- 1Laboratory of Crop Physiology, Department of Plant Biology, Institute of Biology, State University of Campinas (UNICAMP), Campinas, São Paulo, Brazil
- 2Laboratory of Ecophysiology, Embrapa Meio Ambiente, Jaguariúna, São Paulo, Brazil
- 3Department of Research and Development, Embrapa Florestas, Colombo, Paraná, Brazil
Plants have developed specific mechanisms to tackle environmental challenges. During one annual period, a dioecious yerba mate with rhythmic growth can present two growth units (GU1 and GU2) intercalated with two rest periods (R1 and R2). We hypothesized that photosynthetic performance will be much higher under monoculture (MO, high light) than under an agroforestry system (AFS, low light) over annual growth and that secondary sexual dimorphism (SSD) will be more expressed during rest than during growth periods. We evaluated the maximum net photosynthesis (Amax), apparent quantum efficiency of CO2 assimilation (Ф), respiration rate in the dark (Rd) and in the light (RL), RL/Rd ratio, maximum apparent rate of electron transport (Jmax), maximum apparent RuBisCO carboxylation rate (Vcmax), Jmax/Vcmax ratio, and chlorophyll indexes in four (two male and two female) clones. Light was reduced by 92%–95% in AFS compared to MO. Photosynthetic traits generally varied over the annual rhythmic growth and revealed higher photosynthetic performance under MO than AFS. Lower Amax and Rd in all clones under AFS than MO occurred together with higher inhibition of respiration by light (low RL/Rd) under AFS than MO. Despite the strong effect of cultivation systems and growth rhythmicity on photosynthetic traits, our data did not suggest that the frequency of SSD in photosynthetic performance (with the exception of the Jmax/Vcmax ratio) was related to the cultivation system or period of rhythmic growth. The SSD was expressed in a higher Chl b index and lower Chl a/b ratio in female than male plants during R2 and GU2, indicating lower sensitivity of females to extreme light conditions of two cultivation systems than males. Higher Amax and similar respiration rates (Rd and RL) in females compared to in males in early vegetative phases after pruning (herein R1 and GU1) could be considered as a fitness strategy of female plants in their additional effort to produce fruits and seeds in latter phases. Our results additionally revealed the importance of the evaluation period when assessing photosynthesis in plant species with rhythmic growth.
1 Introduction
Ilex paraguariensis A.St.-Hil. (yerba mate) is a dioecious, evergreen, forest tree species that grows up to 15 m tall in its native environment (Carvalho, 2003). It is native to mixed ombrophilous forest formations, as are forests with Araucaria angustifolia occurring in subtropical regions of Paraguay, Argentina, Bolivia, and Brazil (Croge et al., 2021). In the Brazilian economy, yerba mate represents a leading non-wood extractive product from forests in terms of production quantity (Marques et al., 2019).
Yerba mate production of leaves and fine branches used for South American tea preparations is higher in a monoculture (MO) than in an agroforestry system (AFS) (Matsunaga et al., 2014), due to the very low light intensity in the AFS understory (Rakocevic et al., 2008; Rakocevic et al., 2009). In exploration, yerba mate is pruned, maintaining plants as shrubs approximately 2–2.5 m in height, in different geometric forms depending on cultivation system or sex (Rakocevic et al., 2011). To permit yerba mate production, even under an AFS, forests are cleared of various tree and understory species (Petersen et al., 2000), and sometimes, forests are completely cleared to cultivate other crops or to cultivate yerba mate in monoculture (Marques et al., 2019) or in consortium with an annual crop species (de David et al., 2024).
Yerba mate has been intensively studied in the last 3 decades, with a focus on its chemical and phytopharmaceutical properties (Bastos et al., 2007; Rakocevic et al., 2007; Valduga et al., 2016; Gawron-Gzella et al., 2021; Rakocevic et al., 2023a; Aguiar et al., 2024; Marcheafave et al., 2025), on technological and industrial applications (Buffo, 2017), and on management and breeding for improving yield (Mazuchowski et al., 2007; Sturion et al., 2017; Tomasi et al., 2024). In fact, research on yerba mate is rarely oriented to plant physiology (Rakocevic et al., 2009; Rakocevic et al., 2011; Rakocevic et al., 2024) or biology of growth under field conditions (Rakocevic et al., 2012; Matsunaga et al., 2014). As a consequence, such aspects were not mentioned in one recent review of research on this species by Gonçalves and Valduga (2023).
More than 70% of tree species have rhythmic growth (Hallé et al., 1978), meaning that the organogenesis and elongation are characterized by periods of visible aerial plant growth and rests (Hallé and Martin, 1968). The portions of shoots developed during an uninterrupted period of extension are named growth units (GUs). During one annual period, yerba mate can present two GUs (spring and fall) and two rest periods (summer and winter) (Rakocevic et al., 2012; Matsunaga et al., 2014). This pattern differs among the most vigorous individuals, which do not stop growing during the first summer rest in MO, likely due to the high-light environment (Guédon et al., 2018). The phenology of dioecious yerba mate is associated with growth rhythmicity, where the end of spring flush (GU) corresponds to flowering (in both male and female plants, as this species has a dioecious nature), while the summer growth rest corresponds to fruit ripening in female plants (Rakocevic et al., 2009; Rakocevic et al., 2012).
Another important characteristic of yerba mate is the secondary sexual dimorphism (SSD), that is, differences between males and females in characteristics not directly related to gamete production—which is related to phenology and environmental pressures (Rakocevic et al., 2009; Rakocevic et al., 2011; Rakocevic et al., 2012; Rakocevic et al., 2023b; Rakocevic et al., 2024; Matsunaga et al., 2014). SSD in yerba mate is expressed through many ecophysiological traits, including leaf mass, plant architecture, leaf and entire plant gas exchange (Rakocevic et al., 2011; Rakocevic et al., 2012; Rakocevic et al., 2024), or even biomass production (Rakocevic et al., 2023b). Those differential responses can be considered a fitness strategy by the female plants in their effort to reproduce and are expressed in terms of leaf and plant photosynthetic rates in some phenophases (Rakocevic et al., 2009; Rakocevic et al., 2011). One adult female tree produces 0.5–14 kg of fruits, depending on genotype and region (Rakocevic et al., 2012). A higher reproductive investment of yerba mate females to produce fruits might be compensated by greater leaf photosynthesis than males in stages preceding flowering and fruit ripening (Rakocevic et al., 2009) and also at the beginning of fruit ripening (Rakocevic et al., 2024).
The plasticity of plants has revealed morphological and allocation responses to environmental stimuli along with their phenology and ontogeny. As they are simultaneously exposed to diverse abiotic stresses (drought, salinity, low/high light, and mineral deficiency), plants have developed specific mechanisms to tackle abiotic stresses (Melnikova et al., 2017). In various dioecious species, female plants reproduce successfully under limiting environments by exploiting the resources more efficiently than male plants, as in Amaranthus palmeri (Korres et al., 2023). Female Populus tremuloides trees can produce higher biomass than male trees grown in low-nitrogen soil and at ambient CO2, but this pattern is inverted under elevated CO2 (Wang and Curtis, 2001). Similarly, various SSD responses in yerba mate are strongly dependent on the environment, especially light conditions, with different effects observed in female and male plants over phenology under monoculture (high light) and forest understory (low light) (Rakocevic et al., 2009; Rakocevic et al., 2011; Rakocevic et al., 2024). For example, the photosynthesis of young-fully expanded leaves of yerba mate plants grown in anthropized forests (low light) is higher in females than in males but only during the winter growth pause (Rakocevic et al., 2009). Until now, the SSD in photosynthetic performance has only been investigated at the end of spring flush/beginning of fruit ripening in clonal yerba mate (Rakocevic et al., 2024). In this stage, the apparent quantum efficiency (Ф), respiration in the light (RL), RL/Rd ratio (Rd is respiration in the dark), maximum apparent rate of electron transport (Jmax), and maximum apparent carboxylation rate (Vcmax) are higher in females than in males under low light conditions of AFS, but such differentiation is not observed under MO. Simulations of environmental pressures, such as low temperature, water stress, and pruning, promote drastic changes in photosynthetic photon flux density (PPFD) and in the red-to-far-red ratio (R:FR) and result in higher sensitivity of females than male individuals in terms of biomass production (Matsunaga et al., 2014).
The variations in SSD in yerba mate photosynthetic characteristics, or in any tree species, had not been associated with its rhythmic growth. Here, we hypothesized that photosynthesis in yerba mate will be much higher under high light (MO) than under low light (AFS) over the four periods of rhythmic annual growth and that SSD will be more expressed during the rest periods than during the periods of GU formation. To test those hypotheses, four clones (two male and two female) of yerba mate cultivated under MO and AFS were followed during two rest and two GU formation periods.
2 Materials and methods
2.1 Cultivation system and dynamics of measurements during one annual yerba mate rhythmic growth cycle
The experiment was established in the experimental field area of the Federal University of Santa Catarina (UFSC), near Curitibanos county (27°19′09″S, 50°42′39″W, 836 m a.s.l.), Southern Brazil, where two cultivation systems of yerba mate were set in neighboring plots, with 150 m distance between plots (75 m × 20 m). One system was the anthropized rainforest in recovery enriched with yerba mate in its understory (agroforestry system, AFS), and the second one was the full-sun cultivation (monoculture, MO). The rainforest was composed of native and non-native tree species (A. angustifolia, Ocotea spp., Peltophorum dubium, Luehea divaricata, Cordia ecalyculata, Cordia trichotoma, Eugenia involucrata, Syagrus romanzoffiana, Podocarpus spp., Pinus elliottii, Eucalyptus spp. etc.) and understory vegetation.
The climate is defined as subtropical humid (Cfa) according to the Köppen–Geiger classification, with regularly distributed rains (approximately 1,480 mm annually) and an average air temperature of 20.8°C ± 0.3°C in the warmest month (Alvares et al., 2014). The soil is Dystric Cambisol, and its physico-chemical composition was based on samples collected from 0 to 20 cm depth before the planting of yerba mate in 2018. Soil pH in H2O and CaCl2 was 4.8 or 4.15, respectively, sum of bases = 2.02 cmolc dm−3, base saturation = 13.8%, cation exchange capacity at pH 7 = 14.62 cmolc dm−3, effective cation exchange capacity = 4.37 cmolc dm−3, Ca = 1.86 cmolc dm−3, Mg = 0.54 cmolc dm−3, K = 0.16 cmolc dm−3, Al = 2.35 cmolc dm−3, H + Al = 12.78 cmolc dm−3, C = 22.69 g dm−3, OM = 39.03 g dm−3, Fe = 48.35 mg dm−3, Mn = 13.6 mg dm−3, Cu = 11.85 mg dm−3, Zn = 0.86 mg dm−3, and P = 2.52 mg dm−3. Fertilization was done in accordance with soil analyses and recommendations for yerba mate planting and post planting (Penteado Junior and Goulart, 2019). In the MO area, 11 g of N and 16 g of P were used per planting hole, respectively. After that, an annual topdressing fertilization with 30 g of P per plant and two annual fertilizations with 20 g of N and 10 g of K per plant were made. In the AFS area, 11 g of N and 22 g of P were used per planting hole. After that, an annual topdressing fertilization with 40 g of P per plant and two annual fertilizations with 20 g of N and 15 g of K per plant were made. Mechanical weed control was done, and there was no pest/disease management.
Young yerba mate clonal plants from the genetic improvement program of Embrapa (Sturion et al., 2017) were planted in June 2018, with 3 m between the rows and 1.5 m between plants in the row. Among 20 clonal and progeny plants, two male (♂) and two female (♀) clones were chosen for evaluation when the plants were 3 years old. Male clones were labeled “1” and “82,” and female clones were labeled “12” and “84.” Three plants of each clone were followed in each cultivation system (MO and AFS). Each of the three replicate plants per clone was arranged in separate rows, respecting randomized experimental design. The selection was based on the presence of 5–6 visible growth units (GU), using the chronological age as a reference. In December 2021 (beginning of the summer rest period), formation pruning was performed at 40 cm of stem height, leaving approximately 10%–20% of photosynthesizing leaf area for plant recovery.
The measurements of light intensity and photosynthetic parameters (light curve parameters and chlorophyll a and b indexes) were performed in four seasons (summer, fall, winter, and spring). Those periods of measurements corresponded to plant growth rhythmicity after the pruning in December 2021: (i) in February 2022 (summer), end of the growth rest 1 (R1) period, (ii) in May 2022 (fall), end of the growth unit 1 formation (GU1), (iii) August 2022 (winter), end of the growth rest 2 (R2) period, and (iv) November 2022 (spring), end of the growth unit 2 (GU2) formation.
2.2 Light and temperature measurements
Photosynthetic photon flux density (PPFD, µmol photons m−2 s−1) was measured using silicon pyranometer smart sensors (S-LIx-M003, HOBO, Bourne MA, United States), which capture the sun radiation between 400 nm and 700 nm. The PPFD values were recorded with a data logger (H21-USB Data Logger USB, HOBO, Bourne MA, United States) every 10 s, and the mean values were calculated every 10 min and are here explored as hourly mean values. The silicon sensor was maintained from the summer to the spring season in 2022, at 2 m from the soil. Simultaneously to the measurements of PPFD, the air temperature daily course data were registered in both systems during the four seasons. For that, the U23-003 HOBO 2x external temperature data logger with an incorporated sensor (HOBO, Bourne, MA, United States) was used. The one available data logger was moved from one to the other cultivation system every 2–3 weeks during the experimental period. The hourly mean values and standard errors (SE) of diurnal PPFD and daily temperature course in each of the four seasons (summer, fall, winter, and spring) and in each cultivation system (n = 18–72) were calculated.
2.3 Measurements of relative leaf chlorophyll indexes
Relative chlorophyll (Chl) a and b indexes were measured on recently expanded mature leaves, considering each plant of each clone and gender grown under each cultivation system and during each of the four seasons. A portable chlorophyll meter model CFL 2060 (Falker, Porto Alegre RS, Brazil) was used, showing the relative Chl a and b values (indexes) that are highly correlated to concentrations of pigments (Schlichting et al., 2015). Measurements were taken during the morning hours (9:00 to 11:00 a.m.). The ratio Chl a and b was also calculated.
2.4 Measuring and modeling light response curves
The responses of CO2 assimilation to light (Anet/PPFD curves) in yerba mate were measured on the recently expanded mature leaf of each observed plant of each clone and gender, grown under each cultivation system, during each of the four seasons (rhythmic growth periods). The Anet (μmol CO2 m−2 s−1) responses were registered considering 23 light intensities (PPFD) reported in µmol photons m−2 s−1: 1,500, 1,200, 800, 500, 200, 100, 90, 80, 70, 65, 60, 55, 50, 45, 40, 35, 30, 25, 20, 15, 10, 5, and 0 (Supplementary Figure S1A). The 6 cm2 cuvette was fitted with a red-blue light source (6400-02B, LI-COR, Lincoln NE, United States), and [CO2] was set to 420 μL L−1. For the curve, the Anet value at PPFD = 0 μmol m−2 s−1 was assumed as the dark respiration (Rd, μmol m−2 s−1, Rakocevic et al., 2024). The rate of respiration in the light (RL, μmol m−2 s−1) was calculated based on Kok’s method (Kok, 1948) as the y-axis intercept of a first-order linear regression fitted to plots of Anet/PPFD to measurements made over the range of PPFD from 25 μmol m−2 s−1 to 65 μmol m−2 s−1. Gas exchange data were corrected for the increase in intercellular CO2 concentrations (Ci) with decreasing PPFD, which can result in reduced rates of oxygenation and increased rates of carboxylation for RuBisCO. The correction was applied by adjusting RL through iteration to minimize the intercept of photosynthetic electron transport (J, μmol m−2 s−1) as a function of PPFD (Kirschbaum and Farquhar, 1987). J was calculated using the CO2 compensation point in the absence of RL (Γ*, μmol m−2 s−1, von Caemmerer and Farquhar, 1981), as shown in Equation 1:
The effects of atmospheric [O2] or [CO2] on oxygenation (Vo) and carboxylation (Vc) of RuBisCO at saturating light and also RuBisCO kinetic constants (Kc = 272.38 and Ko = 165.82 μM at 25°C) previously determined by Bernacchi et al. (2002) were used to calculate the maximum rate of carboxylation of RuBisCO (Vcmax, μmol m−2 s−1), as in Equation 2:
Γ* from Equations 1, 2 is dependent on the RuBisCO specificity factor Ko for O2 partial pressure (von Caemmerer and Farquhar, 1981) and was calculated as in Equation 3:
The Vo and Vc also used to compute Γ* at PPFD of 500 μmol m−2 s−1 [considered as the light saturation point for yerba mate (Rakocevic et al., 2012)], were estimated as shown in Equations 4, 5:
The maximum net photosynthesis (Amax, μmol m−2 s−1)—a proxy of photosynthetic capacity—was calculated from the modeled A’net/PPFD curves (Supplementary Figure S1B), as well as the degree of light inhibition of respiration, determined by the RL/Rd ratio (da Silva et al., 2017; da Silva et al., 2018) and the apparent quantum efficiency of CO2 assimilation (Ф, μmol CO2 μmol photons−1). Ф was calculated from the initial linear slope of the modeled light response curves (PPFD <30 μmol m−2 s−1), while the maximum apparent rate of electron transport (Jmax, μmol m−2 s−1) was considered as the computed value for PPFD at 1,500 μmol m−2 s−1. Additionally, the ratio between the maximum apparent rate of electron transport and the maximum apparent RuBisCO carboxylation rate (Jmax/Vcmax) was calculated.
2.5 Experimental design and statistical analyses
A complete randomized design with four clones (two females and two males) grown in agroforestry and monoculture situations over four seasons with three replications (plants) was used. Two-way ANOVA was applied to analyze the effects of clones or genders (four clones or two genders) and two cultivation systems (separately for each season) on Chl indexes and photosynthetic traits derived from light response curves. Two families of comparisons/mean contrasts were done: 1) clones and cultivation system effects and 2) genders and cultivation system effects, both calculated separately for each season/growth period. ANOVAs considered mixed linear models (using the lme function from the nlme package) and maximum likelihood to test the significance of studied variables. The Bartlett homogeneity test and the Shapiro normality test were performed for each variable in each season in a model construction. Clones (or genders) and cultivation systems were considered fixed factor effects, while plant number (repetitions) was a random effect observed separately for each season/growth period. If the interaction was not significant, the model reduction was applied (and fitted again). For comparison of variables among the four seasons, one-way ANOVA was performed where season was considered a fixed factor effect, while total plant number was considered as a random effect. All ANOVAs were performed at 95% confidence, followed by a Tukey Honestly Significant Difference test, where p < 0.1 for mean comparisons was considered significant. p-values, estimated means, and standard errors (SE) are shown in the figures. When interaction among factors was significant, only the p-value of interaction was shown, as interactions represent dependence between factors. A second family of comparisons is shown above the figures that compare clone and cultivation system effects during each season/growth period, considering gender and cultivation system effects during each season/growth period. The dynamics of responses over the seasons (one-way ANOVA) are shown as red uppercase letters near season indications. Fisher’s exact test was used to analyze the significance of counting occurrences of SSD over the rhythmic growth (“Yes” for SSD expressed over the rest and growth unit formations and “No” for not expressed). All statistical analyses were performed using R. 4.2.1 software (R Core Team, 2022), using the nlme, emmeans, multcomp, ggplot2, and reshape2 packages.
3 Results
3.1 Light and temperature dynamics in monoculture and agroforestry systems over seasons
The mean midday PPFD values at the top of the yerba mate canopy in MO were 1,120 μmol m−2 s−1, 850 μmol m−2 s−1, 790 μmol m−2 s−1, and 950 μmol m−2 s−1 during the summer, fall, winter, and spring, respectively (Figure 1A). The maximum midday PPFDs registered in MO were 1,760 μmol m−2 s−1, 1,525 μmol m−2 s−1, 1,510 μmol m−2 s−1, and 2,000 μmol m−2 s−1 registered on open-sky days during the summer, fall, winter, and spring, respectively. The incoming light was drastically reduced in AFS, where midday PPFD values were ∼46 μmol m−2 s−1, 39 μmol m−2 s−1, 53 μmol m−2 s−1, and 75 μmol m−2 s−1 during the summer, fall, winter, and spring, respectively (Figure 1B). Light availability in the AFS strongly depended on the upper leaf layer distribution in the forest canopy and could show asymmetric diurnal distribution, as in the summer. The incoming midday PPFD in the AFS was only 4%, 5%, 7%, or 8% of the midday PPFD measured in the MO. The mean daily temperatures were 18.9°C in MO and 17.3°C in AFS during the summer, 13.2°C in MO and 11.0°C in AFS during the fall, 13.6°C in MO and 12.4°C in AFS during the winter, and 18.3°C in MO and 15.5°C in AFS during the spring (Figures 1C, D).
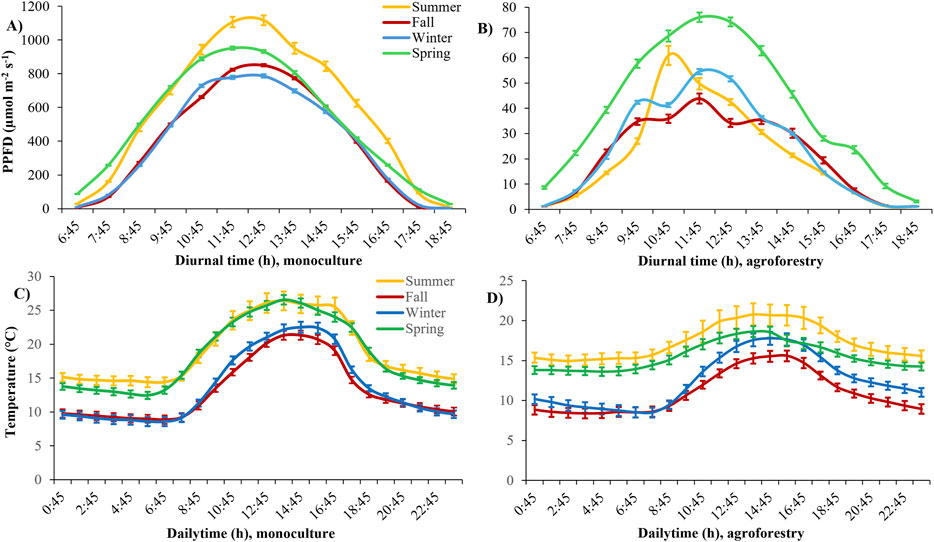
Figure 1. Microclimate in two yerba mate cultivation systems: (A) light intensity in monoculture, (B) light intensity in agroforestry, (C) air temperature in monoculture, and (D) air temperature in agroforestry. Means ± SE (n = 18–72) of measured photosynthetic photon flux density (PPFD) over the diurnal cycle and air temperature over daily hours during four seasons are shown.
3.2 Chlorophyll indexes as affected by clones, genders, cultivation systems, and seasons
The effects of clone, gender, and cultivation systems on the Chl a index were strongly dependent on season (Figure 2A; Supplementary Table 1). The Chl a index was the lowest during the summer rest (R1) and the highest during the spring growth unit (GU2), whereas it was changed by cultivation systems during the summer and fall (R1 and GU1, Supplementary Table 2). During the summer rest (R1), the Chl a index was higher in the AFS than in the MO in the female (♀) clone 84, while the opposite response was observed in ♀ clone 12. The Chl a indexes of male (♂) clones did not vary due to the cultivation system in the summer, but ♂ clone 1 and ♀ clone 84 showed the lowest values under MO. Higher Chl a index values were registered in MO than in AFS during the intensive regrowth period (GU1). During the winter rest (R2), the ♂ clone 1 had a lower Chl a index than the fall other clones. Gender did not show any effect on Chl a index, regardless of the season or cultivation system (Supplementary Table 3).
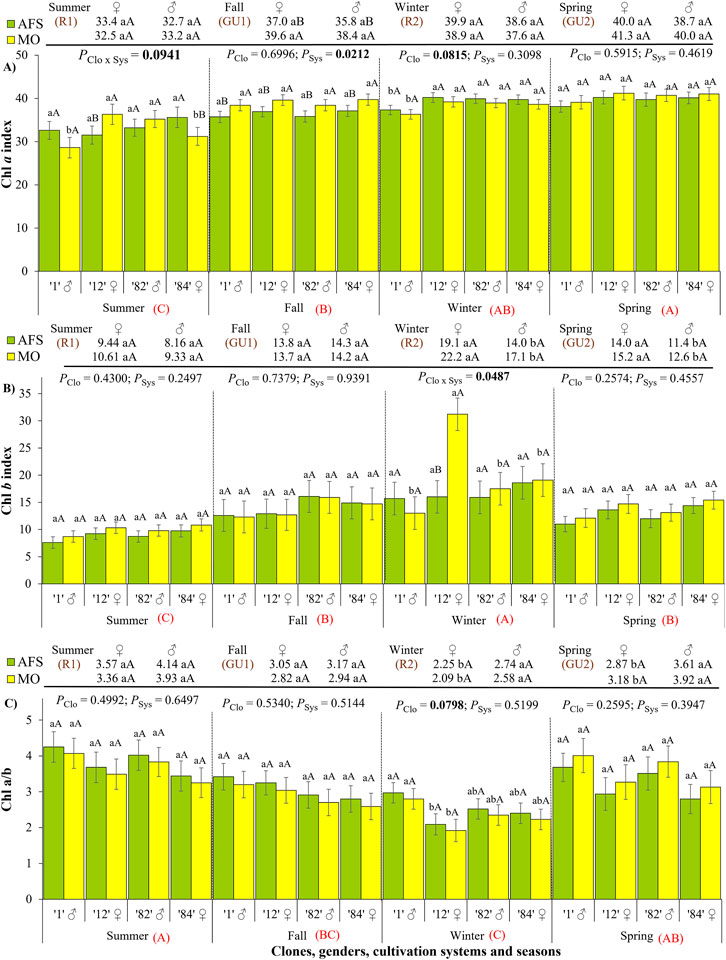
Figure 2. Yerba mate chlorophyll (Chl) indexes: (A) Chl a, (B) Chl b, and (C) ratio between the Chl a and b estimated in two cultivation systems, monoculture (MO) and agroforestry system (AFS), and four clones [1 (♂), 12 (♀), 82 (♂), and 84 (♀)] in four seasons [summer rest 1 (R1), fall growth unit 1 (GU1), winter rest 2 (R2), and spring growth unit 2 (GU2)]. Estimated mean ± SE and p-values with a significance level of <0.1 (bold when significant) are shown. The second family of comparisons is shown above each graph, comparing two genders (female ♀ and male ♂) in each cultivation system and season. For both families of comparisons, lowercase letters compare clone or gender responses under each cultivation system, while uppercase black letters compare cultivation systems for each clone (n = 3) or gender (n = 6) in each season. The uppercase red letters near each season’s indications compare seasonal responses (p < 0.0001 for all three indexes).
The highest values of the Chl b index were noticed during the winter rest (R2), decreasing during the fall and spring (GU1 and GU2), and the lowest values were recorded during the summer rest R1 (Figure 2B; Supplementary Table 1). The Chl b index varied due to the cultivation system only during the winter rest (R2), when it was higher under MO than under AFS in ♀ clone 12. During R2, ♀ clone 12 showed a higher Chl b index than the other three clones under MO (Supplementary Table 2). The leaves of ♀ plants had a higher Chl b index than ♂ ones during the R2 and GU2 periods, regardless of the cultivation system (Supplementary Table 3).
The Chl a/b ratio was the highest in the summer rest (R1) and the lowest in the winter rest period (R2) (Figure 2C). This ratio did not differ between the growth unit periods (GU1 and GU2), and it did not vary due to the cultivation system (Supplementary Table 2). However, ♂ clone 1 had higher Chl a/b values than ♀ clone 12 during R2, regardless of the cultivation system. The leaves of ♀ plants had a lower Chl a/b ratio than the leaves of ♂ plants during the R2 and GU2 periods in both cultivation systems (Supplementary Table 3).
3.3 Photosynthetic traits as affected by clones, genders, cultivation systems, and seasons
The highest maximum net photosynthesis (Amax) values were registered during winter rest (R2) and fall growth unit (GU2) periods, attaining 15.8 μmol m−2 s−1, and the lowest values were registered during spring growth unit (GU2) and summer rest (R1) periods (Figure 3A; Supplementary Table 1). When considering Amax, there was no interaction between clone and cultivation system or between gender and cultivation system (Supplementary Tables 2, 3). The leaves of yerba mate plants cultivated under MO presented higher Amax values than those under AFS during all four seasons, decreasing by 21%–45% in AFS compared to MO. Clone and gender affected Amax only during the summer rest (R1) and the fall growth unit formation (GU1) periods. During those two periods, the leaves of female plants had higher Amax values than males, with the highest values registered in ♀ clone 84 during the summer, while the lowest ones were registered in ♂ clone 1 during the fall.
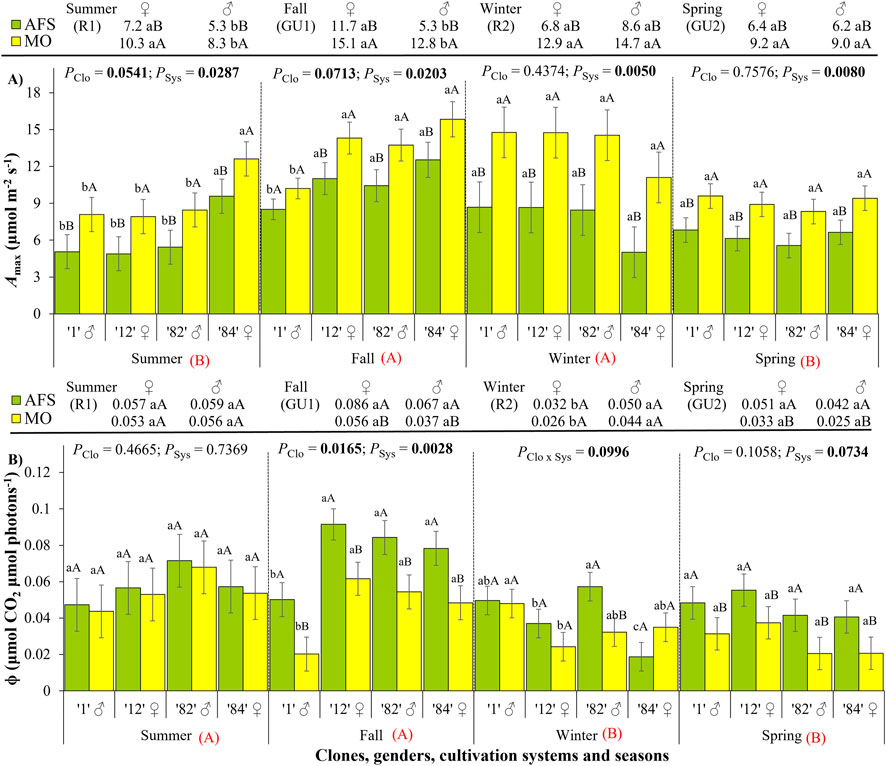
Figure 3. Parameters of photosynthesis derived from yerba mate light curves: (A) maximum net photosynthesis (Amax, μmol m−2 s−1) and (B) apparent quantum efficiency of CO2 assimilation (Ф, μmol CO2 μmol photons−1) estimated in two cultivation systems, monoculture (MO) and agroforestry system (AFS), and four clones [1 (♂), 12 (♀), 82 (♂), and 84 (♀)] in four seasons [summer rest 1 (R1), fall growth unit 1 (GU1), winter rest 2 (R2), and spring growth unit 2 (GU2)]. Estimated mean ± SE and p-values with a significance level of <0.1 (bold when significant) are shown. The second family of comparisons is shown above each graph, comparing two genders (female ♀ and male ♂) in each cultivation system and season. For both families of comparisons, lowercase letters compare clone or gender responses under each cultivation system, while uppercase black letters compare cultivation systems for each clone (n = 3) or gender (n = 6) in each season. The uppercase red letters near each season’s indications compare seasonal responses (p < 0.0001 for both Amax and Ф).
The apparent quantum efficiency of CO2 assimilation (Ф) was higher during the R1 and GU1 than during the R2 and GU2 periods (Figure 3B; Supplementary Table 1). The cultivation systems changed Ф from fall to spring when Ф had been higher in AFS (attaining a mean value of 0.092 μmol CO2 μmol photons−1) than in MO in all clones (Supplementary Table 2). During the fall growth unit period (GU1), the lowest Ф values were observed in the ♂ clone 1 compared to the three other clones, but no significant gender segregation was noticed. During the winter rest period (R2), the lowest Ф value was estimated in ♀ clone 84 (0.019 μmol CO2 μmol photons−1) and the highest in ♂ clone 82 (0.057 μmol CO2 μmol photons−1) under AFS. Under MO and during the winter R2 period, the highest Ф value was estimated in ♂ clone 1, whereas the lowest mean value was found in ♀ clone 12, resulting in lower Ф values in female than male leaves in both light environments (Supplementary Table 3).
The highest values of dark respiration (Rd) were registered during spring under MO (approximately 5.4 μmol m−2 s−1), whereas the mean values during the other seasons varied between 2 μmol m−2 s−1 and 3 μmol m−2 s−1 under MO (Figure 4A). Yerba mate leaves under AFS presented lower Rd values than under MO during all seasons. Clone differentiation in Rd was noticed only during the summer rest period 1 (R1) and spring growth unit 2 (GU2) periods (Supplementary Table 2). The Rd values of ♂ clone 82 and ♀ clone 12 were higher than those of ♂ clone 1 and ♀ clone 84 under both cultivation systems during the summer R1 period. On the other hand, the mean Rd value of ♀ clone 84 was higher than those of ♂ clone 82 and ♀ clone 12 under MO during the spring GU2 period. Such variation in clonal Rd responses indicates that no secondary sexual dimorphism was observed in Rd during the annual experimental period (Supplementary Table 3).
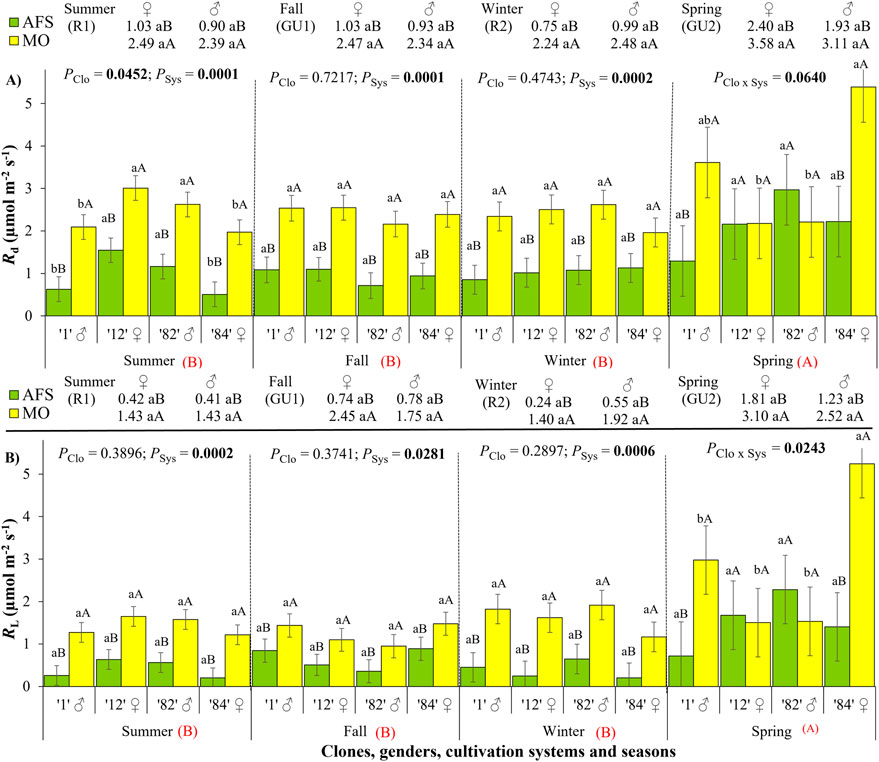
Figure 4. Respiration rates derived from yerba mate light curves: (A) dark respiration (Rd, μmol m−2 s−1) and (B) light respiration (RL, μmol m−2 s−1) estimated in two cultivation systems, monoculture (MO) and agroforestry system (AFS), and four clones [1 (♂), 12 (♀), 82 (♂), and 84 (♀)] in four seasons [summer rest 1 (R1), fall growth unit 1 (GU1), winter rest 2 (R2), and spring growth unit 2 (GU2)]. Estimated mean ± SE and p-values with a significance level of <0.1 (bold when significant) are shown. The second family of comparisons is shown above each graph, comparing two genders (female ♀ and male ♂) in each cultivation system and season. For both families of estimations, lowercase letters compare clone or gender responses under each cultivation system, while uppercase black letters compare cultivation systems for each clone (n = 3) or gender (n = 6) in each season. The uppercase red letters near each season’s indications compare seasonal responses (p < 0.0001 for both Rd and RL).
Light respiration (RL) was lower than Rd but had a similar trend through the seasons (Figure 4; Supplementary Table 1). The only difference between the Rd and RL trends was observed during the summer rest (R1) period when RL did not differ among clones (Figure 4B), as happened for Rd (Figure 4A). Yerba mate leaves under AFS presented lower RL values than under MO during all seasons (Figure 4B). A significant clone effect was registered only during the spring GU2 period when ♀ clone 84 showed the highest RL under MO conditions. No SSD was observed in RL during any season (Supplementary Table 3).
The highest values of maximum RuBisCO carboxylation rate (Vcmax) and maximum electron transport rate (Jmax) were registered during the fall GU1 period, and the lowest ones during the spring GU2 period (Figure 5; Supplementary Table 1). Yerba mate leaves under AFS presented lower Vcmax than under MO from fall GU1 to spring GU2 period (Figure 5A; Supplementary Table 2). A similar trend was observed for Jmax but with differences among clones (Figure 5B; Supplementary Table 2). Clones 1 (♂) and 84 (♀) during the fall and clone 84 (♀) during the spring GU2 had similar Jmax values in both cultivation systems, whereas the other two clones showed higher Jmax values in MO than in AFS. As found for RL, no SSD was observed in Vcmax (Figure 5A) or Jmax (Figure 5B) during any season (Supplementary Table 3).
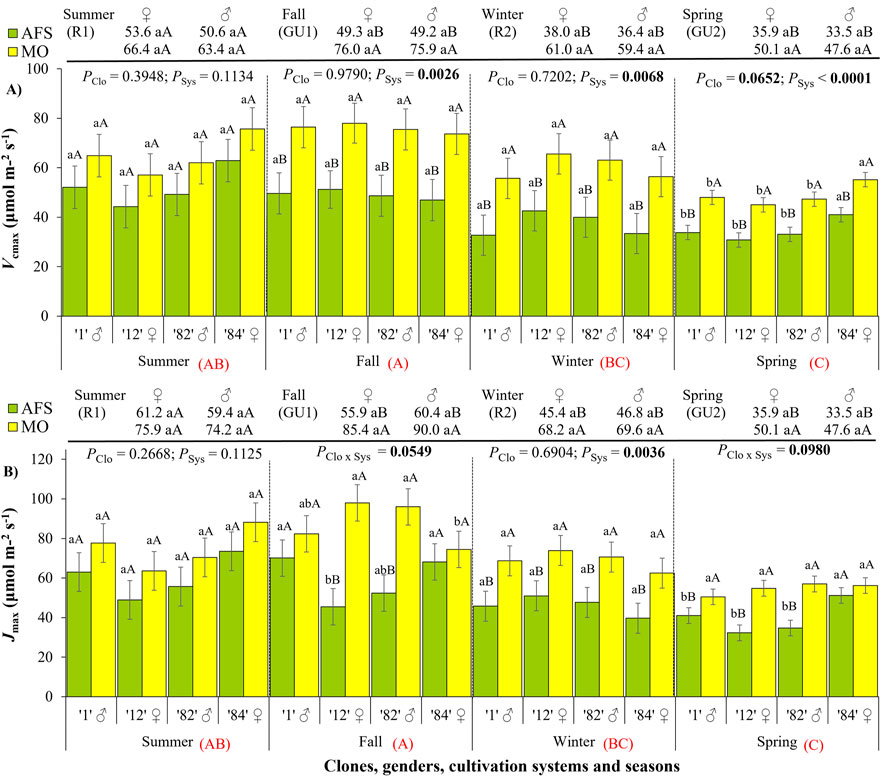
Figure 5. Biochemical parameters derived from yerba mate light curves: (A) maximum apparent RuBisCO carboxylation rate (Vcmax, μmol m−2 s−1) and (B) maximum apparent rate of electron transport (Jmax, μmol m−2 s−1) estimated in two cultivation systems, monoculture (MO) and agroforestry system (AFS), and four clones [1 (♂), 12 (♀), 82 (♂), and 84 (♀)] in four seasons [summer rest 1 (R1), fall growth unit 1 (GU1), winter rest 2 (R2), and spring growth unit 2 (GU2)]. Estimated mean ± SE and p-values with a significance level of <0.1 (bold when significant) are shown. The second family of comparisons is shown above each graph, comparing two genders (female ♀ and male ♂) in each cultivation system and season. For both families of comparisons, lowercase letters compare clone or gender responses under each cultivation system, while uppercase black letters compare cultivation systems for each clone (n = 3) or gender (n = 6) in each season. The uppercase red letters near each season’s indications compare seasonal responses (p < 0.0001 for both Vcmax and Jmax).
The general effect of seasons on RL/Rd or Jmax/Vcmax ratios was not significant during the annual yerba mate growth (Figure 6; Supplementary Table 1), but both ratios were changed by cultivation systems in all growth periods, with the exception of the fall GU1 period (Supplementary Table 2). Considering the cultivation system effect over the seasons, the RL/Rd was higher in MO than in AFS during the summer and winter rest pauses in all clones (Figure 6A). Clones 1 (♂) and 84 (♀) showed higher RL/Rd under MO than AFS only during the spring GU2 period. The ♀ clone 12 showed similar RL/Rd in both cultivation systems, while the ♂ clone 82 showed higher RL/Rd under AFS than in MO. Such clonal variation suggested that SSD in RL/Rd was not expressed during the spring GU2. On the other hand, SSD was expressed in RL/Rd during the winter R2 (Supplementary Table 3), with higher values in males than in females in both cultivation systems, indicating lower inhibition of light respiration in females than in males.
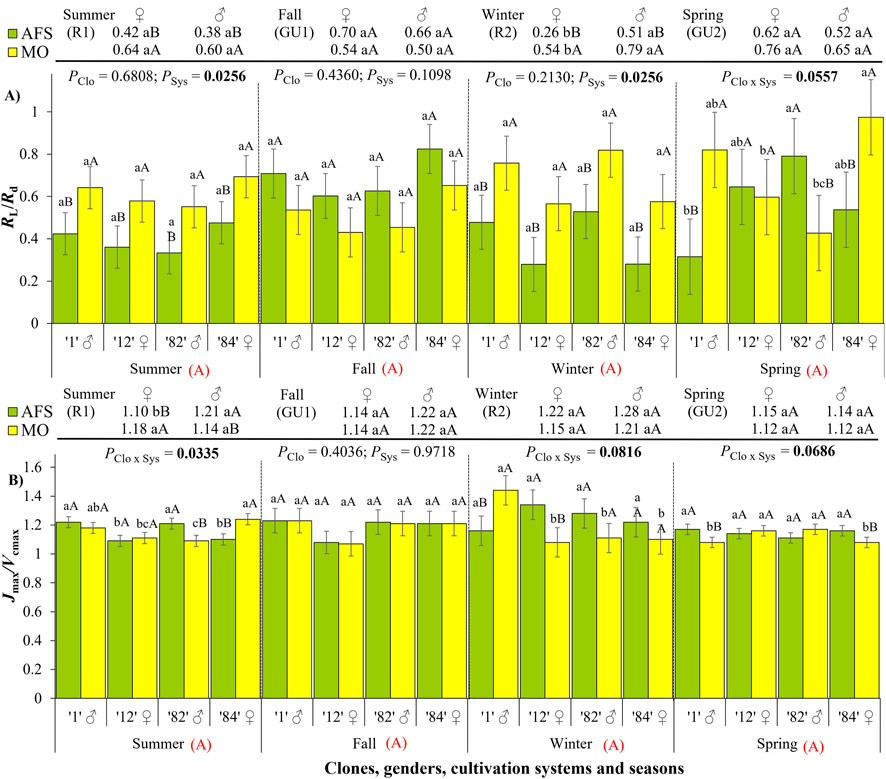
Figure 6. Ratios (A) between the light and dark respirations RL/Rd and (B) between the maximum apparent rate of electron transport and maximum apparent RuBisCO carboxylation rate (Jmax/Vcmax), estimated in two cultivation systems, monoculture (MO) and agroforestry system, (AFS), and four clones [1 (♂), 12 (♀), 82 (♂), and 84 (♀)] in four seasons [summer rest 1 (R1), fall growth unit 1 (GU1), winter rest 2 (R2), and spring growth unit 2 (GU2)]. Estimated mean ± SE and p-values with a significance level of <0.1 (bold when significant) are shown. The second family of comparisons is shown above each graph, comparing two genders (female ♀ and male ♂) in each cultivation system and season. For both families of comparisons, lowercase letters compare clone or gender responses under each cultivation system, while uppercase black letters compare cultivation systems for each clone (n = 3) or gender (n = 6) in each season. The uppercase red letters near the season’s indications compare seasonal responses (not significant for both RL/Rd and Jmax/Vcmax).
In the summer R1, the Jmax/Vcmax ratio was higher in MO than AFS only in ♀ clone 84, while the opposite was found in ♂ clone 82 (Figure 6B; Supplementary Table 2). During the winter R2, Jmax/Vcmax was higher in MO than AFS only in ♂ clone 1, with ♀ clone 12 showing an inverse pattern (AFS > MO). During the summer R1, the males expressed a higher Jmax/Vcmax ratio than females under AFS but not under MO (Supplementary Table 3).
The expression of SSD in photosynthetic performance was observed in six of eleven observed traits, mostly during rest periods (6/24 for traits with SSD expression) than during growth unit formations (3/24). The SSD expression over annual rhythmic growth was not significantly different between the periods of rest and GU formation (Supplementary Table 4).
4 Discussion
This is the first study linking secondary sexual dimorphism, photosynthetic performance, and the annual rhythmic growth of yerba mate under field conditions. In general, yerba mate photosynthetic capacity was modified by the cultivation system and varied over the annual rhythmic growth. In this case study, despite the strong differences in photosynthetic performance induced by the cultivation systems, MO and AFS did not modify the SSD expression, meaning that SSD was expressed parallelly in MO and AFS. In fact, only one exception was observed—males showed higher Jmax/Vcmax than females in AFS but not in MO during the summer rest period (R1). The gender segregation occurred in Chl b (R2 and GU2), Chl a/b, (R2 and GU2), Amax (R1 and GU1), Ф (R2), RL/Rd (R2), and Jmax/Vcmax (R1), but SSD expression frequency was not significantly associated with rest or GU formation periods. In addition, Chl a, Rd, RL, Jmax, and Vcmax did not show any gender segregation over the annual rhythmic growth.
The leaves of higher plant species contain more Chl a than Chl b, and the total Chl is positively correlated with leaf N concentration (Li et al., 2018). Lower total Chl content per unit leaf area and lower Chl a/b ratios are more common in shade species than sun ones (Boardman, 1977) or in shaded compared to sun-exposed leaves of the same species (Lichtenthaler et al., 2007; Zhang et al., 2015). The studied yerba mate clones had lower Chl a under AFS than under MO during the fall growth unit (GU1) period (Figure 2A). Such an environmental effect on Chl a was expressed only in the ♀ clone 12 during the summer rest (R1) and was lost during the winter rest (R2) and spring growth unit (GU2) periods with increasing self-shading of growing plants in MO. Considering the Chl b index, only ♀ clone 12 expressed the difference due to the cultivation system during the winter R2 period. Gender differentiation was expressed for the Chl b index during the winter rest (R2) and spring growth unit (GU2) periods, with females showing higher Chl b index values than males (Figure 2B). SSD in Chl a and b was reported in A. palmeri (Korres et al., 2023), with females exhibiting higher values than males. Under abiotic stress, both Chl a and Chl b decrease, but the Chl a/b ratio tends to increase (Maina and Wang, 2015) due to a greater reduction in Chl b than in Chl a (Ashraf and Harris, 2013). During such chlorophyll degradation, Chl b may also be converted into Chl a (Eckardt, 2009). The higher Chl b index in yerba mate females than males during the R2 and GU2 would suggest that females were less sensitive than males to environmental pressures during the second cycle of plant regrowth after pruning. The Chl a/b ratio declines as irradiance decreases and is higher in trees than in herbs. It is consistent with their adaptation to contrasting irradiance, that is, to the high light of upper forest tree layers vs. the low light of the forest understory (Li et al., 2018). No variation in the Chl a/b ratio was observed in our study due to low or high light conditions promoted by cultivation systems (Figure 2C), but it was higher in males than in females during the winter rest (R2) and spring growth unit (GU2) periods. This was caused by a lower Chl b index in males under both light conditions, indicating that male plants were more stressed than females during R2 and GU2.
The growth of adult yerba mate trees strongly responds to the high-light conditions of MO, showing intensive flushing (Matsunaga et al., 2014; Guédon et al., 2018) and biomass production (Rakocevic et al., 2012; Matsunaga et al., 2014). Adult yerba mate plants cultivated in forest understory differ from those in MO in leaf-area density by up to six times (Rakocevic et al., 2011) and biomass approximately ten times (Rakocevic et al., 2012). In forest understory, the individual leaves are bigger and wider than in MO (Rakocevic et al., 2011). This kind of reaction is typical of forest species classified as “less shade tolerant,” which clearly respond to decreased light intensity (Petriţan et al., 2009). This group of species is characterized by stomata occurrence only on the leaf abaxial epidermis (hypostomatic), as in yerba mate (Rakocevic et al., 2012). The PPFD that attained yerba mate leaf level in the forest understory of AFS was reduced roughly by 92%–95% (depending on daytime) compared to incoming light intensity in MO (Figures 1A, B). Leaf angles over the canopy would improve light use efficiency in AFS while protecting photosynthetic apparatus from high light in MO (Rakocevic et al., 2011; Rakocevic et al., 2024). Even with such structural adjustments, the reduction of Amax in AFS is small compared to the sharp reduction in PPFD. When compared to MO, Amax was reduced between 21% and 45% in fall and winter in AFS, regardless of clones and periods (Figure 3A). This suggests that leaves of yerba mate under AFS could use the sunflecks efficiently as acclimation processes to low light with higher light use efficiency than under MO (Almeida et al., 2022), as found in Fagus sylvatica (Durand et al., 2022). This was supported by lower Rd (Figure 4A) associated with a higher degree of light inhibition of respiration (lower RL/Rd) under AFS than under MO (Figure 6A).
SSD in Amax was expressed during the summer rest (R1) and fall growth unit (GU1) periods (Figure 3A), in different periods than the Chl b and Chl a/b ratio (R2 and GU2, Figures 2B, C). Amax was higher in females than in males (Figure 3A) and occurred in early vegetative stages, as previously observed (Rakocevic et al., 2009; 2011). The gender differentiation was not found in Rd of dioecious P. tremuloides trees (Wang and Curtis, 2001), similarly as happened herein (Figure 5). In yerba mate, Rd and RL were much higher under MO than under AFS during all periods of rhythmic growth (Figure 4). During the growth unit formation (GU2) period, ♀ clone 84 showed a higher respiration rate than other clones in MO but not in AFS. Strong linear correlations have been established among Chl content, Rd, and Ф in various species (Barro et al., 1996). As found for yerba mate, high irradiance increased Rd and Amax in shade-tolerant Glechoma longituba (Zhang et al., 2015). The higher apparent quantum efficiency of CO2 assimilation (Ф) under AFS than under MO (Figure 3B) is one possible cause of no sharp decline in Amax (Figure 3A) or even similar Amax in both cultivation systems (Rakocevic et al., 2024). Regarding the inhibition of respiration by light (a low RL/Rd ratio means a high degree of inhibition), it was generally more pronounced under low light (AFS) than under high light (MO) conditions, except for clone 82 during the spring GU2 (Figure 6A). The RL/Rd ratio also varied between genders during the winter rest (R2) period, showing higher values in males than in females. In the third year of plant life without pruning, during the sixth growth unit formation (GU6), females show higher RL/Rd ratios than males (Rakocevic et al., 2024), indicating that the SSD expressions in the same physiological trait vary with ontogenic age and rhythmic growth. The inhibition of respiration by light varies over the active vegetation period in Quercus rubra, with higher inhibition occurring in the middle of the growing season (high temperatures) and lower inhibition in the early and late season (mild temperatures) (Heskel and Tang, 2018). In our experiment, the general values of RL/Rd did not vary over the seasons, probably because of yerba mate’s origin and cultivation in a subtropical climate, where temperature variations among the seasons were not as large (Figures 1C, D) as they are in temperate climates (Heskel and Tang, 2018). Additionally, given the relatively small sample size, large errors associated with certain parameters (as RL and Rd, Figure 4), and the high p-value <0.1 used to determine significance, a larger scale study is needed to more conclusively observe SSD within yerba mate.
Higher Vcmax and Jmax values are found in upper sunlit canopy layers than in the lower shaded layers (Bachofen et al., 2022). N allocation to RuBisCO and then high Vcmax represents a physiological acclimation to high-light availability (Grassi and Bagnaresi, 2001). Considerable variations in the Vcmax and Jmax of sunlit leaves throughout growing seasons are found in deciduous forests at various altitudes, where Vcmax increases in summer and declines along with leaf senescence during the fall season (Song et al., 2021). Herein, both Vcmax and Jmax were always higher in yerba mate under MO than under AFS (with the exception of the summer rest (R1) period), decreasing over time after pruning from R1 to GU2 (Figure 5). We found SSD expression when considering Jmax/Vcmax, with higher ratios in males than in females in the summer rest (R1) period. The Jmax/Vcmax represents the coordination of resource investment in electron transport for RuBP regeneration and in RuBisCO activity in the Calvin–Benson cycle (Walker et al., 2014). The Jmax/Vcmax ratio was generally higher under AFS than MO, but some clones were not sensitive or even had inverse responses to a light environment, as ♀ clone 84 during the summer rest (R1) or ♂ clone 1 during the winter rest (R2) period (Figure 6B). The Jmax/Vcmax ratio was the only parameter among those studied herein that was modified by SSD under two extreme light conditions (cultivation systems), indicating higher investment in electron transport for RuBP regeneration in males than females only under AFS during the summer rest R1.
In general, the more the clonal difference was expressed, the more probable the SSD expression was observed in physiological features, as viewed in Chl b, Chl a/b, Amax, ϕ, and Jmax/Vcmax. However, no association was established between rhythmic growth and SSD occurrence. No stable expression of higher values of physiological traits relative to one gender over time and seasons is observed in various dioecious trees (Correia and Barradas, 2000; Dawson et al., 2004; Han et al., 2013; Robakowski et al., 2018; Wu et al., 2021). The responses for one annual growth period are not necessarily the general rule for SSD expression, which can vary with ontogenic age and growing conditions. In addition, SDD did not affect the responses of yerba mate to cultivation systems, which is likely because dioecious species show a division of labor for resource acquisition (Galfrascoli and Calviño, 2020; Rakocevic et al., 2023b), indicating similar responses of two genders to two different cultivation systems. The gender segregation observed herein can be considered as a fitness strategy by the female plants in their effort to reproduce and maintain plant structure and functioning, suggested by higher Amax values and similar respiration rates (Rd and RL) as males in the early phases after pruning (herein the summer rest R1 and fall growth GU1 periods).
5 Conclusion
We hypothesized that photosynthetic performance would be much higher under monoculture (MO, high light) than under an agroforestry system (AFS, low light) over annual growth and that secondary sexual dimorphism (SSD) would be more expressed during rest than during growth periods. In fact, photosynthetic traits derived from light response curves generally revealed higher photosynthetic performance under the high-light (MO) than low-light conditions (AFS), which varied over the rhythmic growth in yerba mate. Lower Amax and Rd in all clones under AFS as compared to MO occurred together with higher inhibition of respiration by light (low RL/Rd) under AFS than under MO. Despite its strong effect on photosynthetic traits, cultivation systems did not affect the SSD expression frequency in photosynthetic performance, with the exception of the Jmax/Vcmax ratio expressed only under AFS. The SSD was expressed in a higher Chl b index and lower Chl a/b ratio in females than males, which indicates lower sensitivity of females to extreme light conditions of two cultivation systems than males during the winter rest (R2) and the spring growth unit (GU2) formation periods. Higher Amax and similar respiration rates (Rd and RL) in early vegetative phases after pruning (herein R1 and GU1) in females than in males could be considered a fitness strategy of female plants in their additional reproduction efforts, investing more carbon in flower, fruit, and seed production in the following phenophases. Our case study additionally underlines the importance of the period in which species with rhythmic growth are evaluated.
Data availability statement
The raw data supporting the conclusions of this article will be made available by the authors, without undue reservation.
Author contributions
MR: conceptualization, data curation, formal analysis, funding acquisition, investigation, methodology, project administration, resources, supervision, validation, visualization, writing–original draft, and writing–review and editing. EB: data curation, investigation, methodology, resources, and writing–review and editing. RA: investigation and writing–review and editing. IW: funding acquisition, project administration, resources, and writing–review and editing. RR: investigation, resources, and writing–review and editing.
Funding
The author(s) declare that financial support was received for the research, authorship, and/or publication of this article. This study was supported by the National Council for Scientific and Technological Development (CNPq, Brazil) with the fellowship for Invited Researcher awarded to Miroslava Rakocevic (350509/2020-4). Rafael Vasconcelos Ribeiro is also a CNPq fellow (3042950/2022-1).
Acknowledgments
To Dr. Mário Dobner, Dr. Kelen Haygert Lencina, and undergraduate student Ana Clara Dondoerfer Teixeira from UFSC, Brazil, for assisting us in data collection and maintenance of the experimental field, and to Dr. Ruben D. Molina from the University of Iowa, United States, for assisting in statistics.
Conflict of interest
The authors declare that the research was conducted in the absence of any commercial or financial relationships that could be construed as a potential conflict of interest.
The author(s) declared that they were an editorial board member of Frontiers, at the time of submission. This had no impact on the peer review process and the final decision.
Generative AI statement
The author(s) declare that no Generative AI was used in the creation of this manuscript.
Publisher’s note
All claims expressed in this article are solely those of the authors and do not necessarily represent those of their affiliated organizations, or those of the publisher, the editors, and the reviewers. Any product that may be evaluated in this article, or claim that may be made by its manufacturer, is not guaranteed or endorsed by the publisher.
Supplementary material
The Supplementary Material for this article can be found online at: https://www.frontiersin.org/articles/10.3389/fphbi.2024.1501826/full#supplementary-material
Abbreviations
AFS, agroforestry system; Amax, maximum net photosynthesis; Ф, apparent quantum efficiency of CO2 assimilation; Chl a, chlorophyll a; Chl b, chlorophyll b; GU1 and GU2, growth units 1 and 2; Jmax, maximum apparent rate of electron transport; MO, monoculture; PPFD, photosynthetic photon flux density; R1 and R2, rest periods 1 and 2; Rd, dark respiration; RL, light respiration; SSD, secondary sexual dimorphism; Vcmax, maximum apparent RuBisCO carboxylation rate.
References
Aguiar, N. S., Gabira, M. M., Duarte, M. M., de Cássia Tomasi, J., Hansel, F. A., Lavoranti, O. J., et al. (2024). How shading levels affect bioactive compounds in leaves of yerba mate clones. Biochem. Syst. Ecol. 113, 104796. doi:10.1016/j.bse.2024.104796
Almeida, R. L., Silveira, N. M., Miranda, M. T., Pacheco, V. S., Cruz, L. P., Xavier, M. A., et al. (2022). Evidence of photosynthetic acclimation to self-shading in sugarcane canopies. Photosynthetica 60, 521–528. doi:10.32615/ps.2022.045
Alvares, A. A., Stape, J. L., Sentelhas, P. C., Gonçalves, J. L. M., and Sparovek, G. (2014). Köppen’s climate classification map for Brazil. Meteorol. Z 22 (6), 711–728. doi:10.1127/0941-2948/2013/0507
Ashraf, M., and Harris, P. J. C. (2013). Photosynthesis under stressful environments: an overview. Photosynthetica 51 (2), 163–190. doi:10.1007/s11099-013-0021-6
Bachofen, C., Hülsmann, L., Revill, A., Buchmann, N., and D'Odorico, P. (2022). Accounting for foliar gradients in Vcmax and Jmax improves estimates of net CO2 exchange of forests. Agric. For. Meteorol. 314, 108771. doi:10.1016/j.agrformet.2021.108771
Barro, F., González-Fontes, A., and Maldonado, J. M. (1996). Relation between photosynthesis and dark respiration in cereal leaves. J. Plant Physiol. 149 (1–2), 64–68. doi:10.1016/S0176-1617(96)80174-X
Bastos, D. H. M., Oliveira de, D. M., Matsumoto, R. L. T., Carvalho, P. de O., and Ribeiro, M. L. (2007). Yerba maté: pharmacological properties, research and biotechnology. Med. Aromatic Plant Sci. Biotechnol. 1 (1), 37–46. Available at: https://www.scienceopen.com/document?vid=3bcbb24f-78c0-4481-a3bb-9857999ab96c.
Bernacchi, C. J., Portis, A. R., Nakano, H., von Caemmerer, S., and Long, S. P. (2002). Temperature response of mesophyll conductance Implications for the determination of Rubisco enzyme kinetics and for limitations to photosynthesis in vivo. Plant Physiol. 130, 1992–1998. doi:10.1104/pp.008250
Boardman, N. K. (1977). Comparative photosynthesis of sun and shade plants. Annu. Rev. Plant Physiol. 28, 355–377. doi:10.1146/annurev.pp.28.060177.002035
Buffo, R. (2017). “Yerba mate: chemistry, technology, and biological properties,” in Exploring the nutrition and health benefits of functional foods. Editors H. U. Shekhar, Z. H. Howlader, and Y. Kabir, 185–194. doi:10.4018/978-1-5225-0591-4.ch009
Carvalho, P. E. R. (2003). Espécies arbóreas brasileiras. 1 Edn. Colombo, PR: Embrapa Florestas, 455–466.
Correia, O., and Barradas, M. C. D. (2000). Ecophysiological differences between male and female plants of Pistacia lentiscus L. Plant Ecol. 149, 131–142. doi:10.1023/A:1026588326204
Croge, C. P., Cuquel, F. L., and Pintro, P. T. M. (2021). Yerba mate: cultivation systems, processing and chemical composition. A review. Sci. Agric. 78 (5), e20190259. doi:10.1590/1678-992X-2019-0259
da Silva, J. R., Patterson, A. E., Rodrigues, W. P., Campostrini, E., and Griffin, K. L. (2017). Photosynthetic acclimation to elevated CO2 combined with partial root zone drying results in improved water use efficiency, drought tolerance and leaf carbon balance of grapevines (Vitis labrusca). EEB 134, 82–95. doi:10.1016/j.envexpbot.2016.11.007
da Silva, J. R., Rodrigues, W. P., Ferreira, L. S., Bernado, W. P., Paixão, J. S., Patterson, A. E., et al. (2018). Deficit irrigation and transparent plastic covers can save water and improve grapevine cultivation in the tropics. Agric. Water Manag. 202, 66–80. doi:10.1016/j.agwat.2018.02.013
Dawson, T. E., Ward, J. K., and Ehleringer, J. R. (2004). Temporal scaling of physiological responses from gas exchange to tree rings: a gender-specific study of Acer negundo (Boxelder) growing under different conditions. Funct. Ecol. 18 (2), 212–222. doi:10.1111/j.0269-8463.2004.00838.x
de David, P. R., de David, F. A., Toso, J. O., Pasinato, C., Müller, C., Galon, L., et al. (2024). Growth and development of yerba mate seedlings associated with different winter cover species. Braz. J. Sci. 3 (1), 34–42. doi:10.14295/bjs.v3i1.430
Durand, M., Stangl, Z. R., Salmon, Y., Burgess, A. J., Murchie, E. H., and Robson, T. M. (2022). Sunflecks in the upper canopy: dynamics of light-use efficiency in sun and shade leaves of Fagus sylvatica. New Phytol. 235, 1365–1378. doi:10.1111/nph.18222
Eckardt, N. A. (2009). A new chlorophyll degradation pathway. Plant Cell 21, 700. doi:10.1105/tpc.109.210313
Galfrascoli, G. M., and Calviño, A. (2020). Secondary sexual dimorphism in a dioecious tree: a matter of inter-plant variability?. Flora 266, 151595. doi:10.1016/j.flora.2020.151595
Gawron-Gzella, A., Chanaj-Kaczmarek, J., and Cielecka-Piontek, J. (2021). Yerba mate—a long but current history. Nutrients 13, 3706. doi:10.3390/nu13113706
Gonçalves, I. L., and Valduga, A. T. (2023). Trends in Ilex paraguariensis researches: a bibliometric analysis. J. Ethn. Foods 10, 25. doi:10.1186/s42779-023-00193-4
Grassi, G., and Bagnaresi, U. (2001). Foliar morphological and physiological plasticity in Picea abies and Abies alba saplings along a natural light gradient. Tree Physiol. 21, 959–967. doi:10.1093/treephys/21.12-13.959
Guédon, Y., Costes, E., and Rakocevic, M. (2018). Modulation of the yerba-mate metamer production phenology by the cultivation system and the climatic factors. Ecol. Model. 384, 188–197. doi:10.1016/j.ecolmodel.2018.06.020
Hallé, F., and Martin, R. (1968). Étude de la croissance rythmique de l’hévéa (Hevea brasiliensis Müll Arg. Euphorbiacées Crotonoïdées). Adansonia 2-8, 475–503. Available at: https://archive.org/details/biostor-254684.
Hallé, F., Oldemann, R. A. A., and Tomlinson, P. B. (1978). Tropical trees and forests. Berlin: Springer-Verlag, 444. doi:10.1007/978-3-642-81190-6
Han, Y., Wang, L., Zhang, X., Korpelainen, H., and Li, C. (2013). Sexual differences in photosynthetic activity, ultrastructure and phytoremediation potential of Populus cathayana exposed to lead and drought. Tree Physiol. 33 (10), 1043–1060. doi:10.1093/treephys/tpt086
Heskel, M. A., and Tang, J. (2018). Environmental controls on light inhibition of respiration and leaf and canopy daytime carbon exchange in a temperate deciduous forest. Tree Physiol. 38 (12), 1886–1902. doi:10.1093/treephys/tpy103
Kirschbaum, M. U. F., and Farquhar, G. D. (1987). Investigation of the CO2 dependence of quantum yield and respiration in Eucalyptus pauciflora. Plant Physiol. 83, 1032–1036. doi:10.1104/pp.83.4.1032
Kok, B. (1948). A critical consideration of the quantum yield of Chlorella-photosynthesis. Separatum Enzym. 13, 1–56.
Korres, N. E., Norsworthy, J. K., FitzSimons, T., Roberts, T. L., Oosterhuis, D. M., and Govindjee, G. (2023). Evaluation of secondary sexual dimorphism of the dioecious Amaranthus palmeri under abiotic stress. Sci. Rep. 13, 13156. doi:10.1038/s41598-023-40453-6
Li, Y., Liu, C., Zhang, J., Yang, H., Xu, L., Wang, Q., et al. (2018). Variation in leaf chlorophyll concentration from tropical to cold-temperate forests: association with gross primary productivity. Ecol. Indic. 85, 383–389. doi:10.1016/j.ecolind.2017.10.025
Lichtenthaler, H. K., Babani, F., and Langsdorf, G. (2007). Chlorophyll fluorescence imaging of photosynthetic activity in sun and shade leaves of trees. Photosynth. Res. 93, 235–244. doi:10.1007/s11120-007-9174-0
Maina, J. N., and Wang, Q. (2015). Seasonal response of chlorophyll a/b ratio to stress in a typical desert species: Haloxylon ammodendron. Arid. Land Res. Manag. 29 (3), 321–334. doi:10.1080/15324982.2014.980588
Marcheafave, G. G., Pauli, E. D., Wendling, I., Rakocevic, M., Scarminio, I. C., and Bruns, R. E. (2025). A comparative study using UV-Vis, NIR, and FTIR spectral fingerprinting in yerba mate leaves through mixture design extractions and ASCA models. J. Braz. Chem. Soc. 36 (1), e–20240073. doi:10.21577/0103-5053.20240073
Marques, A. de C., dos Reis, M. S., and Denardin, V. F. (2019). Yerba mate landscapes: forest use and socio-environmental conservation. Ambiente and Sociedade 22, e02822. doi:10.1590/1809-4422asoc201702822vu2019L3AO
Matsunaga, F. T., Rakocevic, M., and Brancher, J. D. (2014). Modelling the 3D structure and rhythmic growth responses to environment in dioecious yerba-mate. Ecol. Model. 290, 34–44. doi:10.1016/j.ecolmodel.2013.10.035
Mazuchowski, J. Z., Silva da, E. T., and Junior, A. M. (2007). Effects of light and nitrogen on the growth of Ilex paraguariensis St. Hil. Rev. Árvore 31 (4), 619–627. doi:10.1590/S0100-67622007000400006
Melnikova, N. V., Borkhert, E. V., Snezhkina, A. V., Kudryavtseva, A. V., and Dmitriev, A. A. (2017). Sex-specific response to stress in Populus. Front. Plant Sci. 8, 1827. doi:10.3389/fpls.2017.01827
Penteado Junior, J. F., and Goulart, I. C. G. R. (2019). Erva 20: Sistema de produção de erva-mate Embrapa: Brasília-DF, Brazil. Available at: https://www.embrapa.br/busca-de-publicacoes/-/publicacao/1106677/erva-20-sistema-de-producao-para-erva-mate.
Petersen, P., Tardin, J. M., and Marochi, F. (2000). From mate extractivism to the regenerative management of Araucaria Forest. Heia Newsl. 1, 17–18.
Petriţan, A. M., von Lüpke, B., and Petriţan, I. C. (2009). Influence of light availability on growth, leaf morphology and plant architecture of beech (Fagus sylvatica L.), maple (Acer pseudoplatanus L.) and ash (Fraxinus excelsior L.) saplings. Eur. J. For. Res. 128, 61–74. doi:10.1007/s10342-008-0239-1
R Core Team (2022). R: a language and environment for statistical computing. Vienna, Austria: R Foundation for Statistical Computing. Available at: https://www.R-project.org/.
Rakocevic, M., Batista, E. R., Matsunaga, F. T., Wendling, I., Marcheafave, G. G., Bruns, R. E., et al. (2024). Canopy architecture and diurnal CO2 uptake in male and female clones of yerba-mate cultivated in monoculture and agroforestry. Ann. Appl. Biol. 184 (2), 210–225. doi:10.1111/aab.12874
Rakocevic, M., Costes, E., and Assad, E. D. (2011). Structural and physiological sexual dimorphism estimated from three-dimensional virtual trees of yerba-mate (Ilex paraguariensis) is modified by cultivation environment. Ann. Appl. Biol. 159 (2), 178–191. doi:10.1111/j.1744-7348.2011.00484.x
Rakocevic, M., Janssens, M., and Scherer, R. (2012). “Light responses and gender issues in the domestication process of yerba-mate, a sub-tropical evergreen,” in Evergreens: types, ecology and conservation. Editors A. D. Bezerra,, and T. S. Ferreira (New York: Nova Science Publicher), 63–95.
Rakocevic, M., Maia, A.H. de N., de Liz, M. V., Imoski, R., Helm, C. V., Cardozo Junior, E. L., et al. (2023a). Stability of leaf yerba mate (Ilex paraguariensis) metabolite concentrations over the time from the prism of secondary sexual dimorphism. Plants 12, 2199. doi:10.3390/plants12112199
Rakocevic, M., Maia, A. H. N., Duarte, M. M., and Wendling, I. (2023b). Secondary sexual dimorphism in biomass production of Ilex paraguariensis progenies associated to their provenances and morphotypes. Exp. Agric. 59, e3. doi:10.1017/S0014479722000552
Rakocevic, M., Medrado, M. J. S., Lavoranti, O. J., and Valduga, A. T. (2007). Quality of yerba-mate leaves originating from male and female plants. Pesq. Flor. Bras. 54, 71–83. Available at: https://pfb.cnpf.embrapa.br/pfb/index.php/pfb/article/view/131.
Rakocevic, M., Medrado, M. J. S., Lucambio, F., and Valduga, A. T. (2008). Intensity of bitterness of processed yerba mate leaves originated in two contrasted light environments. Braz. Arch. Biol. Technol. 51 (3), 569–579. doi:10.1590/S1516-89132008000300018
Rakocevic, M., Medrado, M. J. S., Martim, S. F., and Assad, E. D. (2009). Sexual dimorphism and seasonal changes of leaf gas exchange in the dioecious tree Ilex paraguariensis grown in two contrasted cultivation types. Ann. Appl. Biol. 154, 291–301. doi:10.1111/j.1744-7348.2008.00298.x
Robakowski, P., Pers-Kamczyc, E., Ratajczak, E., Thomas, P. A., Ye, Z.-P., Rabska, M., et al. (2018). Photochemistry and antioxidative capacity of female and male Taxus baccata L. acclimated to different nutritional environments. Front. Plant Sci. 9, 742. doi:10.3389/fpls.2018.00742
Schlichting, A. F., Bonfim-Silva, E. M., de Silva, M. C., Pietro-Souza, W., da Silva, T. J. A., and Farias, L. N. (2015). Efficiency of portable chlorophyll meters in assessing the nutritional status of wheat plants. Rev. Bras. Eng. Agric. Ambient. 19 (12), 1148–1151. doi:10.1590/1807-1929/agriambi.v19n12p1148-1151
Song, G., Wang, Q., and Jin, J. (2021). Exploring the instability of the relationship between maximum potential electron transport rate and maximum carboxylation rate in cool-temperate deciduous forests. Agric. For. Meteorol. 308-309, 108614. doi:10.1016/j.agrformet.2021.108614
Sturion, J. A., Stuepp, C. A., and Wendling, I. (2017). Genetic parameters estimates and visual selection for leaves production in Ilex paraguariensis. Bragantia 76 (4), 492–500. doi:10.1590/1678-4499.2016.419
Tomasi, J. de C., Aguiar de, N. S., Duarte, M. M., Gabira, M. M., Vieira, L. M., Pauletti, V., et al. (2024). Nitrogenized fertigation and genotype effects in yerba mate leaf production in a semi-hydroponic system. J. Soil Sci. Plant Nutr. 24, 914–921. doi:10.1007/s42729-023-01595-8
Valduga, A. T., Gonçalves, I. L., Dartora, N., Mielniczki-Pereira, A. A., and Souza de, L. M. (2016). Phytochemical profile of morphologically selected yerba-mate progênies. Ciênc. Agrotec. 40 (1), 114–120. doi:10.1590/S1413-70542016000100011
von Caemmerer, S., and Farquhar, G. D. (1981). Some relationships between the biochemistry of photosynthesis and the gas exchange of leaves. Planta 153, 376–387. doi:10.1007/BF00384257
Walker, A. P., Beckerman, A. P., Gu, L., Kattge, J., Cernusak, L. A., Domingues, T. F., et al. (2014). The relationship of leaf photosynthetic traits - Vcmax and Jmax - to leaf nitrogen, leaf phosphorus, and specific leaf area: a meta-analysis and modeling study. Ecol. Evol. 4, 3218–3235. doi:10.1002/ece3.1173
Wang, X., and Curtis, P. S. (2001). Gender-specific responses of Populus tremuloides to atmospheric CO2 enrichment. New Phytol. 150, 675–684. doi:10.1046/j.1469-8137.2001.00138.x
Wu, X., Liu, J., Meng, Q., Fang, S., Kang, J., and Guo, Q. (2021). Differences in carbon and nitrogen metabolism between male and female Populus cathayana in response to deficient nitrogen. Tree Physiol. 41 (1), 119–133. doi:10.1093/treephys/tpaa108
Keywords: Amax, chlorophyll, Jmax/Vcmax, respiration, yerba mate
Citation: Rakocevic M, Batista ER, de Almeida RL, Wendling I and Ribeiro RV (2025) Expression of secondary sexual dimorphism in photosynthetic performance of Ilex paraguariensis under contrasted light availability of monoculture and agroforestry during annual rhythmic growth. Front. Photobiol. 2:1501826. doi: 10.3389/fphbi.2024.1501826
Received: 25 September 2024; Accepted: 05 December 2024;
Published: 07 January 2025.
Edited by:
Christophe Brunet, Anton Dohrn Zoological Station Naples, ItalyReviewed by:
Justyna Maria Labuz, Jagiellonian University, PolandMichael Gorka, Rensselaer Polytechnic Institute, United States
Copyright © 2025 Rakocevic, Batista, de Almeida, Wendling and Ribeiro. This is an open-access article distributed under the terms of the Creative Commons Attribution License (CC BY). The use, distribution or reproduction in other forums is permitted, provided the original author(s) and the copyright owner(s) are credited and that the original publication in this journal is cited, in accordance with accepted academic practice. No use, distribution or reproduction is permitted which does not comply with these terms.
*Correspondence: Miroslava Rakocevic, bWltYXJha29AdW5pY2FtcC5icg==