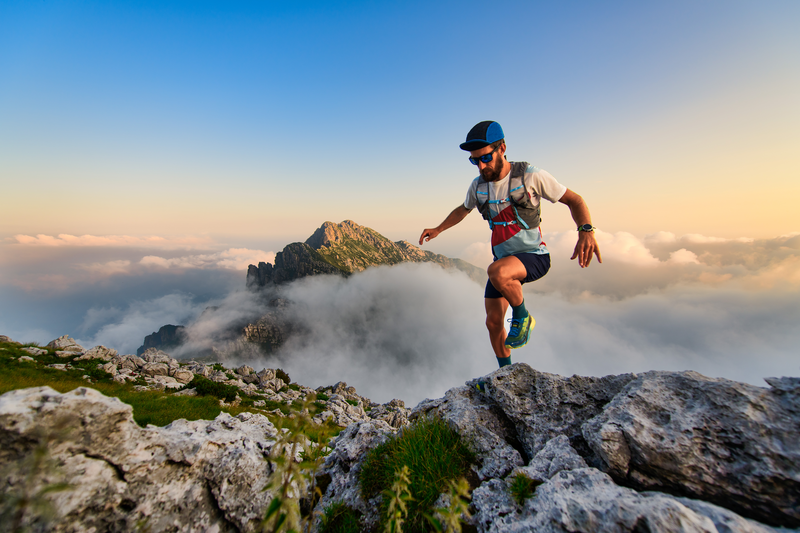
94% of researchers rate our articles as excellent or good
Learn more about the work of our research integrity team to safeguard the quality of each article we publish.
Find out more
ORIGINAL RESEARCH article
Front. Pharmacol.
Sec. Experimental Pharmacology and Drug Discovery
Volume 16 - 2025 | doi: 10.3389/fphar.2025.1584463
This article is part of the Research Topic Anti-cancer Nanomedicines: From Design to Clinical Applications View all 8 articles
The final, formatted version of the article will be published soon.
You have multiple emails registered with Frontiers:
Please enter your email address:
If you already have an account, please login
You don't have a Frontiers account ? You can register here
The treatment of ovarian cancer is hindered by its insidious onset and rapid progression. Exosomes (EXOs) present a promising therapeutic strategy for ovarian cancer by modulating the tumor microenvironment. However, concerns regarding the biosafety of animal-derived EXOs pose significant challenges to the development of innovative formulations. In this study, we propose a universal strategy to engineer plant-derived EXOs as microenvironment nanoregulators for targeted ovarian cancer therapy. EXOs derived from ginger were purified, loaded with the natural bioactive compound curcumin (Cur) with high encapsulation efficiency, and functionalized with a tumortargeting aptamer. Upon intravenous administration, the resulting multifaceted microenvironment nanoregulator, termed A GE@Cur, effectively accumulates at the tumor site and exerts a tumorsuppressive effect through remodeling the tumor microenvironment. This novel therapeutic platform not only addresses the limitations of animal-derived EXOs but also paves the way for the development of innovative microenvironment regulators in clinical applications.
Keywords: Plant-derived exosomes, Tumor Microenvironment, Curcumin, targeted therapy, Drug delivery
Received: 27 Feb 2025; Accepted: 12 Mar 2025.
Copyright: © 2025 Zu, Li, Li, Ma, Yang, Zhang, Ma, Wu and Ha. This is an open-access article distributed under the terms of the Creative Commons Attribution License (CC BY). The use, distribution or reproduction in other forums is permitted, provided the original author(s) or licensor are credited and that the original publication in this journal is cited, in accordance with accepted academic practice. No use, distribution or reproduction is permitted which does not comply with these terms.
* Correspondence:
Chunfang Ha, General Hospital of Ningxia Medical University, Yinchuan, Ningxia Hui Region, China
Disclaimer: All claims expressed in this article are solely those of the authors and do not necessarily represent those of their affiliated organizations, or those of the publisher, the editors and the reviewers. Any product that may be evaluated in this article or claim that may be made by its manufacturer is not guaranteed or endorsed by the publisher.
Research integrity at Frontiers
Learn more about the work of our research integrity team to safeguard the quality of each article we publish.