- 1Division of Photobiology and Photomedicine, Department of Dermatology, Henry Ford Health, Detroit, MI, United States
- 2Department of Medicine, College of Human Medicine, Michigan State University, East Lansing, MI, United States
- 3Department of Physics and Astronomy, Wayne State University, Detroit, MI, United States
Background: The combination of long wavelength ultraviolet A1 radiation (LWUVA1) and visible light (VL) has been shown to produce photodamage and the majority of organic sunscreens lack protection against this spectrum. Currently, established testing protocols for VL photoprotection are lacking.
Objective: To compare pigmentation assessment methods, including Investigator’s Global Assessment (IGA) scoring, Diffuse Reflectance Spectroscopy (DRS), and immunohistochemistry, to assess the utility of each in evaluating VL + LWUVA1 induced pigmentation.
Methods: Anonymized IGA, DRS, and immunohistochemistry data for 37 subjects (Fitzpatrick skin phototypes IV-VI) was retrospectively analyzed for pigment evaluation. Pigmentation was induced on the subjects’ backs with VL + LWUVA1 (0%–0.5% UVA1) irradiation at a dose of 480 J/cm2. Comparisons were made for all three assessment techniques between non-irradiated skin and VL + LWUVA1 irradiated skin, as well as between pure VL (0% UVA1) and VL + LWUVA1 (0.5% UVA1) irradiated sites. All comparisons were made for data collected approximately 24 h after irradiation to evaluate persistent pigment darkening (PPD).
Results: Among all 37 subjects, both IGA scores and DRS detected a statistically significant difference in PPD between irradiated and non-irradiated sites, as well as between VL + LWUVA1 and pure VL irradiated sites. However, MART-1/Melan-A did not indicate a statistically significant difference in PPD between irradiated and non-irradiated sites or between VL + LWUVA1 and pure VL irradiated sites.
Conclusion: Subjective and objective noninvasive assessments were more sensitive in detecting VL + LWUVA1 induced pigmentation and should be preferred over invasive methods. Researchers are advised to initiate assessments with IGA and subsequently incorporate DRS for more objective and comprehensive insights in pigment evaluation.
Introduction
Sunlight is comprised of both ultraviolet (UV) and visible light. Until recently, visible light (VL, 400–700 nm) was believed to minimally impact skin photobiology. (Suh et al., 2007; Duteil et al., 2014). Recent studies, however, have revealed that long wavelength UVA1 (LWUVA1, 370–400 nm) and VL (combination referred to VL + LWUVA1, 370–700 nm) can induce cutaneous effects such as erythema, inflammation, increased ROS formation, and sustained pigmentation. (Cadet et al., 2009; Mahmoud et al., 2010; Ramasubramaniam et al., 2011; Liebel et al., 2012; Duteil et al., 2014; Randhawa et al., 2015; Ezekwe et al., 2022; Pourang et al., 2022).
Organic broad-spectrum sunscreens do not protect against these wavelengths. VL-induced pigmentation occurs in 3 phases: immediate pigment darkening (IPD) occurring within minutes, persistent pigment darkening (PPD) lasting 2–24 h, and delayed tanning (DT) emerging 5–7 days post-irradiation and lasting weeks to months. (Mahmoud et al., 2010; Kohli et al., 2018). IPD and PPD are suggested to result from the redistribution of existing melanin and oxidation, while DT results from new melanin formation. (Mahmoud et al., 2010; Kohli et al., 2018). Studies have shown that exposure to VL induces more persistent and intense effects than exposure to UVA1 alone. (Mahmoud et al., 2010; Ezekwe et al., 2022). UVA 1-induced pigmentation, along with being relatively less intense, was shown to fade within 2 weeks, while that induced by VL lasted throughout the 2 week duration of the study. (Mahmoud et al., 2010). Moreover, erythema was induced by VL irradiation but not by UVA1. (Mahmoud et al., 2010). In addition, prior research has shown that a small amount of long-wavelength LWUVA1 radiation, primarily 370 nm and above, can synergistically enhance the biological impact of VL for both erythema and pigmentation in dark skinned individuals (Kohli et al., 2018). These findings align with known clinical observations for photo-exacerbated conditions such as melasma and post-inflammatory hyperpigmentation, which are most commonly seen in individuals with skin of color. These conditions often flare despite regular use of sunscreens that protect against UV, thus supporting a role for VL in the photobiologic effects (Boukari et al., 2015). Altogether, these highlight the need for photoprotection beyond UV radiation (Lyons et al., 2022).
Currently, standardized guidelines for testing protocols and evaluation of skin responses for VL photoprotection are lacking (Lim et al., 2021). Previous studies have employed various assessment techniques including standard and cross-polarized photography, high-resolution digital camera systems like the Visia®, Reflectance confocal microscopy (RCM), clinical scoring, spectroscopy, histology, and immunohistochemistry (Suh et al., 2007; Henseler, 2022; Duteil et al., 2014; Ezekwe et al., 2022; Pourang et al., 2022). Cross polarization offers advantages over standard photography, by enhancing the visualization of erythema and pigmentation by eliminating surface reflectance (Ezekwe et al., 2022; Oh et al., 2022). High-resolution digital camera systems like the Visia® are now accessible, offering two-dimensional and three-dimensional displays of skin characteristics, including brown marks (Henseler, 2022). 15RCM is a noninvasive imaging technique that enables the evaluation of skin lesions at the cellular level, providing detailed insights into structures such as melanin and hemoglobin (Farabi et al., 2023). However, cross-polarized photos and RCM only provide qualitative visual data and VISIA is limited to spacial extent but does not provide information regarding the intensity of pigmentation (Henseler, 2022; Farabi et al., 2023). Clinical scoring scales, such as the Investigator’s Global Assessment (IGA), measure pigmentation and erythema intensity; however, are not standardized and rely heavily on the investigator’s judgment.
Spectroscopic techniques, such as colorimetry and diffuse reflectance spectroscopy (DRS), provide noninvasive and objective measures. For both these techniques, light is incident on the area of interest and the reflectance spectra is collected by the spectrometer to quantify relative skin hyperpigmentation and erythema intensity (Kortüm et al., 1963). Colorimetry assesses color changes by measuring CIELAB parameters and individual typology angle (ITA), where a greater Δ L and Δ ITA magnitude indicate a greater change in pigmentation (Suh et al., 2007; Kohli et al., 2018; Ezekwe et al., 2022; Kortüm et al., 1963). DRS measures the area under the curve (AUC) for melanin and oxy-hemoglobin concentrations, capturing both pigmentary and erythema changes, with larger AUC values corresponding to darker skin (Kohli et al., 2018; Ezekwe et al., 2022). Furthermore, immunohistochemical markers such as cyclin D1 and cyclooxygenase-2 (COX-2) have been employed to assess proliferation and inflammation respectively, while melanoma antigen recognized by T cells (MART-1), also known as Melan-A, and Fontana Masson have been utilized as markers for pigmentation (Kohli et al., 2020; Ezekwe et al., 2022).
With multiple assessment methods available, the objective of this study was to evaluate and compare the utility of three different techniques for assessing pigmentation, specifically IGA scoring for hyperpigmentation, DRS measured AUC, and MART-1 staining.
Materials and methods
A retrospective analysis was conducted to compare the sensitivity of three pigment evaluation methods, specifically IGA, DRS, and MART-1 staining, using anonymized data from 37 subjects with Fitzpatrick skin phototypes (SPT) IV-VI (4 with SPT IV, 21 with SPT V, and 12 with SPT VI) who enrolled in two IRB-approved studies conducted at the Photomedicine and Photobiology Unit within our institution (Mohammad et al., 2019; Kohli et al., 2020). Pigmentation was induced on the subjects’ backs using VL + LWUVA1 (0%–0.5% LWUVA1) irradiation at a dose of 480 J/cm2 over approximately 45 min. Among the 37 subjects, pigmentation was induced with pure VL (0% UVA1) in 22 subjects and with VL + LWUVA1 (0.5% LWUVA1) in 15 subjects. For VL + UVA1, the light source used was Fiber-Lite 180 (Dolan-Jenner Industries, Boxborough, MA) with a GG 395/3-mm and 3 KG5/3-mm (Schott North America, Inc., Duryea, PA). For pure VL, the light source used was MI-150 with an internal infrared (IR) filter (Dolan-Jenner Industries, Boxborough, MA); 2 additional filters, a 3-mm GG400 and a 3-mm KG5 (Schott North America, Inc., Duryea, PA) were placed in front of the lamp to filter out the UV radiation and further decrease the IR transmission, respectively. Both VL + UVA1 and pure VL sources consisted of a 150W EKE lamp. The spectral output of the filtered VL + UVA1 light through a liquid light guide used for irradiation consisted of 0.49% UVA1 (340–400 nm), 99.33% VL (400–700 nm), and 0.18% IR (700–1,600 nm). The spectral output of the filtered pure VL light source through a fiber optic used for irradiation consisted of 0.01% UVA1 (340–400 nm), 97.69% VL (400–700 nm) and 2.31% IR (700–1,600 nm). Irradiated and the adjacent non-irradiated skin were evaluated for PPD approximately 24 h after irradiation using clinical photography, IGA scores for pigmentation, DRS measured AUC, and MART-1 immunohistochemistry staining of biopsies. Punch biopsies measuring 4 mm were performed.
Irradiation protocol included administration of 480 J/cm2 of visible light using the two light sources described above. Cross polarized photography was performed using the TwinFlash® RL Clinical Camera with cross polarized filters (Canfield Scientific, Parsippany, NJ, United States). A 5-point scale was used for IGA scores, with 0 representing absence of pigmentation and 5 representing very intense pigmentation (Table 1). DRS measurements were conducted to collect apparent absorbance spectra within the irradiated site and from adjacent non-irradiated skin. An average of three measurements was obtained. The area under the curve (AUC) of these spectra, from 400–700 nm, was compared between the irradiated site and adjacent non-irradiated skin. For immunohistochemical analysis, the number of cells staining positive for MART-1 was counted by a board-certified dermatologist, using an Olympus BX51 microscope fitted with an Olympus DP71 digital camera (Olympus America, Inc., Center Valley, PA) at ×40 magnification. Positive MART-1 staining cells were counted in five 40x fields and compared between the irradiated site and the non-irradiated skin.
Statistical analysis was conducted using paired samples t-tests to compare differences in pigmentation between the control/non-irradiated skin and irradiated sites for all assessment methods: IGA, DRS, and MART-1 for all subjects. The utility of the three methods was further evaluated by comparing the differences in pigment intensity between the pure VL (0% LWUVA1) and VL + LWUVA1 (0.5% LWUVA1) irradiated sites.
Results
Cross-polarized photographs in Figure 1 represent clinically evident pigmentation with respect to adjacent normal skin induced by 480 J/cm2 of pure VL (Figure 1A) and VL + LWUVA1 (0.5% UVA1) (Figure 1B) approximately 24 h after irradiation, PPD.
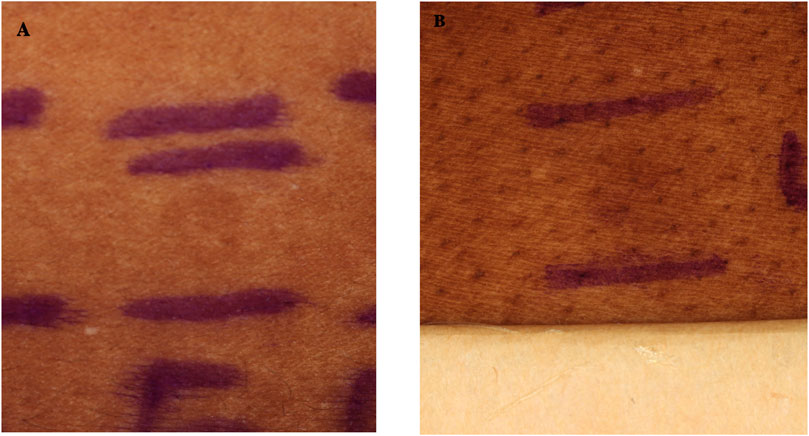
Figure 1. Representative clinical photos of pigmentation induced with pure VL (A) and VL + 0.5% UVA1 (B) in two different patients 24 h after irradiation with a dose of 480 J/cm2. Pigmentation is more intense for VL + UVA1 relative to pure VL.
When comparing all 37 subjects, IGA scores demonstrated a statistically significant difference (p < 0.001) in PPD between irradiated (1.16 +/− 0.12) and non-irradiated skin (0.00 +/− 0.0). This was supported by the objective DRS measured AUC being statistically significant (p < 0.001), with higher AUC values for irradiated skin (308.35 +/− 9.08) compared to non-irradiated skin (281.91 +/- 9.86). While pigmentation was clinically evident, no statistically significant difference (p = 0.326) was demonstrated by MART-1 staining between the irradiated site (36.15 +/− 0.95) and non-irradiated site (36.52 +/− 0.95) (Table 2).
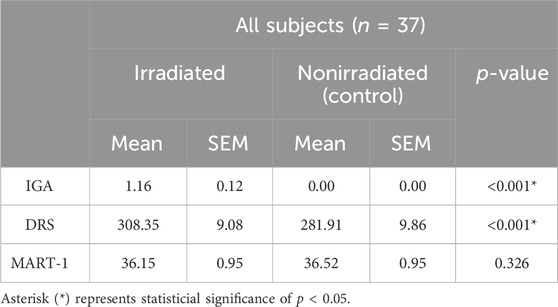
Table 2. Pigmentation evaluation comparison between irradiated and non-irradiated skin usingdifferent assessment methods 24 h post irradiation.
When comparing pigment intensity between sites irradiated with VL + LWUVA1 and pure VL, a statistically significant difference (p < 0.001) in IGA values was observed. The IGA values were higher for VL + LWUVA1 (1.60 +/− 0.16) compared to pure VL (0.86 +/− 0.14). This finding was corroborated by the normalized DRS measured AUC (Δ AUC = AUC of irradiated site-AUC of non-irradiated control) for the two light sources with Δ AUC of 35.83 +/− 4.79 for the VL + LWUVA1 irradiated sites and 20.05 +/− 3.53 for pure VL (statistically significant p = 0.01). MART-1, once again, showed no statistically significant difference in pigmentation between the sites irradiated with these two different light sources (p = 0.25) (Tables 3 and 4).
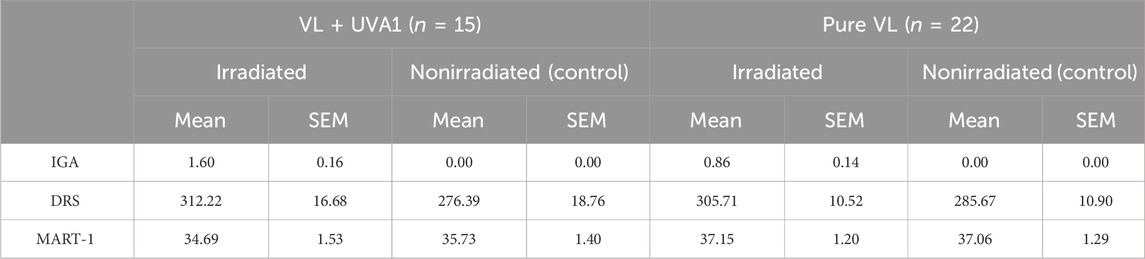
Table 3. Pigmentation evaluation comparison between the two light sources using different assessment methods 24 h post irradiation.
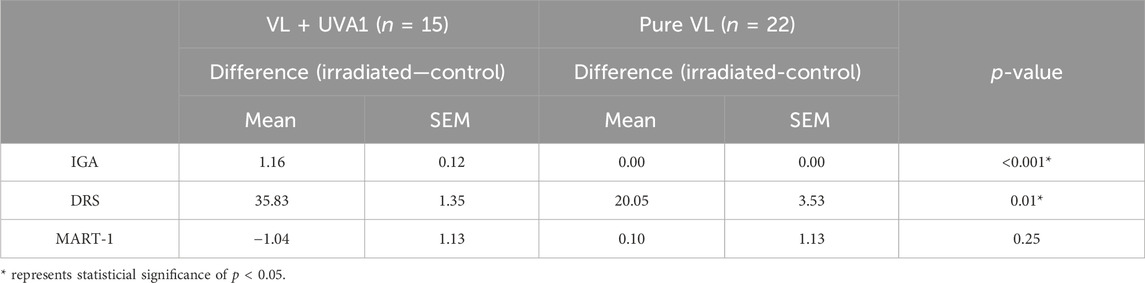
Table 4. Pigmentation evaluation comparing the mean difference (irradiated-control) between the two light sources using different assessment methods 24 h post irradiation, using normalized data.
Discussion
IGA scores and DRS detected a statistically significant difference in PPD between irradiated and non-irradiated sites, as well as between VL + LWUVA1 and pure VL irradiated sites. However, MART-1 staining was not sensitive enough to demonstrate these changes. These results suggest that noninvasive methods are more sensitive in evaluating pigmentary changes than immunohistochemical staining performed after invasive skin biopsies. As such, hyperpigmentation evaluation in dermatologic research should prioritize non-invasive methods, specifically utilizing IGA scoring followed by objective and continuous DRS measurements.
IGA serves as a valuable and quick initial tool for measuring hyperpigmentation. However, its limitations stem from its subjective nature, relying on clinical observation. Variability may be introduced by factors such as lighting conditions or the evaluator’s expertise, even for the most experienced evaluators (Mohammad et al., 2019). To mitigate this associated bias, the same blinded investigator should assess all sites throughout the study. One theorized reason of why IGA was capable of distinguishing between PPD induced by the two light sources could be the absence of continuity between the measured data points. IGA is a five-point scale with large gaps between the score values. As such, to ascertain the validity of the results, it is essential to collect spectroscopic data in addition to this clinical scoring system.
DRS should be utilized in addition to IGA, considering the objective nature and higher sensitivity of this technique relative to IGA. DRS allows for the noninvasive, in-vivo measurement of pigmentation, and is user independent. It quantitatively assesses the melanin and hemoglobin content in the skin (Stamatas et al., 2008; Zonios et al., 2001). Incident light from DRS is partially absorbed by the chromophores present in the skin, such as hemoglobin and melanin (Kortüm et al., 1963). The remaining light that is not absorbed undergoes scattering within the skin tissue, and this light carries information about the tissue’s optical properties (Zonios et al., 2001; (Kortüm et al., 1963). A portion of the scattered light is reflected from the skin surface and subsequently collected by a detector, which measures the intensity of the reflected light at different wavelengths (Kortüm et al., 1963). To analyze the effects of pigmentation, the area under the curve (AUC) is obtained via integration of the absorbance spectrum, and then compared between the irradiated site and control skin site. Unlike IGA, which employs whole numbers from 0 to 5, DRS provides continuous measurements. In this study, the DRS data yielded statistically significant results when comparing irradiated sites to non-irradiated sites as well as VL and VL + LWUVA1 sites, emphasizing its sensitivity in detecting changes in pigmentation.
Lastly, the results demonstrate that invasive methods such immunohistochemical MART-1 staining are inferior to noninvasive measurements for evaluating VL-induced pigmentation. MART-1/Melan-A staining detects melanocytes and is utilized in the diagnostic assessment of melanomas (Hendi et al., 2006). MART-1 protein is a transmembrane structural protein that plays a crucial role in the formation of melanosomes (Beaty et al., 1997; Hendi et al., 2006; Yamaguchi and Hearing, 2014). In the analysis conducted, no statistically significant difference was observed between irradiated and control skin. One plausible explanation could be that biopsies were collected 24 h after irradiation. Studies indicate that pigment formation typically requires 7–10 days, suggesting that more accurate interpretations could be made with biopsies obtained at that time (Henseler, 2022; Farabi et al., 2023). A significant increase in Melan-A-positive cells was reported in a study on day 5 after irradiating patients with skin types I-III with blue light (380 and 480 nm) for 5 consecutive days, resulting in a cumulative dosage of 100 J/cm2 (Kleinpenning et al., 2010). Additionally, another study evaluating the impact of VL irradiation for four consecutive days (120 J/cm2 per day–cumulative dose 480 J/cm2) on subjects with all skin types showed that Melan-A was among the top 10 upregulated markers at 24 h post the last irradiation session time point (Kim et al., 2023). The light source used in that study had 1.5% UVA1, which is greater than that used in our study (maximum 0.5% UVA1). This indicates that while Melan-A was upregulated when the pigmentation response was intense, it was not sensitive enough to detect pigmentary changes when the pigment intensity was subtle though clinically evident.
To conclude, the findings of this work strongly suggest that non-invasive IGA and DRS are the most effective techniques in evaluating changes in VL induced pigmentation, including subtle changes induced 24 h after VL irradiation. Tissue specimen obtained at the same time followed by immunohistochemical staining with MART-1 could not detect these changes and demonstrated limited utility. As such, invasive biopsies may not be the most effective method for quantifying VL induced pigmentation and therefore are not recommended. Limitations of the study include small sample size and data analysis of one specific time-point (24 h) post irradiation.
Data availability statement
The original contributions presented in the study are included in the article/Supplementary material, further inquiries can be directed to the corresponding author.
Ethics statement
The studies involving humans were approved by the Henry Ford Institutional Review Board. The studies were conducted in accordance with the local legislation and institutional requirements. The participants provided their written informed consent to participate in this study.
Author contributions
RB: Writing–review and editing, Writing–original draft. MM: Writing–review and editing, Writing–original draft. MC: Writing–review and editing. IH: Writing–review and editing. HL: Writing–review and editing. TM: Writing–review and editing. IK: Methodology, Investigation, Conceptualization, Writing–review and editing.
Funding
The author(s) declare that no financial support was received for the research, authorship, and/or publication of this article.
Acknowledgments
We would like to acknowledge Sunita Ghosh from Henry Ford Health for performing the statistical analysis.
Conflict of interest
Authors RB, MM, MC, AS, IH, HL, TM, and IK were employed by Henry Ford Health.
The author(s) declared that they were an editorial board member of Frontiers, at the time of submission. This had no impact on the peer review process and the final decision.
Publisher’s note
All claims expressed in this article are solely those of the authors and do not necessarily represent those of their affiliated organizations, or those of the publisher, the editors and the reviewers. Any product that may be evaluated in this article, or claim that may be made by its manufacturer, is not guaranteed or endorsed by the publisher.
References
Beaty, M. W., Fetsch, P., Wilder, A. M., Marincola, F., and Abati, A. (1997). Effusion cytology of malignant melanoma. A morphologic and immunocytochemical analysis including application of the MART-1 antibody. Cancer 81 (1), 57–63. doi:10.1002/(sici)1097-0142(19970225)81:1<57::aid-cncr12>3.0.co;2-b
Boukari, F., Jourdan, E., Fontas, E., Montaudié, H., Castela, E., Lacour, J. P., et al. (2015). Prevention of melasma relapses with sunscreen combining protection against UV and short wavelengths of visible light: a prospective randomized comparative trial. J. Am. Acad. Dermatol 72 (1), 189–190. doi:10.1016/j.jaad.2014.08.023
Cadet, J., Douki, T., Ravanat, J. L., and Di Mascio, P. (2009). Sensitized formation of oxidatively generated damage to cellular DNA by UVA radiation. Photochem Photobiol. Sci. 8 (7), 903–911. doi:10.1039/b905343n
Duteil, L., Cardot-Leccia, N., Queille-Roussel, C., Maubert, Y., Harmelin, Y., Boukari, F., et al. (2014). Differences in visible light-induced pigmentation according to wavelengths: a clinical and histological study in comparison with UVB exposure. Pigment. Cell Melanoma Res. 27 (5), 822–826. doi:10.1111/pcmr.12273
Ezekwe, N., Maghfour, J., and Kohli, I. (2022). Visible light and the skin. Photochem Photobiol. 98 (6), 1264–1269. doi:10.1111/php.13634
Farabi, B., Khan, S., Jamgochian, M., Atak, M. F., Jain, M., and Rao, B. K. (2023). The role of reflectance confocal microscopy in the diagnosis and management of pigmentary disorders: a review. J. Cosmet. Dermatol 22 (12), 3213–3222. doi:10.1111/jocd.15827
Hendi, A., Brodland, D. G., and Zitelli, J. A. (2006). Melanocytes in long-standing sun-exposed skin: quantitative analysis using the MART-1 immunostain. Arch. Dermatol 142 (7), 871–876. doi:10.1001/archderm.142.7.871
Henseler, H. (2022). Investigation of the precision of the Visia(®) complexion analysis camera system in the assessment of skin surface features. GMS Interdiscip. Plast. Reconstr. Surg. DGPW 11, Doc08. doi:10.3205/iprs000169
Kortüm, G., Braun, W., and Herzog, G. (1963). Principles and techniques of diffuse-reflectance spectroscopy. Ange. Che. Inter. Editi. in Eng. 2 (7), 333–341. doi:10.1002/anie.196303331
Kim, S., Rainer, B. M., Qi, J., Brown, I., Ogurtsova, A., Leung, S., et al. (2023). Clinical and molecular change induced by repeated low-dose visible light exposure in both light-skinned and dark-skinned individuals. Photodermatol. Photoimmunol. Photomed. 39 (3), 204–212. doi:10.1111/phpp.12819
Kleinpenning, M. M., Smits, T., Frunt, M. H., van Erp, P. E., van de Kerkhof, P. C., and Gerritsen, R. M. (2010). Clinical and histological effects of blue light on normal skin. Photodermatol. Photoimmunol. Photomed. 26 (1), 16–21. doi:10.1111/j.1600-0781.2009.00474.x
Kohli, I., Braunberger, T. L., Nahhas, A. F., Mirza, F. N., Mokhtari, M., Lyons, A. B., et al. (2020). Long-wavelength ultraviolet A1 and visible light photoprotection: a multimodality assessment of dose and response. Photochem Photobiol. 96 (1), 208–214. doi:10.1111/php.13157
Kohli, I., Chaowattanapanit, S., Mohammad, T. F., Nicholson, C. L., Fatima, S., Jacobsen, G., et al. (2018). Synergistic effects of long-wavelength ultraviolet A1 and visible light on pigmentation and erythema. Br. J. Dermatol 178 (5), 1173–1180. doi:10.1111/bjd.15940
Liebel, F., Kaur, S., Ruvolo, E., Kollias, N., and Southall, M. D. (2012). Irradiation of skin with visible light induces reactive oxygen species and matrix-degrading enzymes. J. Invest. Dermatol. 132 (7), 1901–1907. doi:10.1038/jid.2011.476
Lim, H. W., Kohli, I., Granger, C., Trullàs, C., Piquero-Casals, J., Narda, M., et al. (2021). Photoprotection of the skin from visible light‒induced pigmentation: current testing methods and proposed harmonization. J. Invest. Dermatol. 141 (11), 2569–2576. doi:10.1016/j.jid.2021.03.012
Lyons, A. B., Zubair, R., Kohli, I., Nahhas, A. F., Braunberger, T. L., Mokhtari, M., et al. (2022). Mitigating visible light and long wavelength UVA1-induced effects with topical antioxidants. Photochem Photobiol. 98 (2), 455–460. doi:10.1111/php.13525
Mahmoud, B. H., Ruvolo, E., Hexsel, C. L., Liu, Y., Owen, M. R., Kollias, N., et al. (2010). Impact of long-wavelength UVA and visible light on melanocompetent skin. J. Invest. Dermatol. 130 (8), 2092–2097. doi:10.1038/jid.2010.95
Mohammad, T. F., Kohli, I., Nicholson, C. L., Treyger, G., Chaowattanapanit, S., Nahhas, A. F., et al. (2019). Oral polypodium leucotomos extract and its impact on visible light-induced pigmentation in human subjects. J. Drugs Dermatol 18 (12), 1198–1203.
Oh, Y., Markova, A., Noor, S. J., and Rotemberg, V. (2022). Standardized clinical photography considerations in patients across skin tones. Br. J. Dermatol 186 (2), 352–354. doi:10.1111/bjd.20766
Pourang, A., Tisack, A., Ezekwe, N., Torres, A. E., Kohli, I., Hamzavi, I. H., et al. (2022). Effects of visible light on mechanisms of skin photoaging. Photodermatol. Photoimmunol. Photomed. 38 (3), 191–196. doi:10.1111/phpp.12736
Ramasubramaniam, R., Roy, A., Sharma, B., and Nagalakshmi, S. (2011). Are there mechanistic differences between ultraviolet and visible radiation induced skin pigmentation? Photochem Photobiol. Sci. 10 (12), 1887–1893. doi:10.1039/c1pp05202k
Randhawa, M., Seo, I., Liebel, F., Southall, M. D., Kollias, N., and Ruvolo, E. (2015). Visible light induces melanogenesis in human skin through a photoadaptive response. PLoS One 10 (6), e0130949. doi:10.1371/journal.pone.0130949
Stamatas, G. N., Zmudzka, B. Z., Kollias, N., and Beer, J. Z. (2008). In vivo measurement of skin erythema and pigmentation: new means of implementation of diffuse reflectance spectroscopy with a commercial instrument. Br. J. Dermatol 159 (3), 683–690. doi:10.1111/j.1365-2133.2008.08642.x
Suh, K. S., Roh, H. J., Choi, S. Y., Jeon, Y. S., Doh, K. S., Bae, J. H., et al. (2007). Long-term evaluation of erythema and pigmentation induced by ultraviolet radiations of different wavelengths. Skin. Res. Technol. 13 (2), 154–161. doi:10.1111/j.1600-0846.2007.00213.x
Yamaguchi, Y., and Hearing, V. J. (2014). Melanocytes and their diseases. Cold Spring Harb. Perspect. Med. 4 (5), a017046. doi:10.1101/cshperspect.a017046
Keywords: visible light, ultraviolet A1, photoprotection, threshold dose, diffuse reflectance spectroscopy, hyperpigmentation
Citation: Bardhi R, Mokhtari M, Ceresnie M, Suvarnarkar A, Hamzavi IH, Lim HW, Mohammad TF and Kohli I (2024) Evaluating hyperpigmentation: is invasion of the skin necessary?. Front. Photobiol. 2:1346921. doi: 10.3389/fphbi.2024.1346921
Received: 30 November 2023; Accepted: 26 April 2024;
Published: 24 May 2024.
Edited by:
Tamara Gracia Cazaña, Hospital Universitario Miguel Servet, SpainReviewed by:
Jose-manuel Carrascosa, Hospital Germans Trias i Pujol, SpainJose Aguilera Arjona, University of Malaga, Spain
Catharina M. Lerche, Bispebjerg Hospital, Denmark
Copyright © 2024 Bardhi, Mokhtari, Ceresnie, Suvarnarkar, Hamzavi, Lim, Mohammad and Kohli. This is an open-access article distributed under the terms of the Creative Commons Attribution License (CC BY). The use, distribution or reproduction in other forums is permitted, provided the original author(s) and the copyright owner(s) are credited and that the original publication in this journal is cited, in accordance with accepted academic practice. No use, distribution or reproduction is permitted which does not comply with these terms.
*Correspondence: Indermeet Kohli, aWtvaGxpMUBoZmhzLm9yZw==