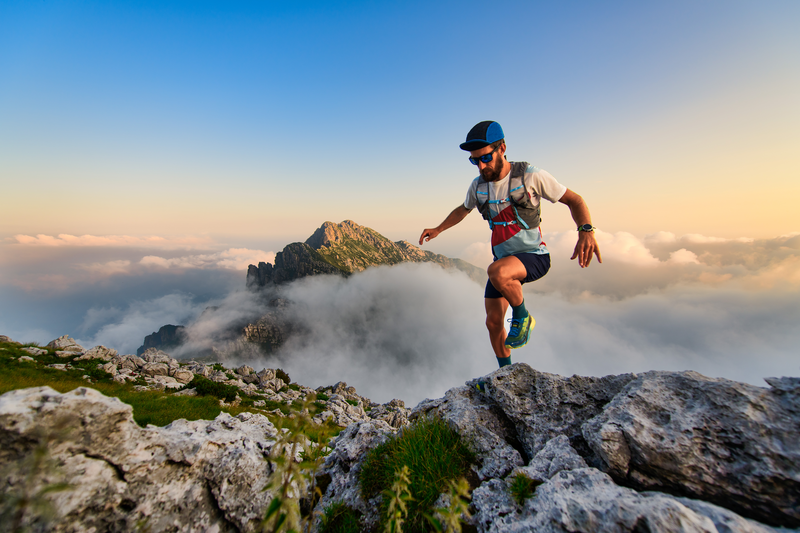
95% of researchers rate our articles as excellent or good
Learn more about the work of our research integrity team to safeguard the quality of each article we publish.
Find out more
REVIEW article
Front. Pharmacol.
Sec. Neuropharmacology
Volume 16 - 2025 | doi: 10.3389/fphar.2025.1591469
The final, formatted version of the article will be published soon.
You have multiple emails registered with Frontiers:
Please enter your email address:
If you already have an account, please login
You don't have a Frontiers account ? You can register here
Mental disorders are complex conditions that encompass various symptoms and types, affecting approximately 1 in 8 people globally. They place a significant burden on both families and society as a whole. So far, the etiology of mental disorders remains poorly understood, making diagnosis and treatment particularly challenging. Extracellular vesicles (EVs) are nanoscale particles produced by cells and released into the extracellular space. They contain bioactive molecules including nucleotides, proteins, lipids, and metabolites, which can mediate intercellular communication and are involved in various physiological and pathological processes. Recent studies have shown that EVs are closely linked to mental disorders like schizophrenia, major depressive disorder, and bipolar disorder, playing a key role in their development, diagnosis, prognosis, and treatment. Therefore, based on recent research findings, this paper aims to describe the roles of EVs in mental disorders and summarize their potential applications in diagnosis and treatment, providing new ideas for the future clinical transformation and application of EVs.
Keywords: extracellular vesicles, Mental Disorders, biomarkers, Information crosstalk, therapy
Received: 11 Mar 2025; Accepted: 01 Apr 2025.
Copyright: © 2025 Wang, Liu and Wang. This is an open-access article distributed under the terms of the Creative Commons Attribution License (CC BY). The use, distribution or reproduction in other forums is permitted, provided the original author(s) or licensor are credited and that the original publication in this journal is cited, in accordance with accepted academic practice. No use, distribution or reproduction is permitted which does not comply with these terms.
* Correspondence:
Ying Wang, Tianjin Anding Hospital, Tianjin, China
Disclaimer: All claims expressed in this article are solely those of the authors and do not necessarily represent those of their affiliated organizations, or those of the publisher, the editors and the reviewers. Any product that may be evaluated in this article or claim that may be made by its manufacturer is not guaranteed or endorsed by the publisher.
Research integrity at Frontiers
Learn more about the work of our research integrity team to safeguard the quality of each article we publish.