- Climate Change Cluster, Faculty of Science, University of Technology Sydney, Sydney, NSW, Australia
Diatoms are crucial in global primary productivity and carbon sequestration, contributing significantly to marine food webs and biogeochemical cycles. With the projected increase in sea surface temperatures, climate change poses significant threats to these essential organisms. This study investigates the photobiological responses of nine diatom species to rapid changes in light and temperature, aiming to understand their adaptability and resilience to climate-induced environmental fluctuations. Using a high-throughput phenoplate assay, we evaluated the maximum quantum yield of photosystem 2 (Fv/Fm), non-photochemical quenching (NPQ) and additional photosynthetic parameters under varying temperature conditions. Our results revealed significant variability in the photophysiological responses among the species, with temperature emerging as a dominant abiotic factor relative to light, accounting for 13.2%–37.5% of the measured variability. Measurements of effect size of temperature and light on Fv/Fm showed that there is additional significant innate variability in the samples when a homogeneous culture is fractioned in 384 subpopulations. Furthermore, hierarchical clustering analysis of the effect size of temperature, light and innate variability on all measured photosynthetic parameters identified two distinct diatom groups. One group exhibited strong interaction between light intensity and temperature, suggesting active synergetic mechanisms to cope with fluctuating environments, while the other showed potential limitations in this regard. These findings highlight diatoms’ diverse strategies to optimize photosynthesis and manage light and thermal stress, providing insights into their potential responses to future climate scenarios. Furthermore, we demonstrate that using the method presented in this work we can functionally cluster different diatom species.
Introduction
Diatoms are a group of single-celled silicified phytoplankton renowned for their ecological significance and diversity. They contribute significantly to global primary productivity, responsible for 20% (Matsuda et al., 2014) of it, and play a pivotal role in ocean carbon fixation, accounting for up to 50% (Bach and Taucher, 2019) of the total. With an estimated 200 000 (Guiry, 2012; Armbrust, 2009) species, out of which 18 186 are taxonomically defined (Guiry, 2024), diatoms are fundamental to marine food webs, biogeochemical cycling, and carbon sequestration.
Climate change poses significant threats to marine ecosystems, with projected increases in sea surface temperatures of over 1°C over the next century (Kay et al., 2023). Diatoms, producing the majority of biomass resources in aquatic systems, are essential to sustaining higher trophic levels. The survival and growth rates of Southern Ocean diatoms under simulated heatwaves were shown to be influenced by thermal exposure and genetic variation (Samuels et al., 2021). Recent studies have highlighted the impact of marine heatwaves on diatom communities, showing that prolonged exposure to elevated temperatures can lead to significant shifts in community composition and function (McCabe et al., 2016). Their biological responses to temperature changes have been documented at community levels and are well known to have detrimental effects on photodamage and repair of their photosystems (Bártolo et al., 2023).
Given the dual possibilities of diatom resilience or vulnerability to climate change, understanding their limits and adaptability is crucial. Current projects focusing on diatom gene function, evolution, and diversity are extensive (Falciatore et al., 2019), yet they often lack insights into how diatoms will react phenotypically to climate-induced changes.
Recent research has highlighted the challenges and solutions for high-throughput phenotyping of diatoms and other microalgae. Rapid light curves and other chlorophyll fluorometry techniques are widely used to assess photosynthetic performance and stress responses (Consalvey et al., 2005; Morris and Kromkamp, 2003). Novel platforms like Phenoplate, PhenoChip, and LIFT-FRRf enable simultaneous assessment of multiple environmental factors on algal physiology (Herdean et al., 2022; Behrendt et al., 2020; Suggett et al., 2022). These methods can reveal species-specific thermal sensitivity thresholds and pH optima for photosynthesis (Behrendt et al., 2020). Temperature significantly affects photosynthetic parameters, particularly maximum photosynthesis rates (Morris and Kromkamp, 2003). Integrating multiple trait measurements, including growth rate, cell size, and photophysiology parameters, into a single workflow allows for efficient phenotyping of microalgae (Argyle et al., 2021a; Hoch et al., 2023). These advancements contribute to understanding diatom adaptability to environmental changes and support efforts in biotechnology and ecological research (Hoch et al., 2023).
This study aims to fill part of this phenome research gap by investigating the photobiological responses of nine diatom species to fast temperature and light changes using a simple and statistically powerful assay. Additionally, we quantify the impact of thermal and light stress, and the temperature-light interaction on photosynthesis using effect size determined from analysis of variance. By examining these responses, we aim to enrich our understanding of diatom response to climate change driven fluctuations in temperature and to get a measure of the diverse responses different diatom species have.
Materials and methods
Phenoplate measurements were carried out using an Open FluorCAM (PSI, Brno, Czech Republic) in conjunction with a 384-well thermocycler (Mastercycler 384 Gradient, Eppendorf, Germany) as previously described (Herdean et al., 2023). Each plate (Hard-Shell 384-well PCR plate, HSP3805, Biorad, United States) was loaded using a liquid reagent dispenser (Welljet, Integra, Australia) by dispensing 40 μL of a well-mixed culture into each well. The cultures were subfractioned directly from a single source into 384 wells, ensuring uniform distribution and to avoid unequal loading. Each plate contained a culture from a single diatom species and was measured immediately after fractioning, without further incubation or growth. Temperature and light calibrations were carried out using a thermal camera (FLIR C2, Teledyne FLIR, LLC, Wilsonville, OR, United States) and a light meter (LI-COR Biosciences, United States). The temperature profile during the measurements was 18°C for 10 min in darkness with an Fv/Fm measurement at the end of the step, followed by 5 min in various temperatures across the 384-well plate (10.6°C–29.3°C) in darkness, followed by another Fv/Fm measurement; temperature across the plate are shown in Supplementary Table S1. These steps were followed by a 14-step rapid light curve (8–1,280 µmol photons m−2 s−1 broad spectrum white actinic light, from the fluorometer LED array), each step was 60 s with pulse amplitude modulation fluorometry (PAM) measurements after every cycle. Fv/Fm values from light-adapted steps during the rapid light curve were calculated according to the work of Oxborough (Oxborough, 2004).
Calculation of partial eta squared (η2) was carried using IBM SPSS Statistics (Version 28.0.1.1 (14), IBM, Armonk, NY, United States) using the Generalized Linear Mixed Model, with temperature, light, and temperature and light interaction as fixed factors (categorical variables). The diatom culture and well position in 384-well plate were considered random effects, and were quantified accordingly. Each photosynthetic parameter was analysed as a target variable with significance level set at 0.05 (confidence interval at 95%). Temperatures were averaged to the closest integer and resulted in 20 categorical levels; light was measured above each one of the 384-wells (Supplementary Table S1) and values were rounded to increments of 10 µmol photons m−2 s−1, resulting in 120 categorical levels. Hierarchical clustering was performed in OriginPro (Version 10.0.0.154 Academic, OriginLab, Northampton, MA, United States).
The nine diatom species provided by the 100 Diatom Genomes Consortium, Achnanthes brevipes var. intermedia (Toyoda and Williams, 2004; Lee et al., 2013), Mayamaea atomus (Bagmet et al., 2021; Bodnar et al., 2011), Nitzschia palea var. fonticola (Trobajo et al., 2009; Trobajo et al., 2006), Entomoneis sp. (Mejdandžić et al., 2018), Grammatophora marina (Sato et al., 2008; Abu Affan et al., 2006), Navicula arenaria var. rostellata, Cylindrotheca fusiformis (Claquin et al., 2004; Smith and Melis, 1988; Duan et al., 2023), Nanofrustulum trainori, and Plagiogramma staurophorum (Kaczmarska et al., 2017) were maintained under controlled light and temperature conditions. The cultures were grown in an Eppendorf Innova incubator (S44i, Eppendorf South Pacific Pty. Ltd., NSW, Australia) under a light intensity of approximately 20 μmol photons m−2 s−1, for all diatoms except C. fusiformis which was grown in 50 μmol photons m−2 s−1. The light:dark cycle was 18:6 h, with a growth temperature of 18°C, except for C. fusiformis, which was cultured at 23°C. Freshwater diatoms M. atomus and N. palea var. fonticola were cultured in MLA growth media with silica; all other diatoms were cultured in F/2 with silica. Culturing was done in conical glass 150 mL flasks.
Phylogenetic tree has been constructed based on two genes including 18S ribosomal RNA gene and ribulose-1,5-bisphosphate carboxylase/oxygenase large subunit gene (rbcL) curated from NCBI (Supplementary Table S2). There is no genetic sequence for N. trainori; thus, Nanofrustulum shiloi were used for constructing the tree. The two sequences were aligned separately via mafft version 7.526 (Katoh and Standley, 2013) and concatenated via seqkit version 2.8.2 (Shen et al., 2016). Maximum likelihood tree was constructed via IQ-tree version 2.3.3 (Nguyen et al., 2014) and maximum parsimony tree was constructed via mpboots version 1.1 (Hoang et al., 2018). Both approaches were bootstraped 1,000 times and plotted in R version 4.4.1 (Team, 2023) using ggtree (Yu et al., 2017).
Results
To investigate the effects of rapid temperature and light fluctuations on diatom photosynthesis, we employed a high-throughput Phenoplate assay, as illustrated in Figure 1. The method has been previously described and used on various organisms (Herdean et al., 2022; Herdean et al., 2023; England et al., 2024) however, this is the first instance where algal cultures are fractioned in 384 subcultures and measured individually. This allows to quantify the innate (natural) and induced (by temperature or light) variability observed in photosynthetic parameters.
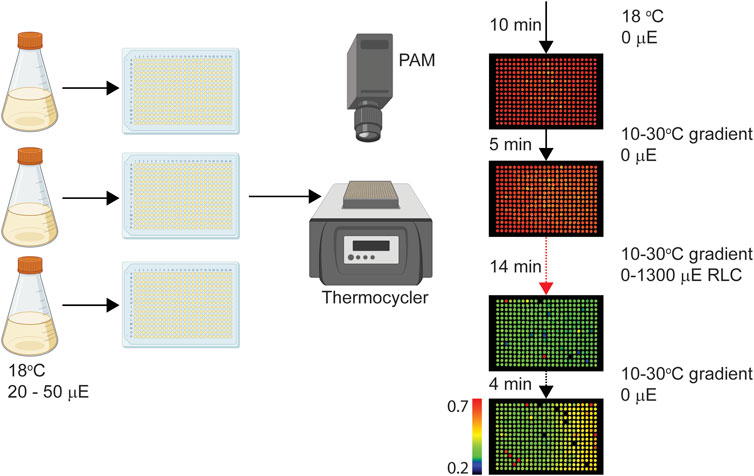
Figure 1. Schematic representation of experimental procedure. Briefly, diatoms were grown in triplicate at 18°C at 20 or 50 µmol photons m−2 s−1 (µE), each replicate was distributed on to a 384 well-plate, which was placed on to a thermocycler. A sequence of temperature changes was carried out by the thermocycler while a pulse amplitude modulated fluorometer (PAM) imaged the samples, as described in materials and methods. Example Fv/Fm data from C. fusiformis is shown on the right side of the figure at 4 time points during the experiment. Colour scaling of images (0.2–0.7) indicates Fv/Fm values, as denoted by the colour bar.
The maximum quantum yield of PSII (Fv/Fm) was assessed for nine diatom species following a 10-min dark adaptation period at 18°C (Figure 2). This temperature matched the growth temperature, selected because it falls within the natural environmental range in which these diatoms are known to thrive. We measured Fv/Fm values of 1,152 individual samples for each species and were able to separate them statistically in 7 distinct groups (Figure 2, statistical significance). Notably, Entomoneis sp. and G. marina exhibited statistically similar Fv/Fm values, suggesting similar photosynthetic efficiencies under the experimental conditions. Likewise, M. atomus and N. arenaria var. rostellata also showed comparable Fv/Fm values, forming another distinct group. The differences among species were statistically significant, as determined by ANOVA and Tukey’s post hoc test (p > 0.05), emphasizing the variability in photosynthetic performance across different diatoms.
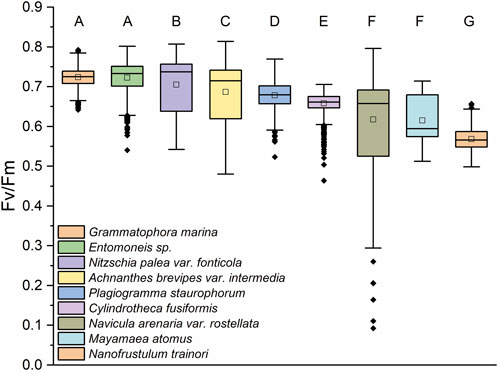
Figure 2. Maximum quantum yield of PSII. Fv/Fm values of all diatom species after 10 min of dark adaptation at 18°C. Values show that the diatoms have distinct PSII efficiencies with Entomoneis sp. and Grammatophora marina being statistically identical, as well as M. atomus and N. rostellata showing similar Fv/Fm values. Letters on top of box plots show the statistical groups determined by ANOVA Tukey test p > 0.05. Number of replicates was 1,152 for each species.
Following the 10 min in dark at 18°C, the protocol continued with 5 min in dark at various temperatures (Supplementary Table S1) after which we measured Fv/Fm, and continued with a rapid light curve at the new temperatures (Figure 1).
The effect sizes (η2) of temperature, light, and their interaction on Fv/Fm were analysed and are presented in pie charts (Figure 3; raw data available in Supplementary Table S3). The analysis revealed that, in all diatom species, 16.2%–31.8% of the variability in Fv/Fm could not be explained by temperature, light or random effects. This unexplained variability, represented by the mustard-yellow section of the charts, is likely indicative of the innate, natural variability within the population (Figure 3). A significant contribution to the observed variability originated from the culture itself (3 total cultures), with the highest contribution observed in P. staurophorum (31.8%). Conversely, the lowest culture-induced variability was seen in N. trainorii and N. fonticola, suggesting these species exhibited more homogeneous biological replicates. The effect size of the culture fractioning in the wells, quantified as a random factor, was neglectable in most diatoms, with the exception of Entomoneis sp., where 36.8% of the effect size in Fv/Fm was attributed to the well position (Figure 3).
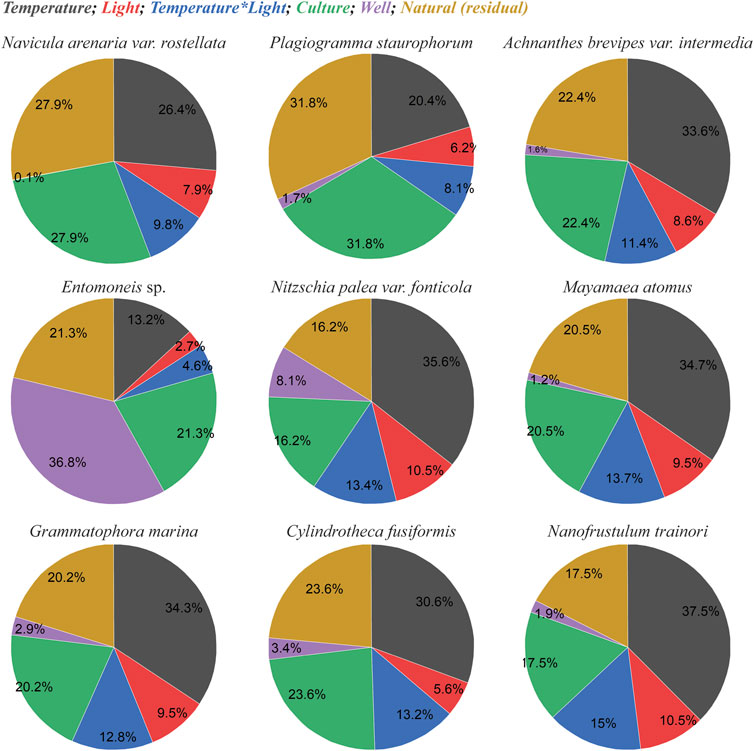
Figure 3. Effect size of temperature, light and temperature-light interaction on Fv/Fm. Pie charts showing the effect size (η2) as a percentage of total measured variability. The contribution of temperature is shown as dark grey, light as red, interacting effect of light and temperature as blue, random effect of the culture as green, random effect of the well position as purple, and the natural variability as mustard yellow. Effect size (Partial Eta Squared, η2) was calculated using results from a Generalized Linear Mixed Model analysis performed in SPSS (see Supplementary Table S3 for calculations).
Between temperature and light, temperature was the dominant driver of variability in Fv/Fm. Its contribution ranged from 13.2% in Entomoneis sp. to 37.5% in N. trainori. The interaction between light and temperature was a more significant factor than light alone across all species, accounting for 4.6%–15% of the variability. Overall, the emerging trend in effect sizes indicates the following hierarchy: temperature had the largest impact, followed by the interaction between temperature and light, while light alone had the smallest effect.
Hierarchical clustering of the effect sizes of temperature and light on photosynthesis was performed to identify patterns and groupings among the diatom species (Figure 4). The clustering utilized effect sizes of temperature, light, temperature-light interaction, and natural variability for photosynthetic parameters such as Fv/Fm, effective PSII quantum yield (Y(II)), non-photochemical quenching (NPQ), fraction of open PSII centers based on a lake model (qL), and coefficient of non-photochemical quenching (qN). The analysis revealed that diatom species could be grouped into clusters based on their photophysiological responses. Species within the same cluster exhibited similar response strategies to temperature and light variations. This suggests that certain diatoms have evolved comparable mechanisms for optimizing photosynthesis and managing light stress. These groupings provide insights into the adaptive strategies employed by different diatoms.
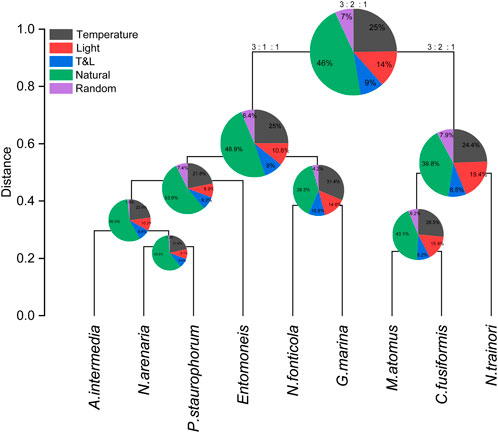
Figure 4. Hierarchical clustering of effect size of temperature and light on photosynthesis. The effect size of temperature (dark grey), light (red), temperature and light interaction (blue), as well as the natural variability (green) of Fv/Fm, Y(II), NPQ, qL, and qN were used to perform the hierarchical clustering. Random effects (purple), as shown in the pie charts, was not used for hierarchical clustering since its effect is not a functional one in the organisms. Pie charts show the effect size as a percentage of total measured variability of all 9 diatom species taken together (largest chart at the top of the figure) and smaller statistical clusters. Pie charts are positioned at the separation points in the dendrogram and represent the effect size distribution of the species in that subset. Numbers above the largest pie chart and on the two main branches indicate the average ratio of temperature: light: light and temperature effect sizes. The vertical axis of the dendrogram show statistical distance with arbitrary units.
Discussion
Diatoms exhibit remarkable diversity in photosynthetic strategies, reflecting their ecological adaptations to variable light and temperature conditions. They have a unique adaptation in how they perform photosynthesis at the carbon fixation level (Reinfelder et al., 2000) and at the light capture level (Fisher et al., 2021). Measurements of photosynthetic traits, such as Fv/Fm and NPQ are extremely powerful at revealing the diverse strategies that diatoms employ to thrive in various environments (Kropuenske et al., 2009). Photoprotective traits (NPQ, qN) and PSII related traits (Fv/Fm, Y(II), qL) have been surprisingly good indicators of the diverse strategies diatoms use to acclimate to their environments (Croteau et al., 2021; Mackey et al., 2008). These traits are also well known to exhibit powerful and heterogeneous responses to light and temperature in a species-dependent manner (Blommaert et al., 2021; Zeng et al., 2020; Argyle et al., 2021b).
In this work, we choose nine different species of diatoms to investigate the level of diversity in their response to light and temperature. Of the nine species, one had previously documented photosynthesis measurements (C. fusiformis (Duan et al., 2023)), seven have documented physiological traits (A. brevipes var. intermedia (Toyoda and Williams, 2004; Lee et al., 2013), M. atomus (Bagmet et al., 2021; Bodnar et al., 2011), N. palea var. fonticola (Trobajo et al., 2009; Trobajo et al., 2006), Entomoneis sp. (Mejdandžić et al., 2018), G. marina (Sato et al., 2008; Abu Affan et al., 2006), C. fusiformis (Claquin et al., 2004; Smith and Melis, 1988; Duan et al., 2023), P. staurophorum (Kaczmarska et al., 2017)), and two are for the first time documented in this paper (N. trainori, N. arenaria var. rostellata). The 100 Diatom Genomes consortium, which aims at sequencing 100 new diatom species, provided the pool of diatoms.
The two freshwater diatoms (M. atomus and N. palea) had different initial Fv/Fm values, but similar patterns in the effect size on Fv/Fm. However, when considering all photosynthetic parameters combined the two species diverged phenotypically to the extent that they were statistically in different clustering branches. Both had ∼35% variability in Fv/Fm coming from temperature, ∼10% from light and ∼13% from the light and temperature interaction. The rest of the variability was partly from variability between cultures but also a significant portion from natural variability, which is ultimately a measure of population heterogeneity.
Temperature was generally expected to have a high effect size (Herdean et al., 2023), which was the case in all diatom species. Considering that temperature was changed abruptly by ±10°C relative to the growth temperature, this points towards a strong response mechanism to rapid temperature fluctuations. These results indicate either specialised response mechanisms to temperature change or increased plasticity to adapt photosynthetic reactions to new temperatures (Wolf et al., 2022).
Interestingly, the interaction between light and temperature showed diverse impacts across the diatom species, with N. trainori exhibiting the highest interaction effect size at 15%. This suggests that some diatoms may have evolved sophisticated mechanisms to cope with simultaneous changes in light and temperature, which could be a critical adaptive strategy in fluctuating environments (Arromrak et al., 2022). These findings may also point to some diatoms employing a form of “photothermal synergy” where the combined effects of light and temperature exceed the sum of their individual impacts. This synergy could enhance the photosynthetic performance and overall fitness of diatoms in dynamic marine environments, allowing them to capitalize on transient conditions of high light and optimal temperatures. Conversely, the synergetic effect of light and temperature may be negative and therefore leading to a faster collapse of the culture. Such synergetic effects would provide a pivotal advantage or disadvantage for diatoms in rapidly changing ecosystems. Surprisingly, the effect of light was lower than temperature and temperature-light interaction when Fv/Fm was considered independent of all other photosynthetic parameters. However, when taken together with NPQ, Y(II), qN and qP the effect size of light was in all cases greater than the combined effect with temperature, as shown in the hierarchical clustering analysis. This highlights how interconnected light and temperature are in photoadaptive plasticity, and that temperature modulates the response to light. This type of plasticity allows diatoms to dynamically adjust their photosynthetic apparatus to cope with varying light conditions, a key factor in their ecological success. Modulating physiological responses, such as pigment composition and non-photochemical quenching, enables diatoms to optimize photosynthesis across different light intensities (Giovagnetti et al., 2014; Derks and Bruce, 2018).
Hierarchical clustering of the effect sizes revealed two distinct groups of diatoms based on their photophysiological responses. One group (M. atomus, C. fusiformis, N. trainori) which showed a distribution of temperature to light to light-temperature of 3:2:1, and a second group (N. fonticola, G. marina, A. intermedia, Entomoneis sp., N. arenaria, P. staurophorum) with an effect size distribution of 3:1:1. This branching of phenotypes was driven by the interaction between light and temperature. In the smaller group, light has a larger effect size relative to the interaction between light and temperature. This may suggest that these species are primarily adapted to environments where light and temperature vary significantly but not necessarily in tandem, such as shallow coastal waters with dynamic light conditions and fluctuating temperatures (e.g., tidal or seasonal changes). In contrast, the larger group, where the effect sizes of light and its interaction with temperature are approximately equal, suggests that these species might be more common in environments where temperature varies more than light. Another remarkable difference was in the increased natural variability in the larger group indicating potential limitations in their ability to adjust to changing light environments using specific response mechanisms. This natural variability-based response strategy to environmental fluctuations is particularly interesting because it employs population heterogeneity as a resilience and survival mechanism. This dual response strategy suggests that diatoms employ diverse strategies to cope with changes in light intensity and temperature, which likely contributes to their wide spread survival. Lastly, we compared the phenotypic clustering with a phylogenetic tree of the same species and found very little resemblance (Supplementary Figure S1), further emphasizing the importance of phenotypic clustering.
In this work we used a rapid Phenoplate (Herdean et al., 2022) assay, with high replicate numbers and a robust statistical analysis to probe the effect size of two of the most important abiotic factors controlling biological activity of diatoms: temperature and light. The results presented show a diverse response of diatoms to these factors, and their interacting effect. We quantified the natural variability in photosynthetic responses, which was particularly pronounced in some species. We hypothesize that this variability stems from the heterogeneous composition of their populations. Future research could further explore the mechanisms driving this variability which could occur through genetic or epigenetic diversity, acclimation processes, and microhabitat variability. Furthermore, phenotypic clustering provided a novel functional dimension that gene-based phylogenetic trees alone are not able to capture. While phylogenetic trees offer insights into the evolutionary relationships and shared ancestry among species, they do not necessarily reflect the current adaptive strategies or physiological performance of organisms in their specific environments. The phenotypic clustering approach revealed that species with similar evolutionary histories might adopt vastly different functional responses to environmental stimuli, such as light and temperature, due to distinct adaptive capacities and ecological strategies. This underlines the importance of integrating phenotypic data with phylogenetic information to gain a more comprehensive understanding of species’ ecological roles and adaptive potential. Additionally, the presented assay can be expanded to quantify the effect size of nutrients, CO2, microbiome or other biotic factors on photosynthesis in diatoms. Understanding how these factors interact could unlock new insights into the resilience and adaptability of diatoms under future climate change scenarios.
Data availability statement
The datasets presented in this study can be found in online repositories. The names of the repository/repositories and accession number(s) can be found in the article/Supplementary Material.
Author contributions
EJ: Data curation, Formal Analysis, Investigation, Writing–review and editing. LH: Conceptualization, Formal Analysis, Methodology, Supervision, Writing–review and editing. KS: Formal Analysis, Investigation, Methodology, Visualization, Writing–review and editing. PR: Resources, Supervision, Writing–review and editing. AH: Conceptualization, Data curation, Formal Analysis, Investigation, Methodology, Project administration, Software, Supervision, Validation, Visualization, Writing–original draft, Writing–review and editing.
Funding
The author(s) declare that no financial support was received for the research, authorship, and/or publication of this article.
Acknowledgments
The authors would like thank Nadine Rijsdijk, Peter Chaerle and Wim Vyverman from Ghent University, Belgium for providing the initial diatom cultures, as well as Phillip Doughty and Lucia Bennar from the Climate Change Cluster for technical support with permits, transport and diatom culturing.
Conflict of interest
The authors declare that the research was conducted in the absence of any commercial or financial relationships that could be construed as a potential conflict of interest.
Generative AI statement
The author(s) declare that no Generative AI was used in the creation of this manuscript.
Publisher’s note
All claims expressed in this article are solely those of the authors and do not necessarily represent those of their affiliated organizations, or those of the publisher, the editors and the reviewers. Any product that may be evaluated in this article, or claim that may be made by its manufacturer, is not guaranteed or endorsed by the publisher.
Supplementary material
The Supplementary Material for this article can be found online at: https://www.frontiersin.org/articles/10.3389/fphbi.2025.1528646/full#supplementary-material
References
Abu Affan, R. K., Jeon, Y.-J., Kim, B.-Y., Lee, J.-B., and Characteristics, G. (2006). Bio-chemical composition and antioxidant activities of benthic diatom Grammatophora marina from jeju coast, korea. Algae 21, 141–148.
Argyle, P. A., Hinners, J., Walworth, N. G., Collins, S., Levine, N. M., and Doblin, M. A. (2021a). A high-throughput assay for quantifying phenotypic traits of microalgae. Front. Microbiol. 12, 706235. doi:10.3389/fmicb.2021.706235
Argyle, P. A., Walworth, N. G., Hinners, J., Collins, S., Levine, N. M., and Doblin, M. A. (2021b). Multivariate trait analysis reveals diatom plasticity constrained to a reduced set of biological axes. ISME Commun. 1, 59. doi:10.1038/s43705-021-00062-8
Armbrust, E. V. (2009). The life of diatoms in the world's oceans. Nature 459, 185–192. doi:10.1038/nature08057
Arromrak, B. S., Li, Z., and Gaitán-Espitia, J. D. (2022). Adaptive strategies and evolutionary responses of microbial organisms to changing oceans. Front. Mar. Sci. 9. doi:10.3389/fmars.2022.864797
Bach, L. T., and Taucher, J. (2019). CO2 effects on diatoms: a synthesis of more than a decade of ocean acidification experiments with natural communities. Ocean. Sci. 15, 1159–1175. doi:10.5194/os-15-1159-2019
Bagmet, V. B., Yu Nikulin, A., Abdullin, S. R., Yu Nikulin, V., and Gontcharov, A. A. (2021). New molecular and phenotypic data on Mayamaea arida (bock) lange-bertalot (bacillariophyta). IOP Conf. Ser. Earth Environ. Sci. 937, 022002. doi:10.1088/1755-1315/937/2/022002
Bártolo, C., Frankenbach, S., and Serôdio, J. (2023). Photoinactivation vs repair of photosystem II as target of thermal stress in epipelic and epipsammic microphytobenthos communities. PLOS ONE 18, e0292211. doi:10.1371/journal.pone.0292211
Behrendt, L., Salek, M. M., Trampe, E. L., Fernandez, V. I., Lee, K. S., Kühl, M., et al. (2020). PhenoChip: a single-cell phenomic platform for high-throughput photophysiological analyses of microalgae. Sci. Adv. 6, eabb2754. doi:10.1126/sciadv.abb2754
Blommaert, L., Chafai, L., and Bailleul, B. (2021). The fine-tuning of NPQ in diatoms relies on the regulation of both xanthophyll cycle enzymes. Sci. Rep. 11, 12750. doi:10.1038/s41598-021-91483-x
Bodnar, O. I., Klochenko, P. D., Grubinko, V. V., and Borisova, Y. V. (2011) Effect of lead ions on functional activity of the diatom Mayamaea atomus, 47, 67–74.
Claquin, P., Kromkamp, J. C., and Martin-Jezequel, V. (2004). Relationship between photosynthetic metabolism and cell cycle in a synchronized culture of the marine alga Cylindrotheca fusiformis (Bacillariophyceae). Eur. J. Phycol. 39, 33–41. doi:10.1080/0967026032000157165
Consalvey, M., Perkins, R. G., Paterson, D. M., and Underwood, G. J. C. (2005). Pam fluorescence: a beginners guide for benthic diatomists. Diatom Res. 20, 1–22. doi:10.1080/0269249x.2005.9705619
Croteau, D., Guérin, S., Bruyant, F., Ferland, J., Campbell, D. A., Babin, M., et al. (2021). Contrasting nonphotochemical quenching patterns under high light and darkness aligns with light niche occupancy in Arctic diatoms. Limnol. Oceanogr. 66, S231–S245. doi:10.1002/lno.11587
Derks, A. K., and Bruce, D. (2018). Rapid regulation of excitation energy in two pennate diatoms from contrasting light climates. Photosynth. Res. 138, 149–165. doi:10.1007/s11120-018-0558-0
Duan, J., Li, C., Zheng, Y., Huang, A., and Xie, Z. (2023). Characterization of exogenous lactate addition on the growth, photosynthetic performance, and biochemical composition of four bait microalgae strains. J. Appl. Microbiol. 134, lxad259. doi:10.1093/jambio/lxad259
England, H., Herdean, A., Matthews, J., Hughes, D. J., Roper, C. D., Suggett, D. J., et al. (2024). A portable multi-taxa phenotyping device to retrieve physiological performance traits. Sci. Rep. 14, 21826. doi:10.1038/s41598-024-71972-5
Falciatore, A., Jaubert, M., Bouly, J.-P., Bailleul, B., and Mock, T. (2019). Diatom molecular research comes of age: model species for studying phytoplankton biology and diversity. Plant Cell. 32, 547–572. [OPEN]. doi:10.1105/tpc.19.00158
Fisher, N. L., Campbell, D. A., Hughes, D. J., Kuzhiumparambil, U., Halsey, K. H., Ralph, P. J., et al. (2021). Divergence of photosynthetic strategies amongst marine diatoms. PLOS ONE 15, e0244252. doi:10.1371/journal.pone.0244252
Giovagnetti, V., Flori, S., Tramontano, F., Lavaud, J., and Brunet, C. (2014). The velocity of light intensity increase modulates the photoprotective response in coastal diatoms. PLOS ONE 9, e103782. doi:10.1371/journal.pone.0103782
Guiry, M. D. (2012). How many species of algae are there? J. Phycol. 48, 1057–1063. doi:10.1111/j.1529-8817.2012.01222.x
Guiry, M. D. (2024). How many species of algae are there? A reprise. Four kingdoms, 14 phyla, 63 classes and still growing. J. Phycol. 60, 214–228. doi:10.1111/jpy.13431
Herdean, A., Hall, C., Hughes, D. J., Kuzhiumparambil, U., Diocaretz, B. C., and Ralph, P. J. (2023). Temperature mapping of non-photochemical quenching in Chlorella vulgaris. Photosynth. Res. 155, 191–202. doi:10.1007/s11120-022-00981-0
Herdean, A., Sutherland, D. L., and Ralph, P. J. (2022). Phenoplate: an innovative method for assessing interacting effects of temperature and light on non-photochemical quenching in microalgae under chemical stress. New Biotechnol. 66, 89–96. doi:10.1016/j.nbt.2021.10.004
Hoang, D. T., Vinh, L. S., Flouri, T., Stamatakis, A., von Haeseler, A., and Minh, B. Q. (2018). MPBoot: fast phylogenetic maximum parsimony tree inference and bootstrap approximation. BMC Evol. Biol. 18, 11. doi:10.1186/s12862-018-1131-3
Hoch, L., Herdean, A., Argyle, P. A., and Ralph, P. J. (2023). High throughput phenomics for diatoms: challenges and solutions. Prog. Oceanogr. 216, 103074. doi:10.1016/j.pocean.2023.103074
Kaczmarska, I., Gray, B. S., Ehrman, J. M., and Thaler, M. (2017). Sexual reproduction in plagiogrammacean diatoms: first insights into the early pennates. PLOS ONE 12, e0181413. doi:10.1371/journal.pone.0181413
Katoh, K., and Standley, D. M. (2013). MAFFT multiple sequence alignment software version 7: improvements in performance and usability. Mol. Biol. Evol. 30, 772–780. doi:10.1093/molbev/mst010
Kay, S., Avillanosa, A. L., Cheung, V. V., Dao, H. N., Gonzales, B. J., Palla, H. P., et al. (2023). Projected effects of climate change on marine ecosystems in Southeast Asian seas. Front. Mar. Sci. 10. doi:10.3389/fmars.2023.1082170
Kropuenske, L. R., Mills, M. M., van Dijken, G. L., Bailey, S., Robinson, D. H., Welschmeyer, N. A., et al. (2009). Photophysiology in two major Southern Ocean phytoplankton taxa: photoprotection in phaeocystis Antarctica and fragilariopsis cylindrus. Limnol. Oceanogr. 54, 1176–1196. doi:10.4319/lo.2009.54.4.1176
Lee, S. D., Park, J. S., and Lee, J. H. (2013). Taxonomic study of the genus Achnanthes (Bacillariophyta) in Korean coastal waters. J. Ecol. Environ. 36, 391–406. doi:10.5141/ecoenv.2013.391
Mackey, K. R. M., Paytan, A., Grossman, A. R., and Bailey, S. (2008). A photosynthetic strategy for coping in a high-light, low-nutrient environment. Limnol. Oceanogr. 53, 900–913. doi:10.4319/lo.2008.53.3.0900
Matsuda, Y., and Kroth, P. G. (2014). “Carbon fixation in diatoms,” in The structural basis of biological energy generation. Editor M. F. Hohmann-Marriott (Dordrecht: Springer Netherlands), 335–362.
McCabe, R. M., Hickey, B. M., Kudela, R. M., Lefebvre, K. A., Adams, N. G., Bill, B. D., et al. (2016). An unprecedented coastwide toxic algal bloom linked to anomalous ocean conditions. Geophys. Res. Lett. 43 (10), 10366–10376. doi:10.1002/2016GL070023
Mejdandžić, M., Bosak, S., Nakov, T., Ruck, E., Orlić, S., Gligora Udovič, M., et al. (2018). Morphological diversity and phylogeny of the diatom genus Entomoneis (Bacillariophyta) in marine plankton: six new species from the Adriatic Sea. J. Phycol. 54, 275–298. doi:10.1111/jpy.12622
Morris, E. P., and Kromkamp, J. C. (2003). Influence of temperature on the relationship between oxygen- and fluorescence-based estimates of photosynthetic parameters in a marine benthic diatom (Cylindrotheca closterium). Eur. J. Phycol. 38, 133–142. doi:10.1080/0967026031000085832
Nguyen, L.-T., Schmidt, H. A., von Haeseler, A., and Minh, B. Q. (2014). IQ-TREE: a fast and effective stochastic algorithm for estimating maximum-likelihood phylogenies. Mol. Biol. Evol. 32, 268–274. doi:10.1093/molbev/msu300
Oxborough, K. (2004). Imaging of chlorophyll a fluorescence: theoretical and practical aspects of an emerging technique for the monitoring of photosynthetic performance. J. Exp. Bot. 55, 1195–1205. doi:10.1093/jxb/erh145
Reinfelder, J. R., Kraepiel, A. M. L., and Morel, F. M. M. (2000). Unicellular C4 photosynthesis in a marine diatom. Nature 407, 996–999. doi:10.1038/35039612
Samuels, T., Rynearson, T. A., and Collins, S. (2021). Surviving heatwaves: thermal experience predicts life and death in a Southern Ocean diatom. Front. Mar. Sci. 8. doi:10.3389/fmars.2021.600343
Sato, S., Mann, D. G., Nagumo, T., Tanaka, J., Tadano, T., and Medlin, L. K. (2008). Auxospore fine structure and variation in modes of cell size changes in Grammatophora marina (Bacillariophyta). Phycologia 47, 12–27. doi:10.2216/07-34.1
Shen, W., Le, S., Li, Y., and Hu, F. (2016). SeqKit: a cross-platform and ultrafast toolkit for FASTA/Q file manipulation. PLOS ONE 11, e0163962. doi:10.1371/journal.pone.0163962
Smith, B. M., and Melis, A. (1988). Photochemical apparatus organization in the diatom Cylindrotheca fusiformis: photosystem stoichiometry and excitation distribution in cells grown under high and low irradiance. Plant Cell. Physiology 29, 761–769.
Suggett, D. J., Nitschke, M. R., Hughes, D. J., Bartels, N., Camp, E. F., Dilernia, N., et al. (2022). Toward bio-optical phenotyping of reef-forming corals using Light-Induced Fluorescence Transient-Fast Repetition Rate fluorometry. Limnol. Oceanogr. Methods 20, 172–191. doi:10.1002/lom3.10479
Toyoda, K., and Williams, D. M. (2004). Description of Achnanthes Bory (Bacillariophyceae) based on Kützing's type slides and materials I. New Morphol. Inf. Achnanthes brevipes var. intermedia (Kütz. ) Cleve. Diatom 20, 159–165.
Trobajo, R., Clavero, E., Chepurnov, V. A., Sabbe, K., Mann, D. G., Ishihara, S., et al. (2009). Morphological, genetic and mating diversity within the widespread bioindicator Nitzschia palea (Bacillariophyceae). Phycologia 48, 443–459. doi:10.2216/08-69.1
Trobajo, R., Mann, D. G., Chepurnov, V. A., Clavero, E., and Cox, E. J. (2006). Taxonomy, life cycle, and auxosporulation of nitzschia fonticola (Bacillariophyta). J. Phycol. 42, 1353–1372. doi:10.1111/j.1529-8817.2006.00291.x
Wolf, K. K. E., Rokitta, S. D., Hoppe, C. J. M., and Rost, B. (2022). Pelagic and ice-associated microalgae under elevated light and 2: contrasting physiological strategies in two Arctic diatoms. Limnol. Oceanogr. 67, 1895–1910. doi:10.1002/lno.12174
Yu, G., Smith, D. K., Zhu, H., Guan, Y., and Lam, T.T.-Y. (2017). ggtree: an r package for visualization and annotation of phylogenetic trees with their covariates and other associated data. Methods Ecol. Evol. 8, 28–36. doi:10.1111/2041-210x.12628
Keywords: diatom, photosynthesis, phenotypic clustering, NPQ, phenoplate
Citation: Juffermans E, Hoch L, Songsomboon K, Ralph PJ and Herdean A (2025) Diatom responses to rapid light and temperature fluctuations: adaptive strategies and natural variability. Front. Photobiol. 3:1528646. doi: 10.3389/fphbi.2025.1528646
Received: 15 November 2024; Accepted: 07 January 2025;
Published: 27 January 2025.
Edited by:
Stefano D’Alessandro, University of Turin, ItalyReviewed by:
Eirini Kaiserli, University of Glasgow, United KingdomJustyna Maria Labuz, Jagiellonian University, Poland
Copyright © 2025 Juffermans, Hoch, Songsomboon, Ralph and Herdean. This is an open-access article distributed under the terms of the Creative Commons Attribution License (CC BY). The use, distribution or reproduction in other forums is permitted, provided the original author(s) and the copyright owner(s) are credited and that the original publication in this journal is cited, in accordance with accepted academic practice. No use, distribution or reproduction is permitted which does not comply with these terms.
*Correspondence: Andrei Herdean, YW5kcmVpLmhlcmRlYW5Ab3V0bG9vay5jb20=
†These authors have contributed equally to this work