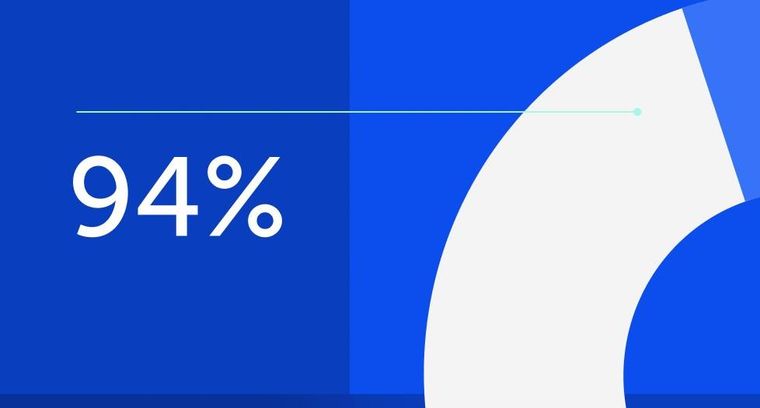
94% of researchers rate our articles as excellent or good
Learn more about the work of our research integrity team to safeguard the quality of each article we publish.
Find out more
MINI REVIEW article
Front. Pharmacol., 27 March 2025
Sec. Pharmacology of Anti-Cancer Drugs
Volume 16 - 2025 | https://doi.org/10.3389/fphar.2025.1585668
This article is part of the Research TopicDecoding Tumor Drug Resistance: Machine Learning’s Role from Molecules to TreatmentView all 9 articles
Brain metastasis in breast cancer (BCBM) significantly threatens the survival and quality of life of patients, particularly those with triple-negative (TNBC) and HER2-positive subtypes. It involves complex molecular mechanisms and diverse signaling pathways. This review highlights recent research on the molecular mechanisms and signaling pathways of BCBM. The process of BCBM includes several key steps: local infiltration of cancer cells into the bloodstream and subsequent spread to the brain. They must then overcome the blood-brain barrier (BBB) to establish and grow in the brain. Multiple signaling pathways, including PI3K/AKT, STAT3, NF-κB, Notch, and Wnt are involved in this process. Overall, BCBM is a complex disease regulated by multiple molecular mechanisms and signaling pathways. To improve patient survival and quality of life, it is crucial to deepen research into the mechanisms of BCBM and explore new treatment targets and strategies. This will enhance our understanding of BCBM and lead to more effective treatments.
Breast cancer (BC) is the second leading cause of cancer-related deaths in women, after lung cancer (Sung et al., 2021). Brain tumors are a significant threat to human life, classified as primary or metastatic, with brain metastases arising from the spread of malignant tumors from other organs (Aldape et al., 2019). The formation of brain metastases is closely linked to BC, and it is a major cause of death in these patients (Sperduto et al., 2020). Approximately 25% of advanced BC patients develop brain metastases, drastically reducing their quality of life and overall survival (OS) (Darlix et al., 2019). BC is heterogeneous, classified into subtypes based on biomarkers like estrogen receptor (ER), progesterone receptor (PR), human epidermal growth factor receptor 2 (HER2), Ki-67, genomic markers (BRCA1, BRCA2, PIK3CA), and immune markers (tumor-infiltrating lymphocytes, PD-L1) (Allison, 2021). HER2-positive BC and triple-negative breast cancer (TNBC) are more prone to brain metastasis than the luminal subtype (Darlix et al., 2019). The median time from diagnosis to brain metastasis is 28–36 months for HER2-positive BC and TNBC, and 47–54 months for the luminal subtype. Once brain metastasis develops, the median OS is about 1 year (Bailleux et al., 2021). Variations in brain metastasis occurrence among BC subtypes complicate treatment strategy choices.
Breast cancer brain metastasis (BCBM) (Wang et al., 2021) involves several sequential steps. First, cancer cells locally infiltrate breast tissue and enter the circulatory system. Next, they overcome the blood-brain barrier (BBB) to access and colonize the brain. Key signaling pathways such as PI3K/AKT, STAT3, and NF-κB regulate cellular growth, invasion, and metastatic capacity throughout this process. The BBB, comprising endothelial cells, pericytes, the basement membrane, and astrocytes, restricts the entry of most anticancer drugs substances and poses a major obstacle in treating BCBM (Belykh et al., 2020), Understanding these steps and associated signaling cascades is crucial for developing targeted therapies.
Primary BC cells initially initiate metastasis and invasion through epithelial-mesenchymal transition (EMT), a pivotal step in cancer progression (Celia-Terrassa and Kang, 2024). During EMT, cells relinquish epithelial characteristics, including cell-cell adhesion, and adopt a mesenchymal phenotype with elongated morphology and enhanced motility (Dongre and Weinberg, 2019). A key event in EMT is the degradation of the basement membrane by enzymes such as metalloproteinases (MMPs), facilitating the invasion of cancer cells (Liao et al., 2024; Wang and Wang, 2022). EMT is not universal among BC cells; it occurs in a subset, indicating metastatic potential is limited to a select group (Pastushenko and Blanpain, 2019). This heterogeneity suggests that the primary tumor comprises cells at various stages of differentiation, each potentially undergoing EMT at different times (Bakir et al., 2020).
The orchestration of EMT involves numerous molecules and signaling cascades (Liang et al., 2023). Transcription factors, especially the Snail family, play a dominant role by downregulating epithelial markers like E-cadherin and promoting mesenchymal traits (Imodoye et al., 2021). The Twist family also contributes to EMT regulation. ZEB1, another crucial transcription factor, collaborates with Snail and Twist and forms an intricate network governing the EMT process (Mohammadi Ghahhari et al., 2022). Snail and Twist suppress E-cadherin expression, a hallmark of EMT, a function also performed by ZEB1 and modulated by pathways like STAT3 (Sadrkhanloo et al., 2022). These factors are clinically linked to BC prognosis. Snail expression is associated with increased recurrence and metastasis, whereas Twist1 correlates with lower survival rates (Saitoh, 2023). ZEB1 overexpression in TNBC and its interaction with the ER underscore its role in promoting aggressive, invasive phenotypes (Mohammadi Ghahhari et al., 2022).
Upon leaving the primary site, tumor cells engage with the extracellular matrix (ECM) and neighboring cells, establishing the tumor microenvironment (TME) (Deng et al., 2024; Zhang X. et al., 2023; Xia Z. et al., 2023; Wang Y. et al., 2022; Li C. et al., 2023). The ECM supports cellular adhesion and migration, housing signaling molecules like cell adhesion proteins and growth factors, facilitating cancer cell migration, invasion, and metastasis (Zhang et al., 2022; Ma et al., 2024) (Wang X. et al., 2022; Soltani et al., 2021). Some cancer cells infiltrate adjacent blood vessels by disrupting the basement membrane and intercellular adhesion, becoming circulating tumor cells (CTCs) (Na et al., 2020). The rise in CTC numbers is a pivotal prognostic indicator linked with distant metastasis survival rates (Pineiro et al., 2020).
Circulating tumor cells (CTCs) face significant challenges in the bloodstream, including immune attacks and hemodynamic stresses, which limit their survival (Na et al., 2020). To evade immune surveillance, CTCs may express PD-L1, which binds to immune cells and inhibits their function (Liu T. et al., 2023; Yilmaz et al., 2023; Wang et al., 2024; Liu et al., 2022). Additionally, CTCs secrete immune-suppressive factors such as TGF-β, impairing immune cell activity (Sanches et al., 2021). They further evade detection by downregulating MHC I molecules, which are essential for presenting tumor antigens to CD8+ T cells (Yamamoto et al., 2020; Xie et al., 2024). Hemodynamic forces also play a critical role in CTC migration and survival. High blood flow velocities expose CTCs to elevated shear stress, potentially leading to mechanical destruction, while low velocities enhance interactions with endothelial cells, promoting extravasation (Taftaf et al., 2021). CTCs can form clusters or associate with platelets, creating CTC-platelet aggregates that protect them from shear stress and natural killer (NK) cell-mediated damage (Donato et al., 2020; Chang et al., 2021; Chi et al., 2022). Platelets also provide CTCs with MHC I molecules, shielding them from cytotoxic T cell attacks (Zhou L. et al., 2023; Zhang J. et al., 2023). Despite these survival mechanisms, tumor cells entering circulation face significant obstacles, and only a subset of CTCs successfully establishes distant metastases.
The brain, being highly vascularized, attracts a significant number of circulating tumor cells (CTCs); however, only a small subset successfully crosses the blood-brain barrier (BBB) to form metastases. The blood-brain barrier is primarily composed of endothelial cells, pericytes, the basement membrane, and astrocytes. It plays a critical role in regulating the passage of substances, ensuring central nervous system homeostasis by selectively permitting the transport of essential nutrients while blocking harmful molecules (Terstappen et al., 2021; Hajal et al., 2021). The blood-brain barrier is essential for maintaining brain function and acts as a robust barrier against cancer cell infiltration. The pre-metastatic microenvironment promotes metastasis through mechanisms such as fibronectin deposition, matrix metalloproteinase expression, and interactions with cytokines and extracellular vesicles (Zhang C. et al., 2024; Li et al., 2024; Yang et al., 2024). Within the central nervous system, interactions between CTCs and local cells create a conducive environment for metastasis. After breaching the BBB, tumor cells trigger reactive astrocytes to release plasminogen activators, initiating an anti-tumor response. While these activators eliminate tumor cells in early metastasis, some cells evade destruction by producing anti-plasminogen activators, such as serpins. In advanced stages, reactive astrocytes contribute to a metastatic microenvironment, supporting tumor progression (Ivanova et al., 2023; Wang J. et al., 2023).
The BBB plays a crucial role in limiting cancer cell colonization and metastasis in the brain. Interactions among CTCs, BBB endothelial cells, secreted cytokines, and central nervous system cells are critical determinants of brain metastasis success.
After breaching the BBB, cancer cells face the challenging task of adapting to the brain microenvironment to establish metastases. Most extravasated cancer cells undergo apoptosis or enter dormancy, with a small fraction resilient to defensive mechanisms, orchestrating vascular remodeling and angiogenesis (Luo et al., 2021). In BC, CTCs activate the HIF-1α signaling cascade to enhance oxygen sensing and cellular adaptation. Additionally, CTCs upregulate anti-apoptotic Bcl-2 family proteins to counter apoptotic signals. However, in brain metastatic lesions of BC, Bcl-2 expression decreases notably compared to primary tumors (Park et al., 2020; Yong et al., 2022). In brain metastasis progression, BC cells activate astrocytes, inducing morphological and functional changes. Activated astrocytes produce chemokines that attract BC cells into the brain parenchyma (Chang et al., 2020). Additionally, astrocytes secrete TGF-β2, which modulates ANGPTL4 expression through SMAD-mediated regulation, facilitating successful colonization (Pedrosa et al., 2018). Growth and angiogenic factors such as VEGF and PDGF promote the formation of new vasculature, providing tumors with ample blood supply (Wang L. et al., 2023). Inflammatory factors are pivotal in diseases progression (Zhai et al., 2024; Xiao et al., 2019; Xiao et al., 2020; Zhang H. et al., 2024; Zhao et al., 2022; Wang et al., 2025). Both CTCs and the brain microenvironment release immune-suppressive factors such as TGF-β and IL-10, inhibiting immune cell activity and impairing their efficacy against tumor cells (Shi et al., 2022). Astrocytes transmit inflammatory cytokines through gap junctions, activating the STAT1 and NF-κB signaling pathways in metastatic cells, promoting proliferation (Zheng et al., 2020). Cancer cells must adapt to the brain’s unique environment to establish brain metastases after crossing the BBB. Astrocytes, growth factors, immune factors, and angiogenesis orchestration play pivotal roles in the evolution of BCBM (Figure 1A).
Figure 1. Signaling Pathways in Breast Cancer Brain Metastasis. (A) The process of brain metastasis. (B) Molecular and signaling pathways.
The PI3K/AKT signaling pathway is activated in 43%–70% of BC patients, enhancing BC cell metastatic potential through increased cell proliferation, invasion, and radiation resistance. The PI3K/AKT pathway plays a crucial role in regulating physiological cell functions like proliferation and migration (Zhai et al., 2023). It is also a major altered pathway in many malignancies. Dysregulation of this pathway is associated with treatment resistance, increased angiogenesis, and invasion. AKT, also known as protein kinase B, has three isoforms: AKT1, AKT2, and AKT3. Despite approximately 80% sequence homology, they often have distinct, sometimes opposing physiological roles. For example, AKT1 decreases cell migration and metastasis formation, whereas AKT2 promotes them. AKT1 is mainly responsible for cell proliferation and survival and also has anti-metastatic effects in BC (Miricescu et al., 2020; Glaviano et al., 2023).
Joanna Kempska et al. used in vitro cell proliferation and migration assays to evaluate the impact of AKT1 knockout (AKT1_KO) and AKT inhibition with Ipatasertib on MDA-MB-231BR cells. Their findings showed that Ipatasertib increased radiosensitivity and reduced cell proliferation in these cells, whereas AKT1 knockout enhanced cell migration, increased clonogenic survival, and decreased radiosensitivity (Kempska et al., 2023). Similarly, Alexandra N. Boix De Jesus et al. demonstrated that Δ133p53β enhances the invasiveness and migratory capabilities of BC cells by activating the AKT pathway, facilitating their traversal of a simulated BBB and increasing the likelihood of brain metastasis (Jesus et al., 2023). Ming Ji et al. found that the PI3K inhibitor XH30 significantly inhibited the proliferation of various brain cancer cells, reduced phosphorylation levels of key proteins in the PI3K signaling pathway, and induced G1 phase cell cycle arrest, while also suppressing tumor growth in a mouse model of lung cancer brain metastasis. Given the prevalence of lung and BC as primary sources of brain metastases, XH30 shows promise as a potential therapeutic for BCBM (Ji et al., 2022). Recent studies highlight the potential efficacy of PI3K inhibitors in treating BCBM, particularly Alpelisib, which is widely studied for its effects on PIK3CA-mutated BC. In the SOLAR-1 trial, Alpelisib combined with the aromatase inhibitor Fulvestrant demonstrated prolonged progression-free survival compared to Fulvestrant alone, especially in patients with PIK3CA mutations (Zhang and Richmond, 2021). Additionally, GDC-0084, a dual PI3K/mTOR inhibitor with significant brain penetration, has shown potential efficacy in clinical trials for PIK3CA-mutated BCBM (Chen et al., 2022).
STAT3, a crucial member of the signal transducer and activator of transcription family, plays a pivotal role in transcriptional regulation within cells. Activated by various extracellular signals such as cytokines and growth factors (Zhang H. et al., 2023), STAT3 translocates to the nucleus and influences the expression of specific genes involved in cell growth, differentiation, apoptosis, and immune responses. This process begins when cytokines like IL-6 bind to their receptors, activating tyrosine kinases such as the JAK family, which then phosphorylate STAT3. Phosphorylated STAT3 forms dimers, migrates to the nucleus, binds to DNA, and regulates target gene transcription (Xia T. et al., 2023; Chen et al., 2024; Zhou JG. et al., 2023). In BC particularly during brain metastasis, abnormal STAT3 activation is closely associated with increased tumor invasiveness and metastatic potential. STAT3 pathway activation enhances tumor cell survival and anti-apoptotic capabilities, aiding adaptation to the hostile brain microenvironment and promoting metastatic lesion formation (Liu et al., 2024).
Zeller et al. found that STAT3 inhibitors significantly reduce BC cell proliferation and migration, especially in astrocyte-conditioned medium simulating the brain environment. This finding underscores the critical role of the STAT3 pathway in regulating BC behavior during brain metastasis (Zeller et al., 2024). Wu et al. revealed that circKIF4A modulates triple-negative breast cancer (TNBC) progression, particularly brain metastasis, via the miR-637/STAT3 axis. Inhibition of circKIF4A significantly suppressed TNBC cell proliferation, migration, and invasion, while STAT3 overexpression reversed these effects. A TNBC xenograft model further confirmed that circKIF4A promotes brain metastasis through this axis (Wu et al., 2024). Targeting the STAT3 pathway has shown promising anti-tumor and anti-metastatic effects in TNBC. For instance, DOXA treatment inhibited STAT3 activation, reducing cell migration and downregulating MMP-2 and MMP-9, key mediators of tumor invasion (Kim et al., 2023). Additionally, reactive astrocytes contribute to drug resistance and brain metastasis in breast cancer (BC) by activating the IL-6/STAT3 pathway. Tamoxifen, by reducing IL-6 expression and STAT3 activation, may counteract brain metastasis by modulating the brain microenvironment (Xu et al., 2020). These findings indicate STAT3 plays a crucial role in BCBM. Thus, targeting the STAT3 pathway has potential clinical value in preventing or treating BCBM.
The NF-κB pathway plays a critical role in breast cancer (BC), particularly in tumor invasiveness and metastasis. Upon activation, NF-κB translocates to the nucleus, where it initiates the transcription of genes involved in cell adhesion, migration, and invasion, thereby promoting tumor progression (Devanaboyina et al., 2022). In BCBM, NF-κB activation is a key mechanism that facilitates immune evasion, enhances tumor cell survival, and supports brain colonization (Sivamaruthi et al., 2023). Studies have demonstrated that overexpression of OR5B21 in BC cells induces epithelial-mesenchymal transition (EMT) via the NF-κB pathway, enhancing invasive and migratory capabilities, particularly in brain metastasis (Li et al., 2021). Similarly, high expression of GBP5 in triple-negative breast cancer (TNBC) correlates with NF-κB activation, promoting invasion and migration, thereby accelerating brain metastasis (Cheng et al., 2021). Furthermore, FAK-mediated activation of the NF-κB pathway enhances cancer cell interactions with the brain microenvironment, increasing survival and growth in the brain. Systemic inhibition of NF-κB has been shown to reduce brain metastasis development, highlighting its potential as a therapeutic target for BCBM (Lorusso et al., 2022).
The NF-κB pathway also contributes to therapy resistance in BCBM. For instance, RNF126 enhances radiation resistance in BC cells by activating NF-κB signaling, and its inhibition with dihydroartemisinin (DHA) significantly increases tumor sensitivity to radiation therapy (Liu W. et al., 2023). Similarly, S100A9 activates the NF-κB pathway through its receptor RAGE, contributing to radiation resistance. Elevated blood levels of S100A9 may serve as a non-invasive biomarker for assessing radiation therapy response, offering a personalized treatment approach for BCBM (Monteiro et al., 2022). These findings underscore the central role of NF-κB in BCBM and suggest that targeting this pathway could provide a promising therapeutic strategy. Moreover, recent studies highlight the complex interplay among the PI3K/AKT, STAT3, and NF-κB pathways in BCBM. PI3K/AKT, a key regulator of tumor progression (Li Z. et al., 2023), interacts with STAT3 and NF-κB to create a pro-metastatic niche. AKT activation promotes STAT3 phosphorylation via mTORC1, enhancing tumor survival and immune evasion (Mo et al., 2024; Marotta et al., 2011).
In BCBM, Notch signaling is increasingly recognized as a critical regulator of tumor cell migration and colonization in the brain (Nam et al., 2008; Qiao et al., 2022). Studies have shown that Notch pathway activation in BC cells enhances BBB disruption, facilitating cancer cell extravasation into the brain parenchyma (Nam et al., 2008). Astrocytes within the brain microenvironment can secrete Notch ligands, further amplifying these signals in metastatic cells (Xing et al., 2013). As a result, BCBM cells gain a selective advantage, manifesting in more aggressive phenotypes and resistance to conventional treatments. In BCBM, aberrant Notch activation has been linked to increased tumor-initiating cell (TIC) populations and therapeutic resistance (Giuli et al., 2019). Besides, Notch signaling can promote the self-renewal of cancer stem-like cells, facilitating their capacity to seed new metastatic lesions in the brain (Giuli et al., 2019). Preclinical evidence suggests that Notch inhibition can reduce the frequency of brain metastatic lesions and sensitize tumor cells to therapies like radiation and chemotherapy (Mollen et al., 2018).
Previous studies have demonstrated that endothelial Wnt/β-catenin signaling plays an essential role in the formation and maintenance of the BBB (Huang et al., 2024), as well as in brain tumorigenesis (Smid et al., 2008). The active WNT/β-catenin signaling contributes to basal breast tumors metastasizing to brain (Fogarty et al., 2005). Thus, the active WNT/β-catenin signaling by BC cells metastasizing to brain could point to mimicry which, if proven, supports the view that the seed grows better in the soil it resembles (Smid et al., 2008). Besides, in BCBM, abnormal Wnt signaling contributes to cancer stemness, enhancing tumor cell self-renewal and resistance to therapy (Kilmister et al., 2022) (Figure 1B; Table 1).
Breast cancer brain metastasis (BCBM) is a severe and life-threatening complication in breast cancer (BC) patients, involving a multi-step process that includes local invasion, circulation, blood-brain barrier (BBB) penetration, and brain colonization. This process is regulated by key signaling pathways such as PI3K/AKT, STAT3, NF-κB, Notch, and Wnt, which influence tumor cell survival, migration, immune evasion, and therapeutic resistance. Understanding the regulatory mechanisms of these pathways in BCBM can elucidate the molecular basis of the disease and provide new therapeutic targets. However, challenges such as the BBB and the complex TME complicate treatment efforts. Current strategies combine systemic and local therapies, with targeted therapies showing promise in some patients. Nevertheless, resistance to treatment remains a significant hurdle, highlighting the need for more effective therapeutic approaches.
Future research on BCBM is expected to focus on multidimensional in vivo and in vitro studies, including epigenetics, to uncover new treatment insights. Advances in single-cell analysis will enhance our understanding of interactions between cancer cells and the brain microenvironment, while biomarker-based research will facilitate early detection and personalized treatment strategies. Additionally, innovative drug delivery systems and nanotherapies hold promise for overcoming the challenges posed by the BBB and TME. In conclusion, BCBM represents a critical complication in BC patients, and its treatment faces numerous challenges. Ongoing research will deepen our understanding of its mechanisms and lay the groundwork for developing more effective therapies to improve patient outcomes.
ZL: Writing–original draft, Writing–review and editing, Conceptualization, Investigation, Methodology. YM: Conceptualization, Investigation, Writing–original draft, Writing–review and editing. YZ: Writing–original draft, Writing–review and editing. YY: Writing–original draft, Writing–review and editing. YJ: Conceptualization, Funding acquisition, Writing–original draft, Writing–review and editing, Resources.
The author(s) declare that financial support was received for the research and/or publication of this article. This study was supported by the National Natural Science Foundation of China (Grant No.81860464); Science Foundation of Guangxi Province (Grant No.2020GXNSFBA297072).
The authors declare that the research was conducted in the absence of any commercial or financial relationships that could be construed as a potential conflict of interest.
The author(s) declare that no Generative AI was used in the creation of this manuscript.
All claims expressed in this article are solely those of the authors and do not necessarily represent those of their affiliated organizations, or those of the publisher, the editors and the reviewers. Any product that may be evaluated in this article, or claim that may be made by its manufacturer, is not guaranteed or endorsed by the publisher.
Aldape, K., Brindle, K. M., Chesler, L., Chopra, R., Gajjar, A., Gilbert, M. R., et al. (2019). Challenges to curing primary brain tumours. Nat. Rev. Clin. Oncol. 16, 509–520. doi:10.1038/s41571-019-0177-5
Allison, K. H. (2021). Prognostic and predictive parameters in breast pathology: a pathologist's primer. Mod. Pathol. 34, 94–106. doi:10.1038/s41379-020-00704-7
Bailleux, C., Eberst, L., and Bachelot, T. (2021). Treatment strategies for breast cancer brain metastases. Br. J. Cancer 124, 142–155. doi:10.1038/s41416-020-01175-y
Bakir, B., Chiarella, A. M., Pitarresi, J. R., and Rustgi, A. K. (2020). EMT, MET, plasticity, and tumor metastasis. Trends Cell Biol. 30, 764–776. doi:10.1016/j.tcb.2020.07.003
Belykh, E., Shaffer, K. V., Lin, C., Byvaltsev, V. A., Preul, M. C., and Chen, L. (2020). Blood-brain barrier, blood-brain tumor barrier, and fluorescence-guided neurosurgical oncology: delivering optical labels to brain tumors. Front. Oncol. 10, 739. doi:10.3389/fonc.2020.00739
Celia-Terrassa, T., and Kang, Y. (2024). How important is EMT for cancer metastasis? PLoS Biol. 22, e3002487. doi:10.1371/journal.pbio.3002487
Chang, G., Shi, L., Ye, Y., Shi, H., Zeng, L., Tiwary, S., et al. (2020). YTHDF3 induces the translation of m(6)a-enriched gene transcripts to promote breast cancer brain metastasis. Cancer Cell 38, 857–871.e7. doi:10.1016/j.ccell.2020.10.004
Chang, P. H., Chen, M. C., Tsai, Y. P., Tan, G. Y. T., Hsu, P. H., Jeng, Y. M., et al. (2021). Interplay between desmoglein2 and hypoxia controls metastasis in breast cancer. Proc. Natl. Acad. Sci. U. S. A. 118, e2014408118. doi:10.1073/pnas.2014408118
Chen, Q., Xiong, J., Ma, Y., Wei, J., Liu, C., and Zhao, Y. (2022). Systemic treatments for breast cancer brain metastasis. Front. Oncol. 12, 1086821. doi:10.3389/fonc.2022.1086821
Chen, Y., Liang, R., Li, Y., Jiang, L., Ma, D., Luo, Q., et al. (2024). Chromatin accessibility: biological functions, molecular mechanisms and therapeutic application. Signal Transduct. Target Ther. 9, 340. doi:10.1038/s41392-024-02030-9
Cheng, S. W., Chen, P. C., Lin, M. H., Ger, T. R., Chiu, H. W., and Lin, Y. F. (2021). GBP5 repression suppresses the metastatic potential and PD-L1 expression in triple-negative breast cancer. Biomedicines 9, 371. doi:10.3390/biomedicines9040371
Chi, H., Xie, X., Yan, Y., Peng, G., Strohmer, D. F., Lai, G., et al. (2022). Natural killer cell-related prognosis signature characterizes immune landscape and predicts prognosis of HNSCC. Front. Immunol. 13, 1018685. doi:10.3389/fimmu.2022.1018685
Darlix, A., Louvel, G., Fraisse, J., Jacot, W., Brain, E., Debled, M., et al. (2019). Impact of breast cancer molecular subtypes on the incidence, kinetics and prognosis of central nervous system metastases in a large multicentre real-life cohort. Br. J. Cancer 121, 991–1000. doi:10.1038/s41416-019-0619-y
Deng, Y., Shi, M., Yi, L., Naveed Khan, M., Xia, Z., and Li, X. (2024). Eliminating a barrier: aiming at VISTA, reversing MDSC-mediated T cell suppression in the tumor microenvironment. Heliyon 10, e37060. doi:10.1016/j.heliyon.2024.e37060
Devanaboyina, M., Kaur, J., Whiteley, E., Lin, L., Einloth, K., Morand, S., et al. (2022). NF-κB signaling in tumor pathways focusing on breast and ovarian cancer. Cancer. Oncol. Rev. 16, 10568. doi:10.3389/or.2022.10568
Donato, C., Kunz, L., Castro-Giner, F., Paasinen-Sohns, A., Strittmatter, K., Szczerba, B. M., et al. (2020). Hypoxia triggers the intravasation of clustered circulating tumor cells. Cell Rep. 32, 108105. doi:10.1016/j.celrep.2020.108105
Dongre, A., and Weinberg, R. A. (2019). New insights into the mechanisms of epithelial-mesenchymal transition and implications for cancer. Nat. Rev. Mol. Cell Biol. 20, 69–84. doi:10.1038/s41580-018-0080-4
Fogarty, M. P., Kessler, J. D., and Wechsler-Reya, R. J. (2005). Morphing into cancer: the role of developmental signaling pathways in brain tumor formation. J. Neurobiol. 64, 458–475. doi:10.1002/neu.20166
Giuli, M., Giuliani, E., Screpanti, I., Bellavia, D., and Sjjoo, C. (2019). Notch signaling activation as a hallmark for triple-negative breast cancer subtype, J. Oncol., 2019:8707053, doi:10.1155/2019/8707053
Glaviano, A., Foo, A. S. C., Lam, H. Y., Yap, K. C. H., Jacot, W., Jones, R. H., et al. (2023). PI3K/AKT/mTOR signaling transduction pathway and targeted therapies in cancer. Mol. Cancer 22, 138. doi:10.1186/s12943-023-01827-6
Hajal, C., Le Roi, B., Kamm, R. D., and Maoz, B. M. (2021). Biology and models of the blood-brain barrier. Annu. Rev. Biomed. Eng. 23, 359–384. doi:10.1146/annurev-bioeng-082120-042814
Huang, X., Wei, P., Fang, C., Yu, M., Yang, S., Qiu, L., et al. (2024). Compromised endothelial Wnt/β-catenin signaling mediates the blood-brain barrier disruption and leads to neuroinflammation in endotoxemia, J. Neuroinflammation, 21:265, doi:10.1186/s12974-024-03261-x
Imodoye, S. O., Adedokun, K. A., Muhammed, A. O., Bello, I. O., Muhibi, M. A., Oduola, T., et al. (2021). Understanding the complex milieu of epithelial-mesenchymal transition in cancer metastasis: new insight into the roles of transcription factors. Front. Oncol. 11, 762817. doi:10.3389/fonc.2021.762817
Ivanova, M., Porta, F. M., Giugliano, F., Frascarelli, C., Sajjadi, E., Venetis, K., et al. (2023). Breast cancer with brain metastasis: molecular insights and clinical management. Genes (Basel) 14, 1160. doi:10.3390/genes14061160
Jesus, A. N. B., Taha, A., Wang, D., Mehta, P. M., Mehta, S., Reily-Bell, A., et al. (2023). Increased expression of the Δ133p53β isoform enhances brain metastasis. Int. J. Mol. Sci. 24, 1267. doi:10.3390/ijms24021267
Ji, M., Wang, D., Lin, S., Wang, C., Li, L., Zhang, Z., et al. (2022). A novel PI3K inhibitor XH30 suppresses orthotopic glioblastoma and brain metastasis in mice models. Acta Pharm. Sin. B 12, 774–786. doi:10.1016/j.apsb.2021.05.019
Kempska, J., Oliveira-Ferrer, L., Grottke, A., Qi, M., Alawi, M., Meyer, F., et al. (2023). Impact of AKT1 on cell invasion and radiosensitivity in a triple negative breast cancer cell line developing brain metastasis. Front. Oncol. 13, 1129682. doi:10.3389/fonc.2023.1129682
Kilmister, E. J., Koh, S. P., Weth, F. R., Gray, C., and Tan, STJB (2022). Cancer metastasis and treatment resistance: mechanistic insights and therapeutic targeting of cancer stem cells and the tumor microenvironment, Biomedicines, 10:2988, doi:10.3390/biomedicines10112988
Kim, S., Park, J. M., Park, S., Jung, E., Ko, D., Park, M., et al. (2023). Suppression of TNBC metastasis by doxazosin, a novel dual inhibitor of c-MET/EGFR. J. Exp. Clin. Cancer Res. 42, 292. doi:10.1186/s13046-023-02866-z
Li, C., Wirth, U., Schardey, J., Ehrlich-Treuenstatt, V. V., Bazhin, A. V., Werner, J., et al. (2023a). An immune-related gene prognostic index for predicting prognosis in patients with colorectal cancer. Front. Immunol. 14, 1156488. doi:10.3389/fimmu.2023.1156488
Li, M., Schweiger, M. W., Ryan, D. J., Nakano, I., Carvalho, L. A., and Tannous, B. A. (2021). Olfactory receptor 5B21 drives breast cancer metastasis. iScience 24, 103519. doi:10.1016/j.isci.2021.103519
Li, Y., Zheng, Y., Tan, X., Du, Y., Wei, Y., and Liu, S. (2024). Extracellular vesicle-mediated pre-metastatic niche formation via altering host microenvironments. Front. Immunol. 15, 1367373. doi:10.3389/fimmu.2024.1367373
Li, Z., Zhou, H., Xia, Z., Xia, T., Du, G., Franziska, S. D., et al. (2023b). HMGA1 augments palbociclib efficacy via PI3K/mTOR signaling in intrahepatic cholangiocarcinoma. Biomark. Res. 11, 33. doi:10.1186/s40364-023-00473-w
Liang, R., Hong, W., Zhang, Y., Ma, D., Li, J., Shi, Y., et al. (2023). Deep dissection of stemness-related hierarchies in hepatocellular carcinoma. J. Transl. Med. 21, 631. doi:10.1186/s12967-023-04425-8
Liao, H., Wang, H., Zheng, R., Yu, Y., Zhang, Y., Lv, L., et al. (2024). LncRNA CARMN suppresses EMT through inhibiting transcription of MMP2 activated by DHX9 in breast cancer. Cell Signal 113, 110943. doi:10.1016/j.cellsig.2023.110943
Liu, S., Li, W., Liang, L., Zhou, Y., and Li, Y. (2024). The regulatory relationship between transcription factor STAT3 and noncoding RNA. Cell Mol. Biol. Lett. 29, 4. doi:10.1186/s11658-023-00521-1
Liu, T., Li, C., Zhang, J., Hu, H., and Li, C. (2023a). Unveiling efferocytosis-related signatures through the integration of single-cell analysis and machine learning: a predictive framework for prognosis and immunotherapy response in hepatocellular carcinoma. Front. Immunol. 14, 1237350. doi:10.3389/fimmu.2023.1237350
Liu, W., Zheng, M., Zhang, R., Jiang, Q., Du, G., Wu, Y., et al. (2023b). RNF126-Mediated MRE11 ubiquitination activates the DNA damage response and confers resistance of triple-negative breast cancer to radiotherapy. Adv. Sci. (Weinh) 10, e2203884. doi:10.1002/advs.202203884
Liu, Y., Cheng, L., Song, X., Li, C., Zhang, J., and Wang, L. (2022). A TP53-associated immune prognostic signature for the prediction of the overall survival and therapeutic responses in pancreatic cancer. Math. Biosci. Eng. 19, 191–208. doi:10.3934/mbe.2022010
Lorusso, G., Wyss, C. B., Kuonen, F., Vannini, N., Billottet, C., Duffey, N., et al. (2022). Connexins orchestrate progression of breast cancer metastasis to the brain by promoting FAK activation. Sci. Transl. Med. 14, eaax8933. doi:10.1126/scitranslmed.aax8933
Luo, L., Liu, P., Zhao, K., Zhao, W., and Zhang, X. (2021). The immune microenvironment in brain metastases of non-small cell lung cancer. Front. Oncol. 11, 698844. doi:10.3389/fonc.2021.698844
Ma, B., Qin, L., Sun, Z., Wang, J., Tran, L. J., Zhang, J., et al. (2024). The single-cell evolution trajectory presented different hypoxia heterogeneity to reveal the carcinogenesis of genes in clear cell renal cell carcinoma: based on multiple omics and real experimental verification. Environ. Toxicol. 39, 869–881. doi:10.1002/tox.24009
Marotta, L. L., Almendro, V., Marusyk, A., Shipitsin, M., Schemme, J., Walker, S. R., et al. (2011). The JAK2/STAT3 signaling pathway is required for growth of CD44⁺CD24⁻ stem cell-like breast cancer cells in human tumors. J. Clin. Invest 121, 2723–2735. doi:10.1172/JCI44745
Miricescu, D., Totan, A., Stanescu, S. I. I., Badoiu, S. C., Stefani, C., and Greabu, M. (2020). PI3K/AKT/mTOR signaling pathway in breast cancer: from molecular landscape to clinical aspects. Int. J. Mol. Sci. 22, 173. doi:10.3390/ijms22010173
Mo, H., Zhang, X., and Ren, L. (2024). Analysis of neuroglia and immune cells in the tumor microenvironment of breast cancer brain metastasis. Cancer Biol. Ther. 25, 2398285. doi:10.1080/15384047.2024.2398285
Mohammadi Ghahhari, N., Sznurkowska, M. K., Hulo, N., Bernasconi, L., Aceto, N., and Picard, D. (2022). Cooperative interaction between ERα and the EMT-inducer ZEB1 reprograms breast cancer cells for bone metastasis. Nat. Commun. 13, 2104. doi:10.1038/s41467-022-29723-5
Mollen, E. W., Ient, J., Tjan-Heijnen, V. C., Boersma, L. J., Miele, L., Smidt, M. L., et al. (2018). Moving breast cancer therapy up a notch. Front. Oncol. 8, 518. doi:10.3389/fonc.2018.00518
Monteiro, C., Miarka, L., Perea-Garcia, M., Priego, N., Garcia-Gomez, P., Alvaro-Espinosa, L., et al. (2022). Stratification of radiosensitive brain metastases based on an actionable S100A9/RAGE resistance mechanism. Nat. Med. 28, 752–765. doi:10.1038/s41591-022-01749-8
Na, T. Y., Schecterson, L., Mendonsa, A. M., and Gumbiner, B. M. (2020). The functional activity of E-cadherin controls tumor cell metastasis at multiple steps. Proc. Natl. Acad. Sci. U. S. A. 117, 5931–5937. doi:10.1073/pnas.1918167117
Nam, D. H., Jeon, H. M., Kim, S., Kim, M. H., Lee, Y. J., Lee, M. S., et al. (2008). Activation of notch signaling in a xenograft model of brain metastasis. Clin. Cancer Res. 14, 4059–4066. doi:10.1158/1078-0432.CCR-07-4039
Park, H. A., Brown, S. R., and Kim, Y. (2020). Cellular mechanisms of circulating tumor cells during breast cancer metastasis. Int. J. Mol. Sci. 21, 5040. doi:10.3390/ijms21145040
Pastushenko, I., and Blanpain, C. (2019). EMT transition states during tumor progression and metastasis. Trends Cell Biol. 29, 212–226. doi:10.1016/j.tcb.2018.12.001
Pedrosa, R., Mustafa, D. A., Soffietti, R., and Kros, J. M. (2018). Breast cancer brain metastasis: molecular mechanisms and directions for treatment. Neuro Oncol. 20, 1439–1449. doi:10.1093/neuonc/noy044
Pineiro, R., Martinez-Pena, I., and Lopez-Lopez, R. (2020). Relevance of CTC clusters in breast cancer metastasis. Adv. Exp. Med. Biol. 1220, 93–115. doi:10.1007/978-3-030-35805-1_7
Qiao, X., Ma, B., Sun, W., Zhang, N., Liu, Y., Jia, L., et al. (2022). JAG1 is associated with the prognosis and metastasis in breast cancer. Sci. Rep. 12, 21986. doi:10.1038/s41598-022-26241-8
Sadrkhanloo, M., Entezari, M., Orouei, S., Ghollasi, M., Fathi, N., Rezaei, S., et al. (2022). STAT3-EMT axis in tumors: modulation of cancer metastasis, stemness and therapy response. Pharmacol. Res. 182, 106311. doi:10.1016/j.phrs.2022.106311
Saitoh, M. (2023). Transcriptional regulation of EMT transcription factors in cancer. Semin. Cancer Biol. 97, 21–29. doi:10.1016/j.semcancer.2023.10.001
Sanches, S. M., Braun, A. C., Calsavara, V. F., Barbosa, P., and Chinen, L. T. D. (2021). Comparison of hormonal receptor expression and HER2 status between circulating tumor cells and breast cancer metastases. Clin. (Sao Paulo) 76, e2971. doi:10.6061/clinics/2021/e2971
Shi, X., Yang, J., Deng, S., Xu, H., Wu, D., Zeng, Q., et al. (2022). TGF-beta signaling in the tumor metabolic microenvironment and targeted therapies. J. Hematol. Oncol. 15, 135. doi:10.1186/s13045-022-01349-6
Sivamaruthi, B. S., Raghani, N., Chorawala, M., Bhattacharya, S., Prajapati, B. G., Elossaily, G. M., et al. (2023). NF-κB pathway and its inhibitors: a promising frontier in the management of alzheimer's disease. Biomedicines 11, 2587. doi:10.3390/biomedicines11092587
Smid, M., Wang, Y., Zhang, Y., Sieuwerts, A. M., Yu, J., Klijn, J. G., et al. (2008). Subtypes of breast cancer show preferential site of relapse. Cancer Res. 68, 3108–3114. doi:10.1158/0008-5472.CAN-07-5644
Soltani, M., Zhao, Y., Xia, Z., Ganjalikhani Hakemi, M., and Bazhin, A. V. (2021). The importance of cellular metabolic pathways in pathogenesis and selective treatments of hematological malignancies. Front. Oncol. 11, 767026. doi:10.3389/fonc.2021.767026
Sperduto, P. W., Mesko, S., Li, J., Cagney, D., Aizer, A., Lin, N. U., et al. (2020). Survival in patients with brain metastases: summary report on the updated diagnosis-specific graded prognostic assessment and definition of the eligibility quotient. J. Clin. Oncol. 38, 3773–3784. doi:10.1200/JCO.20.01255
Sung, H., Ferlay, J., Siegel, R. L., Laversanne, M., Soerjomataram, I., Jemal, A., et al. (2021). Global cancer statistics 2020: GLOBOCAN estimates of incidence and mortality worldwide for 36 cancers in 185 countries. CA Cancer J. Clin. 71, 209–249. doi:10.3322/caac.21660
Taftaf, R., Liu, X., Singh, S., Jia, Y., Dashzeveg, N. K., Hoffmann, A. D., et al. (2021). ICAM1 initiates CTC cluster formation and trans-endothelial migration in lung metastasis of breast cancer. Nat. Commun. 12, 4867. doi:10.1038/s41467-021-25189-z
Terstappen, G. C., Meyer, A. H., Bell, R. D., and Zhang, W. (2021). Strategies for delivering therapeutics across the blood-brain barrier. Nat. Rev. Drug Discov. 20, 362–383. doi:10.1038/s41573-021-00139-y
Wang, J., Zuo, Z., Yu, Z., Chen, Z., Tran, L. J., Zhang, J., et al. (2023a). Collaborating single-cell and bulk RNA sequencing for comprehensive characterization of the intratumor heterogeneity and prognostic model development for bladder cancer. Aging (Albany NY) 15, 12104–12119. doi:10.18632/aging.205166
Wang, J. F., Wang, J. S., Liu, Y., Ji, B., Ding, B. C., Wang, Y. X., et al. (2025). Knockdown of integrin β1 inhibits proliferation and promotes apoptosis in bladder cancer cells. Biofactors 51, e2150. doi:10.1002/biof.2150
Wang, L., Liu, W. Q., Broussy, S., Han, B., and Fang, H. (2023b). Recent advances of anti-angiogenic inhibitors targeting VEGF/VEGFR axis. Front. Pharmacol. 14, 1307860. doi:10.3389/fphar.2023.1307860
Wang, X., Zhao, Y., Strohmer, D. F., Yang, W., Xia, Z., and Yu, C. (2022b). The prognostic value of MicroRNAs associated with fatty acid metabolism in head and neck squamous cell carcinoma. Front. Genet. 13, 983672. doi:10.3389/fgene.2022.983672
Wang, Y., Wang, J., Liu, J., and Zhu, H. (2024). Immune-related diagnostic markers for benign prostatic hyperplasia and their potential as drug targets. Front. Immunol. 15, 1516362. doi:10.3389/fimmu.2024.1516362
Wang, Y., and Wang, X. (2022). A pan-cancer analysis of heat-shock protein 90 Beta1(HSP90B1) in human tumours. Biomolecules 12, 1377. doi:10.3390/biom12101377
Wang, Y., Ye, F., Liang, Y., and Yang, Q. (2021). Breast cancer brain metastasis: insight into molecular mechanisms and therapeutic strategies. Br. J. Cancer 125, 1056–1067. doi:10.1038/s41416-021-01424-8
Wang, Y., Zhu, H., and Wang, X. (2022a). Prognosis and immune infiltration analysis of endoplasmic reticulum stress-related genes in bladder urothelial carcinoma. Front. Genet. 13, 965100. doi:10.3389/fgene.2022.965100
Wu, S., Lu, J., Zhu, H., Wu, F., Mo, Y., Xie, L., et al. (2024). A novel axis of circKIF4A-miR-637-STAT3 promotes brain metastasis in triple-negative breast cancer. Cancer Lett. 581, 216508. doi:10.1016/j.canlet.2023.216508
Xia, T., Zhang, M., Lei, W., Yang, R., Fu, S., Fan, Z., et al. (2023b). Advances in the role of STAT3 in macrophage polarization. Front. Immunol. 14, 1160719. doi:10.3389/fimmu.2023.1160719
Xia, Z., Chen, S., He, M., Li, B., Deng, Y., Yi, L., et al. (2023a). Editorial: targeting metabolism to activate T cells and enhance the efficacy of checkpoint blockade immunotherapy in solid tumors. Front. Immunol. 14, 1247178. doi:10.3389/fimmu.2023.1247178
Xiao, J., Huang, K., Lin, H., Xia, Z., Zhang, J., Li, D., et al. (2020). Mogroside II(E) inhibits digestive enzymes via suppression of interleukin 9/interleukin 9 receptor signalling in acute pancreatitis. Front. Pharmacol. 11, 859. doi:10.3389/fphar.2020.00859
Xiao, J., Lin, H., Liu, B., Xia, Z., Zhang, J., and Jin, J. (2019). Decreased S1P and SPHK2 are involved in pancreatic acinar cell injury. Biomark. Med. 13, 627–637. doi:10.2217/bmm-2018-0404
Xie, H., Xi, X., Lei, T., Liu, H., and Xia, Z. (2024). CD8(+) T cell exhaustion in the tumor microenvironment of breast cancer. Front. Immunol. 15, 1507283. doi:10.3389/fimmu.2024.1507283
Xing, F., Kobayashi, A., Okuda, H., Watabe, M., Pai, S. K., Pandey, P. R., et al. (2013). Reactive astrocytes promote the metastatic growth of breast cancer stem-like cells by activating Notch signalling in brain. EMBO Mol. Med. 5, 384–396. doi:10.1002/emmm.201201623
Xu, Y., Zhu, Y., Yue, Y., Pu, S., Wu, J., Lv, Y., et al. (2020). Tamoxifen attenuates reactive astrocyte-induced brain metastasis and drug resistance through the IL-6/STAT3 signaling pathway. Acta Biochim. Biophys. Sin. (Shanghai) 52, 1299–1305. doi:10.1093/abbs/gmaa127
Yamamoto, K., Venida, A., Yano, J., Biancur, D. E., Kakiuchi, M., Gupta, S., et al. (2020). Autophagy promotes immune evasion of pancreatic cancer by degrading MHC-I. Nature 581, 100–105. doi:10.1038/s41586-020-2229-5
Yang, H., Li, Z., Zhu, S., Wang, W., Zhang, J., Zhao, D., et al. (2024). Molecular mechanisms of pancreatic cancer liver metastasis: the role of PAK2. Front. Immunol. 15, 1347683. doi:10.3389/fimmu.2024.1347683
Yilmaz, M., Kaplan, F., Mender, I., Gryaznov, S. M., and Dikmen, Z. G. (2023). Cancer stem cells and anti-tumor immunity. Curr. Stem Cell Res. Ther. 18, 445–459. doi:10.2174/1574888X18666221017142032
Yong, L., Tang, S., Yu, H., Zhang, H., Zhang, Y., Wan, Y., et al. (2022). The role of hypoxia-inducible factor-1 alpha in multidrug-resistant breast cancer. Front. Oncol. 12, 964934. doi:10.3389/fonc.2022.964934
Zeller, S. L., Spirollari, E., Dicpinigaitis, A. J., Wainwright, J. V., Hanft, S. J., Gandhi, C. D., et al. (2024). Brain metastases are regulated by immuno-inflammatory signaling pathways governed by STAT3, MAPK and tumor suppressor p53 status: possible therapeutic targets. Anticancer Res. 44, 13–22. doi:10.21873/anticanres.16783
Zhai, X., Xia, Z., Du, G., Zhang, X., Xia, T., Ma, D., et al. (2023). LRP1B suppresses HCC progression through the NCSTN/PI3K/AKT signaling axis and affects doxorubicin resistance. Genes Dis. 10, 2082–2096. doi:10.1016/j.gendis.2022.10.021
Zhai, X., Zhang, H., Xia, Z., Liu, M., Du, G., Jiang, Z., et al. (2024). Oxytocin alleviates liver fibrosis via hepatic macrophages. JHEP Rep. 6, 101032. doi:10.1016/j.jhepr.2024.101032
Zhang, C., Qin, C., Dewanjee, S., Bhattacharya, H., Chakraborty, P., Jha, N. K., et al. (2024a). Tumor-derived small extracellular vesicles in cancer invasion and metastasis: molecular mechanisms, and clinical significance. Mol. Cancer 23, 18. doi:10.1186/s12943-024-01932-0
Zhang, C., Sun, D., Li, C., Liu, Y., Zhou, Y., and Zhang, J. (2022). Development of cancer-associated fibroblasts subtype and prognostic model in gastric cancer and the landscape of tumor microenvironment. Int. J. Biochem. Cell Biol. 152, 106309. doi:10.1016/j.biocel.2022.106309
Zhang, H., Xia, T., Xia, Z., Zhou, H., Li, Z., Wang, W., et al. (2024b). KIF18A inactivates hepatic stellate cells and alleviates liver fibrosis through the TTC3/Akt/mTOR pathway. Cell Mol. Life Sci. 81, 96. doi:10.1007/s00018-024-05114-5
Zhang, H., Yang, T., Wu, H., Yi, W., Dai, C., Chen, X., et al. (2023c). MPP8 governs the activity of the LIF/STAT3 pathway and plays a crucial role in the differentiation of mouse embryonic stem cells. Cells 12, 2023. doi:10.3390/cells12162023
Zhang, J., Peng, G., Chi, H., Yang, J., Xie, X., Song, G., et al. (2023b). CD8 + T-cell marker genes reveal different immune subtypes of oral lichen planus by integrating single-cell RNA-seq and bulk RNA-sequencing. BMC Oral Health 23, 464. doi:10.1186/s12903-023-03138-0
Zhang, X., Zhang, P., Cong, A., Feng, Y., Chi, H., Xia, Z., et al. (2023a). Unraveling molecular networks in thymic epithelial tumors: deciphering the unique signatures. Front. Immunol. 14, 1264325. doi:10.3389/fimmu.2023.1264325
Zhang, Z., and Richmond, A. (2021). The role of PI3K inhibition in the treatment of breast cancer, alone or combined with immune checkpoint inhibitors. Front. Mol. Biosci. 8, 648663. doi:10.3389/fmolb.2021.648663
Zhao, Y., Wei, K., Chi, H., Xia, Z., and Li, X. (2022). IL-7: a promising adjuvant ensuring effective T cell responses and memory in combination with cancer vaccines? Front. Immunol. 13, 1022808. doi:10.3389/fimmu.2022.1022808
Zheng, J., Mo, J., Zhu, T., Zhuo, W., Yi, Y., Hu, S., et al. (2020). Comprehensive elaboration of the cGAS-STING signaling axis in cancer development and immunotherapy. Mol. Cancer 19, 133. doi:10.1186/s12943-020-01250-1
Zhou, J. G., Liang, R., Wang, H. T., Jin, S. H., Hu, W., Frey, B., et al. (2023b). Identification and characterization of circular RNAs as novel putative biomarkers to predict anti-PD-1 monotherapy response in metastatic melanoma patients - knowledge from two independent international studies. Neoplasia 37, 100877. doi:10.1016/j.neo.2023.100877
Keywords: breast cancer, brain metastasis, molecular mechanisms, signaling pathways, immune surveillance
Citation: Liang Z, Mo Y, Zhang Y, Yu Y and Ji Y (2025) Molecular mechanisms and signaling pathways related to brain metastasis in breast cancer. Front. Pharmacol. 16:1585668. doi: 10.3389/fphar.2025.1585668
Received: 01 March 2025; Accepted: 21 March 2025;
Published: 27 March 2025.
Edited by:
Minghua Ren, First Affiliated Hospital of Harbin Medical University, ChinaReviewed by:
Rui Liang, Chongqing University, ChinaCopyright © 2025 Liang, Mo, Zhang, Yu and Ji. This is an open-access article distributed under the terms of the Creative Commons Attribution License (CC BY). The use, distribution or reproduction in other forums is permitted, provided the original author(s) and the copyright owner(s) are credited and that the original publication in this journal is cited, in accordance with accepted academic practice. No use, distribution or reproduction is permitted which does not comply with these terms.
*Correspondence: Yinan Ji, anluNzE2QGhvdG1haWwuY29t
†These authors have contributed equally to this work
Disclaimer: All claims expressed in this article are solely those of the authors and do not necessarily represent those of their affiliated organizations, or those of the publisher, the editors and the reviewers. Any product that may be evaluated in this article or claim that may be made by its manufacturer is not guaranteed or endorsed by the publisher.
Research integrity at Frontiers
Learn more about the work of our research integrity team to safeguard the quality of each article we publish.