022) Review of intraluminal optical coherence tomography imaging for cerebral aneurysms. Front. Photonics 3:1015661. doi: 10.3389/fphot.2022.1015661
Received: 10 August 2022; Accepted: 28 September 2022;
Published: 14 October 2022.
Edited by:
Gijs Van Soest, Erasmus University Rotterdam, NetherlandsReviewed by:
Jiawen Li, University of Adelaide, AustraliaCopyright © 2022 Ku, Pasarikovski, Dobashi, Ramjist, Priola and Yang. This is an open-access article distributed under the terms of the Creative Commons Attribution License (CC BY). The use, distribution or reproduction in other forums is permitted, provided the original author(s) and the copyright owner(s) are credited and that the original publication in this journal is cited, in accordance with accepted academic practice. No use, distribution or reproduction is permitted which does not comply with these terms.
*Correspondence: Jerry C. Ku, SmVycnkua3VAbWFpbC51dG9yb250by5jYQ==
Disclaimer: All claims expressed in this article are solely those of the authors and do not necessarily represent those of their affiliated organizations, or those of the publisher, the editors and the reviewers. Any product that may be evaluated in this article or claim that may be made by its manufacturer is not guaranteed or endorsed by the publisher.
Research integrity at Frontiers
Learn more about the work of our research integrity team to safeguard the quality of each article we publish.