- College of Pharmacy, Heilongjiang University of Chinese Medicine, Harbin, China
Aster tataricus L.f. (A.tataricus), a perennial herb of the genus Aster in the Asteraceae (Compositae) family. It is associated with a spicy, bitter, and warm nature and belongs to the Lung Meridian. The medicinal parts of A.tataricus are flowers, leaves, roots, and rhizomes. A.tataricus was first recorded in Shuo Wen under the alias “Zi Wan” (茈菀). Traditionally, it is utilised to clear lung qi, promote fluid flow, calm adverse-rising energy, relieve cough, resolve sputum, and regulate secretions. However, it is worth noting that A.tataricus has certain hepatotoxicity. Modern pharmacology indicates that A.tataricus can be used to treat various diseases, including those of the respiratory and urinary systems. In this review, all available information on A.tataricus was collected via academic databases such as PubMed, SciFinder Scholar, CNKI, iPlant, Google Scholar, Web of Science, GBIF, and Masterpieces of Traditional Chinese Medicine. To date, more than 200 metabolites have been isolated and characterized from A.tataricus, including terpenoids, flavonoids, polypeptides, and others. These compounds demonstrate a wide range of pharmacological activities, such as anti-inflammatory effects, antitussive and bronchodilatory properties, anticancer activity, antioxidant effects, treatment of osteolytic disorders, management of urinary system diseases, alleviation of acute lung injury, and enhancement of memory. Meanwhile, the different polarity extracts of A.tataricus also exhibit some toxicological characteristics, the astin has a similar structure to that of cyclochloridine, the hepatotoxic metabolite of penicillin; its saponins also have hemolytic effects. However, there are currently few studies on the toxicology of A.tataricus. Further in-depth research is needed to explore the potential mechanisms underlying the toxicity of A.tataricus. The toxicity of A.tataricus can be reduced through compatibility and processing, but this aspect has received little discussion and further research on quality standardization is needed. To ensure the sustainable development of A.tataricus, we have also summarized its artificial cultivation techniques. Shionone and astin are the characteristic components of A.tataricus. Their pharmacological effects have been deeply studied, but the research on other metabolites is relatively scarce. Therefore, this article focuses on botany, artificial cultivation, phytochemistry, ethnopharmacology, pharmacology, toxicology, and comprehensive utilization of A.tataricus. Discuss the future research prospects and existing problems of A.tataricus, and provide references for further research on A.tataricus and the establishment of quality control standards.
1 Introduction
According to The Compendium of Materia Medica, Aster tataricus L.f. (A.tataricus) is termed “Ziwan” (紫菀) due to its soft, purple roots (K.D, 2023). It is a perennial herb of the genus Aster in the Asteraceae (Compositae) (Yang, 2003). It is also known as China regional common names such as Huan Hun Cao(还魂草), Qing Wan(青菀), and Shan Ma Lan (山马兰) (Li et al., 2010). In temperate regions of America, Europe, and northern Asia, A.tataricus is an important cash crop with high ornamental value. Its ability to attract bees and butterflies makes it a popular flower in Europe and America (K.D, 2023). In some Asian countries, young seedlings, tender stems, and leaves harvested from May to June are consumed as vegetables (Ahn et al., 2018). Additionally, A.tataricus is used in the production of essential oils (Choi, 2012), pesticides (Rhee et al., 1981), antioxidants in edible oils (Chen et al., 2012), and other products. The plant is distributed across China, Korea, Japan, the northern United States, and eastern Siberia in Russia (Figure 1). It thrives in shady, humid areas at altitudes of 400–2,000 m (Wan et al., 2016). The earliest application of A.tataricus can be traced back to the Shennong Materia Medica. It is regarded as an important medicine for lung disease, as it moistens the lungs and relieves cough and phlegm. In recent decades have shown that in addition to eliminating phlegm and relieving cough, Extensive biological testing has demonstrated that A.tataricus exhibits a wide range of pharmacological properties, such as anti-inflammation (Wu et al., 2023), treating acute lung injury (Song et al., 2022), anti-tumor (Schafhauser et al., 2019), and alleviating osteoporosis (Lee et al., 2023b; Su et al., 2022). Especially, its effect in relieving cough and phlegm has lasted for more than 2000 years (Dong et al., 2024). Traditional medicine practitioners believed that the expectorant and antitussive role of A.tataricus is ascribed to the tropism of taste. Different from traditional medicine, modern medical practitioners believe that A.tataricus plays a role by reducing inflammatory factors (Deng et al., 2023) and relaxing bronchial smooth muscle (Peng et al., 2016a). Over the past few decades, various nutrients and active compounds have been isolated from A.tataricus, including amino acids, essential trace elements, terpenoids, flavonoids, polypeptides, organic acids, and volatile oils (Han et al., 2023). Among these, terpenoids are the most abundant and extensively studied components (Huang et al., 2008). Shionone, a characteristic terpenoid, is present throughout the plant and serves as a quality marker of A.tataricus according to the Pharmacopoeia of the People’s Republic of China (ChP) (Wu et al., 2003; Yang et al., 2024). Studies have shown that polypeptides have obvious anticancer function, but it has a similar structure to that of cyclochloridine, the hepatotoxic metabolite of penicillin (Peng et al., 2016b).
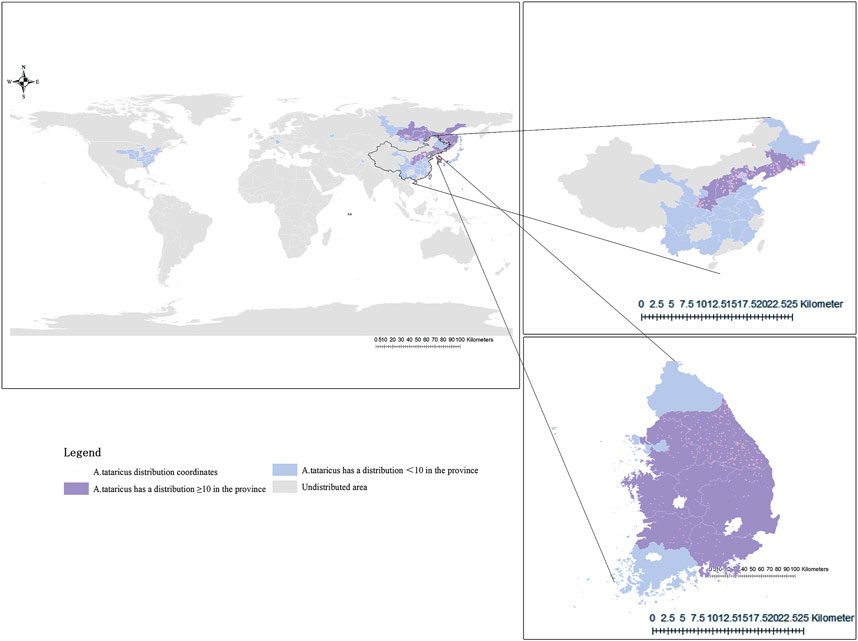
Figure 1. Distribution map of A.tataricus. Orange dots represent the distribution position of A.tataricus. Purple-shaded areas represent regions where A.tataricus is distributed across more than 10 coordinates. Blue-shaded areas represent regions where A.tataricus is distributed across less than 10 coordinates, and grey-shaded areas represent regions where A.tataricus is not distributed.
Owing to the remarkable therapeutic efficacy of A.tataricus, its market demand has steadily increased over recent years. Consequently, expanding production capacity has become a critical priority. This review provides an in-depth examination of the botany, phytochemistry, ethnopharmacology, pharmacological activities, and toxicology of A.tataricus. Additionally, it offers the first comprehensive summary of cultivation techniques and integrated utilization strategies for this species. Furthermore, this study elucidates the “double-edged sword” nature of cyclic peptides—highlighting both their therapeutic benefits and potential toxicity—which serves as a foundational reference for ensuring safe usage and guiding structural modifications in future research, thereby enhancing the developmental potential of A.tataricus.
2 Materials and methods
2.1 Identification and selection of studies
The initial phase of our analysis involved systematically assessing all studies identified through keyword searches pertaining to A.tataricus. Following the removal of duplicate entries, we conducted a preliminary review of titles and abstracts to evaluate their relevance based on the established inclusion criteria. For studies that satisfied these criteria, a detailed examination was performed, encompassing a thorough analysis of the full text and an in-depth review of the reference lists to ensure a comprehensive understanding of the relevant literature.
2.2 Search strategy
We identified the studies independently using the following keywords: Aster tataricus, A.tataricus extract, Fan hun cao, Zi wan. In addition, reported pharmacological activities and phytochemical compositions were searched as keywords. This study only includes results found before March 2025 (without time restrictions before this date). The search was carried out in the electronic bibliographic databases, including PubMed (https://pubmed.ncbi), CNKI (http://www.cnki.net), Baidu Scholar (https://xueshu.baidu.com/), Google Scholar (http://scholar-xm.top) and Traditional Chinese Medicine Baodian Network (www.ZYBD.com).
2.3 Inclusion and non-inclusion criteria
Our inclusion criteria encompassed all experimental studies investigating various aspects of A.tataricus, including its botany, phytochemistry, ethnopharmacology, pharmacology, toxicology, and comprehensive utilization, irrespective of the extraction methods employed. Additionally, we incorporated Chinese dissertations and theses that detailed the properties of A.tataricus. Studies performed in humans, editorials, conference abstracts, the contents of dissertations that have been published in scientific journals, and conference proceedings were also excluded.
2.4 Search results
The preliminary search yielded a total of 4016 records, distributed across various databases as follows: 83 from PubMed, 1921 from CNKI, 990 from Baidu Scholar, 1021 from Google Scholar, and one from the Traditional Chinese Medicine Baodian Network. After removing 2001 duplicate entries, we conducted a thorough review of titles and abstracts, which led to the exclusion of an additional 475 reports. This left 2015 studies for further evaluation. Upon detailed examination of the full texts, 767 studies were excluded: 432 for involving A.tataricus in combination with other substances, 333 for being published prior to 2003, and 2 due to unavailability of the full text. Through this rigorous screening process, a final set of 152 articles was selected for inclusion (Figure 2).
2.5 Others
According to the method described in the literature, VOS viewer software was used to visualize the similarity of all keywords in the literature reported before September 2024 (Figure 3). The figure shows that many studies have been conducted on the extraction of its chemical components, and most of the research on A.tataricus is concentrated on animal experiments.
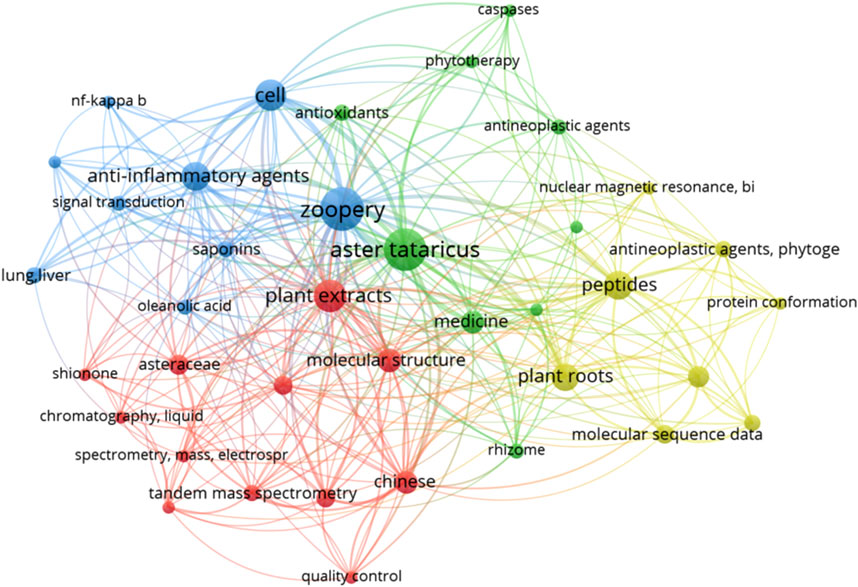
Figure 3. VOS viewer was used to visualise the keyword similarity across publications related to A.tataricus. The figure represents linear relationships between keywords, the larger the dot, the more frequently the word appears in the article. Many studies have explored the extraction of its chemical components, and most of A.tataricus research has been conducted using animal models.
At the same time, GBIF (www.gbif.org) and ArcGIS software were used to depict the distribution map of A.tataricus, biorender (https://www.biorender.com/) was used to draw the pharmacological mechanism map of A.tataricus, the flowchart templet from the PRISMA statement (https://www.prisma-statement.org/), and we utilised the iPlant (http://www.iplant.cn/) as a key resource to describe the botany and Photoshop was used to beautify the pictures.
3 Botany
During the Northern and Southern Dynasties of China, Hongjing Tao described the morphological characteristics of A.tataricus in Ji Zhu of the Materia Medica, his description—highlighting basal leaves, thick stem hairs, purple-colored tongue-shaped flowers, and soft roots—aligns with the current understanding of A.tataricus (Yu et al., 2023). After 1500 years of history, the shape of A.tataricus is now described as follows: The rhizome rises obliquely, the stem is upright and strong, reaching a height of 40–50 cm. With fibrous dead leaves at the base and adventitious roots, ribs, and grooves, covered with sparse, coarse hair and sparse leaves. The basal leaves wither during anthesis. These leaves are oblong or elliptic-spoon-shaped, the lower part tapers to grow into a stalk, and measure 20–50 cm in length and 3–13 cm in width. The leaf margin is either ornately lobed or shallowly serrated with small acute teeth. The leaves on the lower part of the stem are spatulate-oblong in shape, with their bases gradually tapering or abruptly narrowing into a broad-winged petiole; the outer margin is densely cleft except at the tip. The middle leaves are oblong to oblong-lanceolate, sessile, with entire margins or shallowly toothed. The upper lobe is narrow. The leaves are thickly papery in texture, with the upper surface being rough and the lower surface sparsely pubescent, featuring dense hairs along the veins. There are 6–10 pairs of lateral veins. The capitulum measures 2.5–4.5 cm in diameter and is mostly arranged in a compound corymbose cyme at the apex of the stem, with slender pedicels and linear bracteoles. The involucre is hemispherical, measuring 1–2.5 cm in diameter, with three layers of imbricate phyllaries that are linear to linear-lanceolate in shape, with apical or obtuse tips, densely puberulent, and with broadly membranous and reddish-purple margins. There are approximately 20 ligulate flowers, with the ligules being blue-purple. The achenes are obovate-oblong in shape, purplish-brown in color, and sparsely hirsute on the upper part. The corolla is simple, stained white or reddish, and composed primarily of strigose setae (Li H. et al., 2024) (Figure 4).
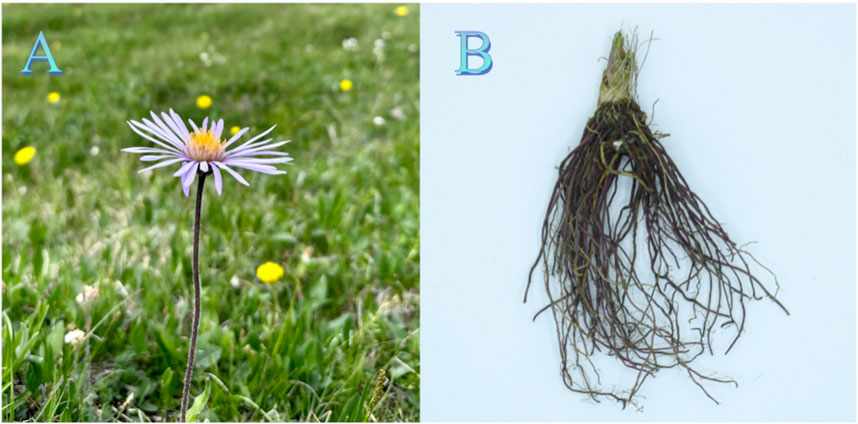
Figure 4. The morphology and medicinal parts of A.tataricus. (A) The aerial part of A.tataricus (photographed in the National Botanical Garden of China, Beijing). (B) The root and rhizome of A.tataricus (dried medicinal material).
4 Artificial cultivation
Environmental factors such as soil composition, climate, and altitude have a significant impact on the efficacy of traditional Chinese medicine (TCM). Authentic medicinal herbs, which are synonymous with high-quality Chinese medicinal herbs, are selected through long-term clinical application of TCM, produced in a specific region, and have a high reputation. It has the characteristics of more stable quality and better efficacy (Huang et al., 2020). In China, A.tataricus is mainly produced in Hebei and Anhui provinces. It grows easily in low mountain shady slope wetlands, mountain tops, low mountain grassland, and marshland at an altitude of 400–2,000 m. Therefore, A.tataricus should be selected in loam soil with loose and high humus content or sandy loam. Through the review of previous studies, it was found that the bisexual and monosexual flowers of A.tataricus coexisted, self-pollination was the main method, and the seed setting rate was extremely low, which could not meet the seed demand of large-scale planting of A.tataricus. Therefore, most of the cultivation of A.tataricus uses roots and rhizomes as seedlings, so it is extremely important to establish a standardized system of high-quality seedlings (Yao et al., 2019). There are three main factors affecting the quality of seedlings: shoot hair number, shoot diameter, and shoot distance (Tian et al., 2012). The study found that (Song N. N. et al., 2024), the seedlings with strong, purplish red rhizomes, dense and short nodes, many dormant buds, white and tender broken bones, and no diseases and pests were selected. The young part at the lower end and the upper end of the reed head were removed, and the middle part was cut into small segments of 5–10 cm, with 2–3 bud eyes in each segment. Because A.tataricus leaves grow fastest from July to September, it is recommended to carry out spring planting (after the land is thawed, before the spring equinox term) and water 1–2 times before emergence. It likes to be moist, so it needs to be watered frequently to keep the soil surface moist, but it cannot be watered too much, which makes it easy to rot the roots. In addition to this, three weeding and fertilization procedures were performed during A.tataricus growth. The first time was after the whole seedling, the second time was about 7–10 cm in the seedling height, and the last time was before the plant was closed (Hu, 2014). Around the time of frost’s fall, that is, around the end of October, is the best time to harvest aster, and strong roots should be selected as seedlings at this time.
5 Nutrients and phytochemistry
Roots of A.tataricus (RA) contain 20 amino acids necessary for protein synthesis, including seven essential amino acids for humans: lysine, methionine, threonine, valine, leucine, isoleucine, phenylalanine, and histidine—the last one is particularly important for infants and young children. Among the free amino acids in RA, arginine and histidine have the highest mass fraction (Xi et al., 2003). Dietary arginine, in particular, supports wound healing, regulates endocrine function, and enhances immune activity (Morris, 2016) (Figure 5).
RA is also rich in trace elements, with high levels of Ca, Mg, and Fe, followed by Zn, Mn, Cu, and Mo, while Se is present in the lowest concentrations (Xi et al., 2003). A multitude of research indicates that the variety and density of inorganic elements in traditional Chinese medicinal practices play a pivotal role in the medicinal characteristics of their constituents (Ding, 2021; Hu, 2017; Li H. Y. et al., 2022; Chen W. et al., 2022). Using ICP-MS combined with stoichiometry, researchers compared inorganic elements of different medicinal parts of A.tataricus. Roots were found to have higher concentrations of U, V, Cr, Ga, and Ag, whereas rhizomes contained more Cu, Cd, Tl, and Zn (Li L. et al., 2023).
In 2018, Sun et al. (2018) identified and initially characterized 131 compounds in A.tataricus, including various bioactive compounds, such as terpenoids, polypeptides, flavonoids, phenols, organic acids, and volatile oils. Zhao et al. (2015) further isolated and determined phenolic acids, pentapeptides, and triterpene saponins in RA. Over the past 2 years, a new biflavonoid compound (Chen T. et al., 2022), 5 α-pyranone derivatives (Li X. et al., 2023), and five terpenoids (Li J. et al., 2024) have been discovered in A.tataricus. To date, approximately 200 chemical components have been successfully isolated and confirmed from A.tataricus, among which terpenoids are the most abundant, accounting for approximately 40% of the total components (Fan et al., 2024).
5.1 Terpenoids
Terpenoids are the primary active substances in A.tataricus. To date, 66 terpenoids have been identified in A.tataricus, and their chemical structures are listed in Table 1 and Figure 6. Studies have shown that the predominant component in A.tataricus is shionone-type triterpene compounds. As the first isolated and structurally determined compound in A.tataricus, shionone is a unique component of this plant, characterised by a complete six-membered four-ring skeleton and a unique structure of 3-oxo-4-monomethyl. Ninety percent of shionone is synthesised in a complex pathway mediated by a single enzyme, shionone synthase (Sawai et al., 2011). As a quality marker, ChP stipulates that authentic A.tataricus must contain no less than 0.10% shionone (Jaiswal and Lee, 2023). Studies have shown that (Zhang et al., 2022) the content of shionone in fresh medicinal materials is approximately three times that of traditional Chinese medicine pieces, and the content varies greatly in different medicinal materials. The content in roots is about 100 times that of stems and flowers. In roots, the content of shionone also varies. The content of shionone in roots is higher than that in main roots and rhizome. At the same time, some studies have shown that processing methods (Yang et al., 2021), producing area (Wu et al., 2003), and harvest season also affect the content of shionone.
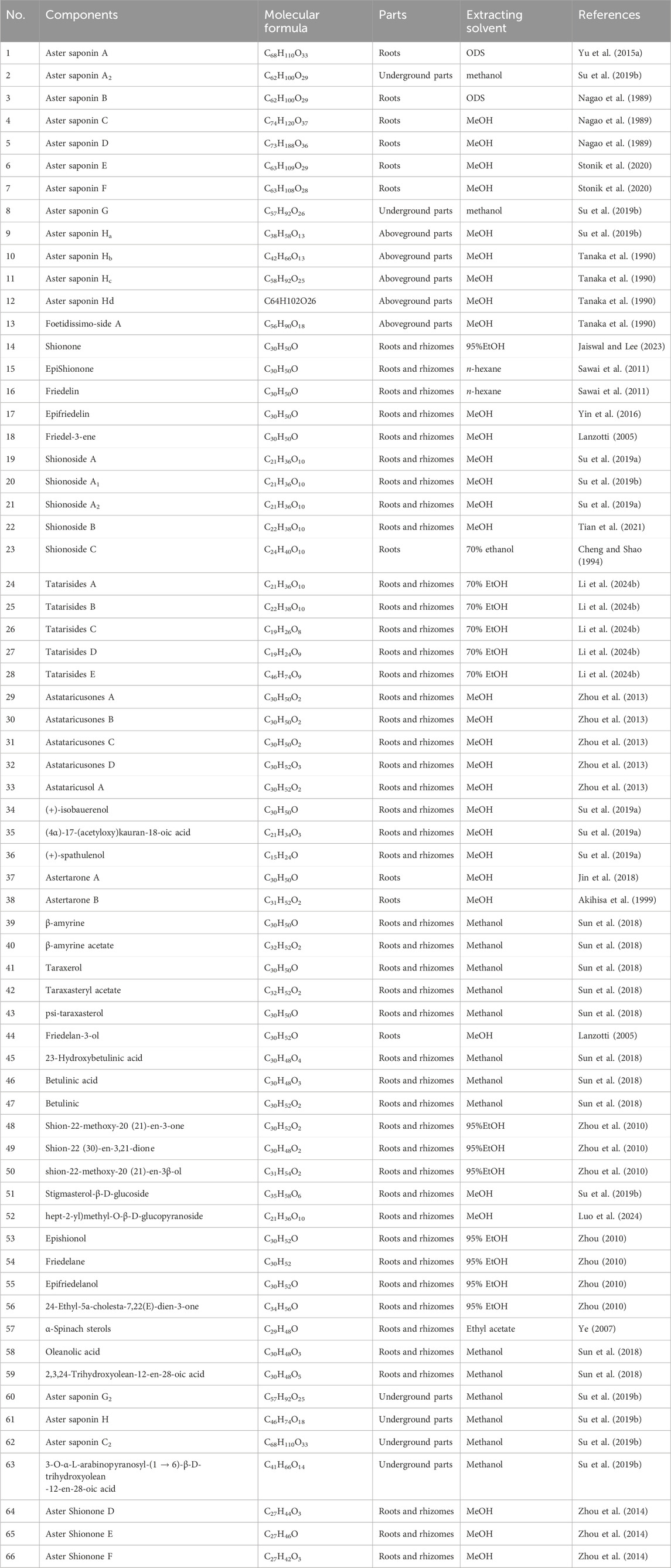
Table 1. Names, molecular formulas, harvesting parts, extraction reagents and literature sources of terpenoids in A.tataricus.
5.2 Flavonoids
Flavonoids, widely found in plants, regulate their physiological functions. They are the largest class of secondary metabolites in plants and exhibit potent pharmacological activities (Liu et al., 2021). Jia et al. (2023) identified more than 80 flavonoid metabolites in A.tataricus through multiple omics methods. The metabolites included 31 flavonols, 29 flavonoids, six isoflavones, five dihydroflavonoids, five anthocyanins, two flavonoid carbon glycosides, and one each of dihydroflavonol and flavanol. In recent years, from the MeOH extract of A.tataricus, a novel biflavonoid compound of the C-3′-C-6″ variety, termed (2R,2″R)-7-O-methyl-2,3,2″,3″-tetrahydrorobustaflavone, was extracted (Chen T. et al., 2022) (Figure 7). At present, the determination of flavonoid content in A.tataricus mainly focuses on quercetin and kaempferol, which mainly accumulate in the cortex and pith (Guo et al., 2016). After measuring the quercetin content in different parts of A.tataricus in different areas, it was found that the quercetin content in the roots and rhizomes is considerably higher than that in the flowers (Song et al., 2009).
5.3 Polypeptides
Peptides also stand out as distinct elements in A.tataricus (Figure 8). Currently, 28 peptides have been isolated from A.tataricus, including halogenated cyclic polypeptides (astins A-P, and tataricins A-B) and linear polypeptides (asterinins A-F, and aurantiamide acetate) (Zhao et al., 2015). Studies found that (Rossi et al., 2004) astins are only found in the root of A.tataricus, and their conformational flexibility is closely linked to the biological activity of their family members. In particular, cyclic astin family members, in the form of cyclic peptides, inhibit the growth of anti-tumor cell lines, whereas non-cyclic astins structures do not exhibit similar anti-tumor activity. Cyclic pentapeptides in the astin family are distinguished by having four amino acids that are not proteins, of which dichloroproline and isothreonine are the most prominent characteristic residues (Cozzolino et al., 2005). In 1998, Lu et al. (Lu et al., 1998) discovered and isolated a dipeptide compound aurantiamide acetate from the petroleum ether extracts of the roots and rhizomes of A.tataricus. This component exhibited inhibitory activity against superoxide radical formation. In 2013, Xu et al. (2013) identified eight new polypeptide compounds from A.tataricus, including two new cyclic tetrapeptides, tataricins A and tataricins B. These two compounds have unique cyclic peptide skeletons and △2,4Pro side chains. Additionally, six novel chlorinated cyclopentanone peptides were isolated, including Astin P, a cyclic pentapeptide with the sequence (L-Pro(Cl2)1-L-allo-Thr2-LSer3-L-b-Phe4-L-ava5) (Figure 9). A study by Schafhauser et al. (2019) pointed out that astins originate from the endophytic fungus Cyanodermela astris, which is associated with A.tataricus. These compounds are products of the NRPS biosynthetic pathway. They exist not only in roots and rhizomes but are also abundant in leaves and inflorescences.
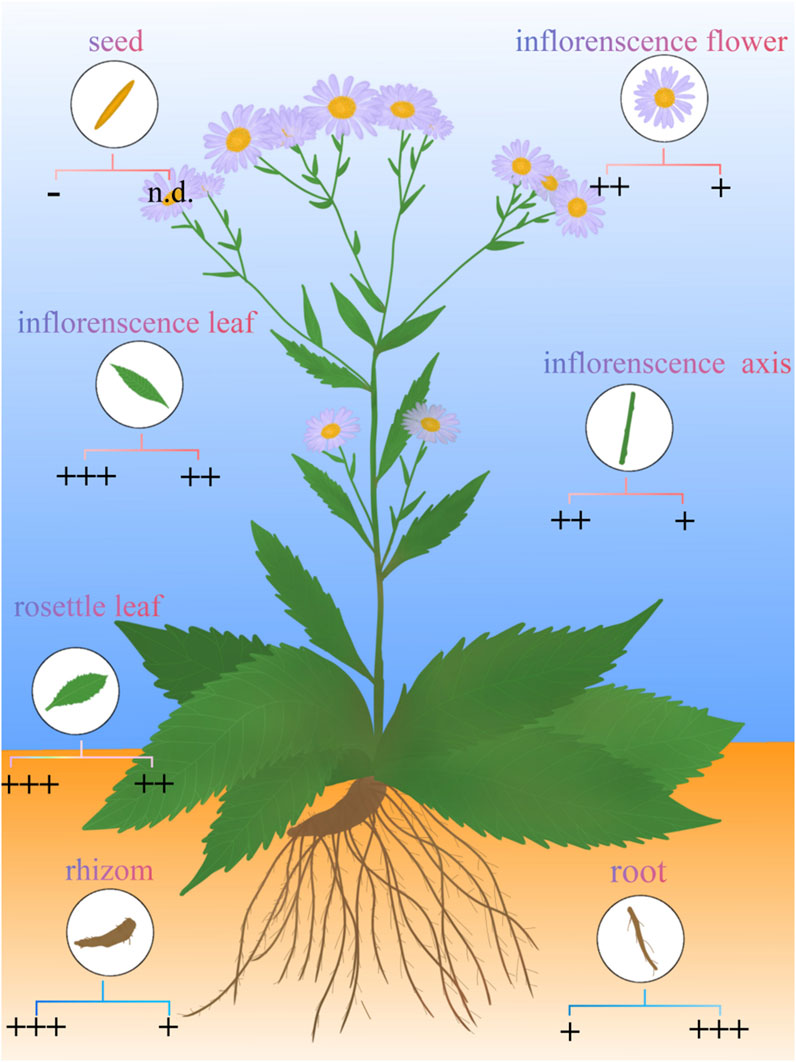
Figure 8. Distribution of shionone and astins in A.tataricus (represented in circular symbols in different tissues). The concentrations of shionone and astins are represented in sign below, the left is the concentration of astins, the right is the concentration of shionone, astins concentration:+: <5 × 1011, ++: 5 × 1011–5 × 1012, +++: >5 × 1012, N.D.: Unknown concentration; shionone concentration: +: <0.15%, ++: 0.15%–0.3%, +++: >0.3%. Data source: (Chen et al., 2020; Gao et al., 2003; Zhang et al., 2022; Zhang et al., 2021; Schafhauser et al., 2019).
5.4 Phenols and organic acids
The organic acids in the medicinal parts of A.tataricus are primarily aromatic acids (Sun et al., 2018). An evaluation of three chlorogenic acid contents in different underground parts of A.tataricus revealed that the rhizome had the highest chlorogenic acid content, followed by the parent root. In contrast, the root had the lowest content (Wang et al., 2020a). Tian et al. (2008) simultaneously determined three chemical components and found that A.tataricus contains a high content of ferulic acid. In addition to chlorogenic acid and ferulic acid, a variety of organic acid components (including polyphenols) are present in A.tataricus, including cryptochlorogenic acid, caffeic acid, isoferulic acid, benzoic acid, para-hydroxybenzoic acid, oleanolic acid, palmitic acid, succinic acid, caffeic acid methyl ester, 3,4-dicaffeoylquinic acid, 4-hydroxybenzoic acid, dicetyl ester, cetyl p-hydroxycinnamate, docosanoic acid, behenic acid, 2, 2-dimethylsuccinic acid (Jin et al., 2008) and the phenolic compounds 3-O-feruloylquinate methyl ester and (+)-isolaricidin- 9-β-D-glucopyranoside (Gao et al., 1994) (Figure 10).
5.5 Volatile oil
Yang et al. (2008) identified seven volatile oil components and isolated 1-acetoxy-2-ene(E)-4,6-decandiyne. Zhang J. L. et al. (2012) analyzed and found that the content of 1-acetoxy-2-ene(E)-4,6-decandiyne is the most abundant volatile oil i in A.tataricus, followed by 32 volatile oil components, such as 5-(1,3-dimethylbutylidene)-1,3- cyclopentadiene, γ-elemene, m-diisopropyl, α-springene, τ-cadinol, and β-pinene (Figure 11).
5.6 Other compounds
In addition to the compounds mentioned above, A.tataricus also contains polysaccharides, coumarins, sterols, and anthraquinone derivatives (Fan et al., 2024). Two alkanes, n-octadecane and n-tricanol, were also separated from the methanol extract (Ye, 2007). Acetylene and other substances have been isolated from the ethanol extract of A.tataricus (ATEE) (Zhou, 2010). Among them, coumarin compounds include coumarins, scopoletin, and umbellitone (Cai et al., 2023). Li X. et al. (2023) identified five unidentified α-pyranone derivatives, namely neuropyrones A–E, from the endophytic fungus Neurosporadictyophora WZ-497. Du et al. (2014) successfully purified a homogeneous polysaccharide (ATP-II) consisting of glucose, galactose, mannose, rhamnose, and arabinose (2.1: 5.2: 2.1: 1.0: 1.2) from A.tataricus. Zhang Y. et al. (2012) isolated A water-soluble polysaccharide (WATP) composed of galactose, glucose, fucose, rhamnose, arabinose, and mannose (2.1: 1.3: 0.9: 0.5: 0.3: 0.6) from A.tataricus. Wang et al. (2003) isolated anthraquinones in A.tataricus from the roots and rhizome extracted by ethyl acetate, including emodin, chrysophanol, aloe-emodin, and physcion methyl ether (Figure 12), whereas Ng et al. (2003) isolated 1,7-dihydroxy-6-methyl-anthraquinone, and the bisanthraquinone skyrin from A.tataricus. Skyrin was identified as the main colorant of its endophytic fungus Cyanodermela astris (Jahn et al., 2017). In addition, amide compounds such as [N-(N-benzoyl-L-phenylalanoyl)-O-acetyl-L-phenylalanine] (Zou et al., 1999), and furan compounds, including 11-hydroxy-10,11-dihydro-euparin (Jin et al., 2008) are present in A.tataricus. At present, there are few studies on steroids in A.tataricus and only three sterols have been found, isolated, and identified, namely stigmasterol, β-sitosterol, and daucosterol. In addition, Tang et al. (2006) extracted 0.1506% lipid-soluble total alkaloids from A.tataricus.
6 Ethnopharmacology
6.1 Traditional application
A.tataricus is distributed across China, Korea, Japan, the northern United States, and eastern Siberia in Russia. Over millennia, A.tataricus’s roots, stems, leaves, and whole plants have served diverse medicinal roles in traditional folk medicine across southeast Asia. In China, A.tataricus is known as “Zi Wan” or “Huan Hun Cao”. A.tataricus was first recorded in Shuo Wen under the alias “Zi Wan” (茈菀). Table 2 records the herbal research of A.tataricus. Shennong Materia Medica used the name “Zi Wan” (紫菀), which has been carried over subsequent generations. A.tataricus is an important drug for clearing and moistening the lungs. Its pharmacological effect was first recorded in Shennong’s Herbal Classic (Li, 1800). The whole herb is mainly used for treating coughs caused by the upward flow of qi, cold-heat condensation in the chest, poison removal, paralysis, and soothing the five internal organs. The parts used are mainly dry roots and stems. The Supplementary Records of Famous Physicians (Tao, 2013) state that “A.tataricus treats symptoms such as coughing up pus and blood, asthma, palpitations, fatigue, or lesions in the five internal organs. It is also used to treat weakness, address deficiencies, and childhood epilepsy. The Xinxiu Materia Medica of the Tang Dynasty integrates the descriptions of the sexual and taste effects of A.tataricus in the Shennong Materia Medica Classic and Supplementary Records of Famous Physicians. A.tataricus is primarily used to treat lung-related diseases. During the Tang and Song Dynasties, Rihuazi Materia Medica (Ri and Shang, 1983) compared with its predecessors, it has introduced additional therapeutic uses, including quenching thirst, moisturising skin, and replenishing bone marrow. In terms of clinical applications, Qianjin Fang (Sun, 2019) recorded a prescription for treating women with oliguria and hematuria. During the Jin and Yuan Dynasties, Xueyue Jia noted in Medicine Huayi that (Jia, 2015) A.tataricus was mainly used for chronic coughing, expectoration, coughing up blood, lung weakness, and diabetes in the lung system. Additional indications for use in the liver and kidney meridians included insufficient fatigue and heat in the liver meridian, heat accumulation and stagnation of qi, vomiting blood and bleeding in the stool, dry stools, and short and red urine in the kidney system. Modern texts, such as the Chinese Materia Medica (Administration C. F. A. D, 1999) described the effects of A.tataricus as “moistening the lungs and lowering the qi, alleviating phlegm and easing cough.” It is primarily used for lung deficiency, chronic cough, lung weakness, and coughing up pus or blood, aligning with its historical applications. The 2020 edition of the ChP reiterates these uses. In conclusion, A.tataricus helps relieve lung qi, pass body fluid, calm adverse-rising energy, relieve cough, resolve phlegm, and smooth secretions (Yu et al., 2023).
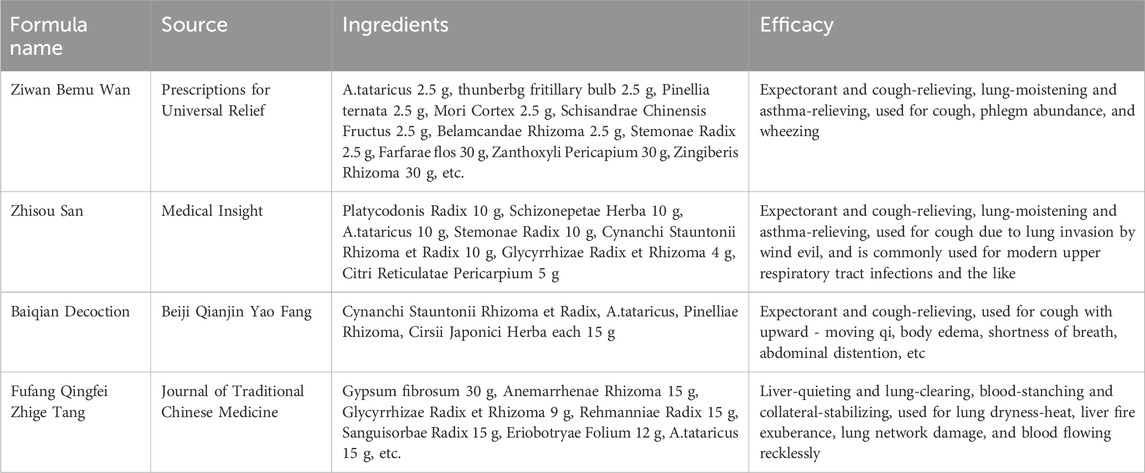
Table 2. A.tataricus related formulas and their chemical components and biological activity effects.
In Japanese Kampo medicine, A.tataricus is commonly used in compound preparations, mainly for treating respiratory diseases, such as for expectorant and cough-stopping purposes, for moistening the lungs, and for exerting antibacterial and anti-inflammatory effects (Commission J. P, 2023). In traditional Korean medicine, it is also often used to treat coughs and asthma (Kang et al., 2021). However, in China, its roots and rhizomes are primarily used for medicinal purposes (Wang et al., 2020b), whereas in South Korea, its flowers are used (Chen et al., 2012). So, many ancient documents from Chinese traditional medicine, Korean medicine, and Japanese traditional medicine have recorded the effects of A.tataricus on relieving cough and phlegm (Su et al., 2019a). Despite varying cultural differences, the historical use of A.tataricus reveals a consistent global trend. Hence, the establishment of a thorough drug quality standard system becomes imperative to effectively support quality control and the advancement of new drug development.
6.2 Processing
The practice of processing holds significant importance in the realm of traditional Chinese medicine, as it can reduce the toxicity of herbs and improve their efficacy. This is true of A.tataricus as well. When detecting the chemical composition of six A.tataricus processed slices, namely raw, honey-fried, stir-fried, vinegar-fried, wine-fried, and steamed, it was found that stir-fried A.tataricus had the highest content of shionone and flavonoids, while raw A.tataricus had the lowest content of shionone (Fan et al., 2018). After honey-fried, the shionone content in A.tataricus significantly increases, while flavonoid levels drop. Its expectorant and cough-relieving effects become more pronounced. In contrast, in the stir-fried product, only shionone shows a marked rise, with flavonoid content remaining largely unchanged (Wang X. et al., 2023; Wu et al., 2006; Xiu et al., 2006). Shizhen Li pointed out in The Compendium of Materia Medica that the spicy nature of A.tataricus can strongly affect the body but may also damage lung yin (Li et al., 2022b). Hence, it is used in conjunction with other medicinal materials to treat wind-cold cough, asthma, fatigue-induced coughing, vomiting, pus formation, bleeding, and other symptoms. For example, in Zhang’s Yi Tong, A.tataricus is combined with drugs such as Ejiao, Fritillaria, Ophiopogon japonicus L. f. (O.japonicus), and Schisandra chinensis Turcz. to address deficiencies, fatigue, lung weakness, and hemoptysis. It is decocted with Glycyrrhiza uralensis Fisch, Asparagus cochinchinensi, Platycodon grandiflorus (P.grandiflorus), honey, Amygdalus Communis Vas, and Mori Cortex to treat coughing and threatened abortion (Yu et al., 2023). A.tataricus is often paired with Tussilago farfara L. (T.farfara), Stemona japonica, and Ephedrae Herba as a standard method for treating pulmonary diseases. When combined with P. grandiflorus, Poria, and O. japonicus, it enhances the effects of dispersing lung qi and promoting water metabolism. Additionally, when used with Coicis Semen, P. grandiflorus, and Amygdalus communis Vas., it further strengthens the actions of dispersing lung qi and relaxing the bowel (Tian et al., 2021). One of the most common prescriptions in China, Zhishou powder (止嗽粉), uses A.tataricus as the primary ingredient (monarch medicine) and is extensively employed in the treatment of both acute and chronic bronchitis, as well as chronic coughs (Dong et al., 2024). Table 3 lists some formulas that affect the chemical composition and biological activity of A.tataricus (Table 2).
7 Pharmacology
7.1 Anti-inflammatory effect
Numerous research reports have pointed out that A.tataricus has well-established anti-inflammatory activity. Inflammation is a natural self-defense mechanism by which the body initiates protection. It represents a multifaceted physiological mechanism that intricately involves the interplay of the immune system, vascular system, and a diverse array of molecular mediators (Prockop and Oh, 2012). A.tataricus mediates inflammatory processes by inhibiting pro-inflammatory mediators, including NO, PGE-2, TNF-α, IL-1β, and IL-6 (Luo et al., 2024). Figure 13 shows a map of the anti-inflammatory mechanisms of A.tataricus.
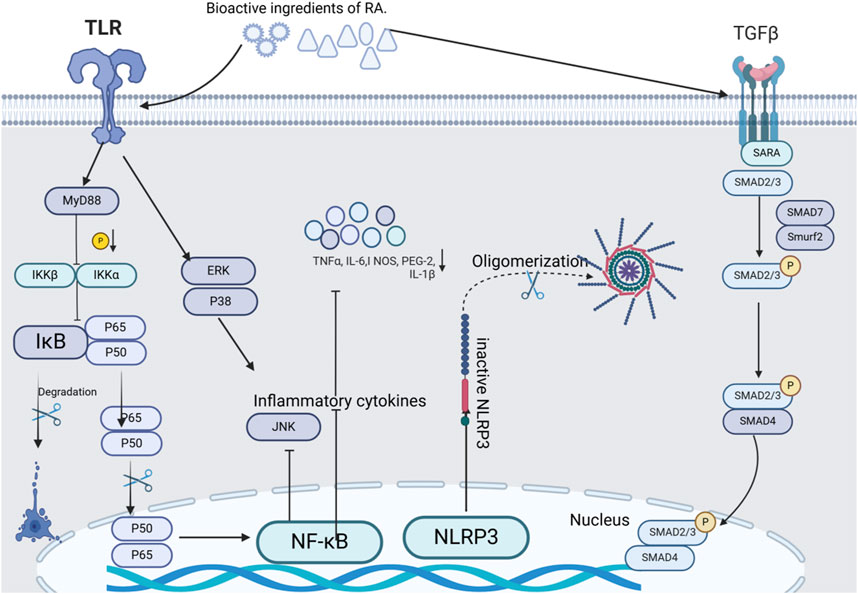
Figure 13. The chief anti-inflammatory, expectorant and antitussive molecular targets and signaling pathways mediated by A.tataricus treatment.
Su et al. (2019b) analyzed the potential inflammatory mechanisms of A.tataricus and found that various compounds in the plant inhibited the release of NO from RAW264.7 cells stimulated by LPS. Among these, aster saponin B was significantly more effective than the other compounds. Through inhibition of NF-κB activation significantly reduced NO production in LPS-induced RAW264.7 cells and blocked phosphorylation of JNK, ERK, and p38 in the MAPK pathway. Collectively, these processes result in a substantial anti-inflammatory effect and effectively inhibit the production of inflammatory cytokines (PGE-2, IL-6, and IL-1β). Li et al. (2018) pointed out that astin C inhibits cGASSTING signaling and the innate inflammatory response triggered by cytoplasmic DNA. As an effective active ingredient, 4-HPA in A.tataricus inhibits hyperosmotic and hypoxia-induced HIF-1α in NR8383 macrophages and reduces inflammatory cytokine levels (Liu et al., 2014). TGF-β1, a widely studied growth factor has many biological functions, including inflammatory response stimulation. Wang et al. (2022) revealed that the aqueous extract of A.tataricus significantly decreased the expression levels of TGF-β1 in the lung tissues of mice infected with Mycoplasma pneumoniae.
Shionone exhibits satisfactory anti-inflammatory activity. It diminishes the secretion of TNF-α and IL-1β by inflammatory macrophages. The inhibitory effect was observed to be the same as that of NF-κB inhibitors, both demonstrating a dose-responsive mechanism that curtails NO release (Wang et al., 2015). In their study of TNBS-induced Crohn’s disease (CD) like colitis in mice, Xu et al. found that (Xu et al., 2024) shionone can reduce intestinal inflammatory mediators (TNF-α, IL-6/IL-1β) expression by inhibiting apoptosis of intestinal epithelial cells. Wang X. et al. (2020), Wang et al. (2021) highlighted that in their investigation of interstitial cystitis (IC), A.tataricus extract (ATE) demonstrated notable anti-inflammatory effects on both rat bladder tissues and urothelial cells (SV-HUC-1) by inhibiting heat shock protein expression and downregulating NLRP3/GSDMD-N signaling pathway. These effects were particularly pronounced when experimented using ATE. Thus, ATE has a remarkable capability to downregulate NLRP3 and other pyropyretic-associated proteins, suggesting its potential use as an NLRP3 inhibitor for the treatment of IC. Furthermore, shionone functions via the NF-κB/NLRP3/GSDMD-N route, reducing the mRNA and protein quantities of NF-κB, NLRP3, ASC, Pro-caspase-1, Caspase-1, GSDMD, and GSDMD-N, thus disrupting the NLRP3 inflammasome pathway. This facilitates IC occurrence reduction.
7.2 Expectorant and antitussive effects
The most common pharmacological effects of A.tataricus are its expectorant and antitussive effects. Lu et al. (1999) found that shionone and epifriedelanol have expectorant and antitussive effects. Yang et al. (2008) found that 1-acetoxy-2-ene (E)-4,6-decyne is the main compound in the volatile oil of A.tataricus and has expectorant effects. Yu et al. (2015b) confirmed that the expectorant effect of A.tataricus is better than that of the anti-cough effect and found that Fr-50, a key component, demonstrates significantly better expectorant effects than shionone. Analysis of Fr-50 revealed that it consisted of 12 chlorogenic acids, 7 saponins, and 13 pentapeptides.
Pharmacopoeia and scholarly documents indicate that A.tataricus is primarily used for phlegm removal, cough relief, lung relaxation, and asthma alleviation. Under normal circumstances, coughs are classified as either dry or wet, with different pathogenesis depending on their underlying causes (Asthma Group R. M. B., 2022). Several studies have shown that A.tataricus processed with honey has better expectorant and antitussive effects than raw products (Wu et al., 2006). The antitussive effect of A.tataricus has two main aspects, as described below.
7.2.1 Anti-inflammatory effect
Sputum and repeated coughing are two typical clinical symptoms of respiratory mucus hypersecretion and chronic non-specific inflammation of surrounding tissues, which can be attributed to chronic inflammatory respiratory diseases (Zhang and Zhou, 2014; Deng et al., 2023). Studies have shown that the TLR4/MyD88/NF-κB pathway is one of the pathogenesis pathways of bronchitis (Wu et al., 2020). Wu et al. (2023) conducted research evaluating the anti-inflammatory properties of Zi Wan San (RA and Tussilago farfara L), focusing on the TLR4/MyD88/NF-κB signaling pathway. The investigation revealed that administering various dosages of Zi Wan San led to significantly reduced TGF-β1, IL-1β, and IL-6 concentrations, alongside a notable decrease in the overall count and types of white blood cells. This reduction was also accompanied by improved lung tissue pathology, lower scores of airway goblet cell proliferation scores, and a decreased in the expression levels of TLR4, MyD88, and NF-κB proteins. Consequently, A.tataricus demonstrates the potential to mitigate airway inflammation in chronic bronchitis through its interaction with the TLR4/MyD88/NF-κB signaling pathway. And Ai and Li (2023) found that shionone can reduce reactive oxygen species (ROS) levels, inhibit OVA-induced oxidative stress response and NF-κB signaling pathway in young asthmatic rats, and effectively relieve asthma. Its pharmacological effects are similar to those of dexamethasone.
7.2.2 Vasodilation of bronchial smooth muscles
Cough-variant asthma (CVA) is a special type of asthma with cough as the main symptom and is often chronic and refractory. Irritating dry cough is the main clinical manifestation. In Western medicine, bronchodilators are typically used to treat this condition (Asthma Group R. M. B., 2022). Analysis of 218 prescriptions revealed that A.tataricus appeared 205 times during the treatment of CVA (Song C. et al., 2024). Peng et al. (2016a) observed the function of isolated guinea pig trachea smooth muscles and found that high doses of ATEE almost completely inhibited the contraction of isolated guinea pig smooth muscles caused by Ach, histamine, and CaCl2. ATEE exhibited a significant non-competitive and coercive effect on these substances, leading to the relaxation of bronchial smooth muscles and exerting an antitussive effect. Chen (2019) verified that ATE eluted using 75% ethanol can not only effectively inhibit the spontaneous contraction of isolated guinea pig tracheal smooth muscle, but also considerably inhibit the contraction of tracheal smooth muscle caused by various agonists, and block the Ca2+ channel of smooth muscle cells. It antagonizes the muscarine or histamine receptors on the smooth muscle, inhibits the inflow of Ca2+ into the cells, relaxes the lungs, and relieves asthma (Figure 14).
7.3 Anti-tumor effect
Bioactive compounds of A.tataricus exert inhibitory effects against diverse tumor types, including gastric, lung, prostate, skin, and breast cancers and glioma (Li et al., 2022b). They mainly induce tumor cell apoptosis, inhibit tumor cell proliferation and migration, and maintain the dynamic balance of oxidative stress in the tumor microenvironment.
Gastric cancer, a formidable global health challenge, stands as the predominant cause of cancer-associated deaths worldwide. Zhang Y. et al. (2012) demonstrated that WATP exhibits potent tumor growth inhibitory effects against the human gastric cancer cell line SGC-7901 while maintaining a non-toxic profile. This effect is achieved by inducing apoptosis via Ca2+ and △Ψ m-dependent mechanisms. The administration of ATE demonstrates its capability to suppress the Wnt/β-catenin signaling pathway and the expression of VEGF and Ki-67 proteins, thereby inhibiting the proliferation and invasion of lung adenocarcinoma A549 cells. Moreover, it successfully limits the expansion and movement of cancerous tissue while not causing major toxic effects on regular embryonic lung fibroblasts (Yao et al., 2022). In 2021, Chen T. et al. (2022) studied the discovery of a new bioflavonoid compound in A.tataricus. This compound primarily inhibited the proliferation and migration of A549 cells, inducing cell death through a non-apoptotic mechanism and thereby inhibiting the spread of lung and pancreatic cells. Liu et al. (2017) demonstrated in vitro that different concentrations of honey-processed A.tataricus decoction had a specific inhibitory effect on the proliferation of breast cancer MCF-7 cells in the G1 phase. Moreover, a certain concentration of honey-processed A.tataricus decoction can also effectively inhibit the migration of colorectal cancer LOVO cells, thereby preventing the recurrence of colorectal cancer (Qian et al., 2015; Yang et al., 2015). Clonogenic ability, an important characteristic of cancer cells, was also targeted, as ATE effectively inhibited the growth of oral squamous cell carcinoma SCC-9 cells by exerting cytotoxicity and anti-clonogenic activity (Wang et al., 2017).
ATP-II in A.tataricus effectively inhibited the in vitro proliferation of glioma C6 cells. In vivo, studies have demonstrated that ATP-II effectively suppresses the growth of glioma C6-implanted tumors and promotes apoptosis in tumor cells by enhancing the Bax/Bcl-2 ratio and stimulating the activities of Caspase-3, 8, and 9. Both strategies led to a robust apoptotic response, characterised by the swift activation of Caspase-3, an effect that was further amplified by the suppression of the survival kinase Akt. This research highlighted that the ability of ATP-II to effectively downregulate Akt effectively represents a distinctive factor contributing to the reduction of tumor growth (Du et al., 2014).
Cyclic polypeptides in A.tataricus exhibit desirable anti-tumor effects, and their activity depends on their cyclic nature. Proline dichloride residues prepared via chemical transformation and liver microsomal biotransformation on various homologues sin rats show that 1,2-cis dechlorinated proline residues play an important role in the anti-tumor activity of astin A, B, and C, and have good inhibitory activity against sarcoma 180A (Morita et al., 1996). Studies on the anti-mice ascites tumor cells of astins have shown moderate anti-tumor activity, with the activity of astin A and B being approximately 10 times that of astin C (Morita et al., 1993). The backbones of astin B and astin C were modified using Lawesson’s reagents, and the resulting thioderivatives showed higher anti-tumor activity than the corresponding parent astin (Cozzolino et al., 2005). Moreover, the main compound of the endophytic bacterium Cyanodermella astris isolated from A.tataricus is the bisanthraquinone skyrin, which has anti-cancer activity (Jahn et al., 2017).
7.4 Anti-oxidation and anti-aging effects
Du et al. (2017) found that ATS can enhance the activities of superoxide dismutase (SOD), glutathione peroxidase, and catalase (CAT) in the retinas of streptozotocin-induced diabetic rats and reduced oxidative stress, thus protecting the retina of diabetic rats. Different compounds isolated from different herbs have different effects on combating hemolysis and lipid peroxidation (Yu and Liu, 2009). Ng et al. (2003) showed that quercetin and kaempferol in A.tataricus exhibit potent antioxidative capabilities, demonstrating their effectiveness in hindering hemolysis, lipid peroxidation, and the production of superoxide free radicals. Scopoletin and emodin demonstrated efficacies comparable to those of quercetin and kaempferol in the prevention of superoxide radical formation, albeit with lower potency in the inhibition of lipid peroxidation. Aurantiamide acetate exhibited a remarkable ability to reduce superoxide radical formation, whereas 1,7-dihydroxy-6-methylanthraquinone was specifically effective in inhibiting superoxide radical formation alone.
Epifriedelanol, a key component of ATE, demonstrates efficacy in mitigating adriamycin-induced cellular senescence through its ability to suppress SA-β-gal activity and minimize the production of ROS, alongside reducing the activity of p53 protein and the levels of p21 in both human fibroblasts and human umbilical vein endothelial cells (Yang et al., 2011). The family of sirtuin genes, encompassing SIRT1, SIRT2, SIRT3, and SIRT6, participates in cellular activities like repairing DNA damage, aging, and apoptosis and is crucial in reproductive and developmental processes (Wątroba et al., 2017). Ren et al. (2023) has been highlighted by studies involving mature sow oocytes in vitro that epifriedelanol when derived from A.tataricus, exhibits notable effects. It can augment the antioxidant and anti-apoptotic functions of oocytes by upregulating SIRT family gene expression, thereby extending the in vitro culture age of pig oocytes.
7.5 Treatment of osteolytic diseases
RANKL and macrophage colony-stimulating factors regulate monocyte differentiation into osteoclasts (Silbermann et al., 2014; Wu et al., 2017; Zou et al., 2008). To investigate the effect of aster shionoside A2 on RANKL-induced RAW264.7 cells and Bone Marrow Macrophages (BMM), discovered that administering aster shionoside A2 significantly reduced the phosphorylation levels of ERK1/2, JNK, and p38 proteins, inhibited osteoclast-related gene activation in reaction to RANKL, and impeded the transcription and translation of NFATC1 and c-fos throughout the osteoclast differentiation phase (Su et al., 2022). Subsequently, Lee et al. (2023b) studied the mechanism of ATEE in alleviating osteoporosis, and demonstrated that it significantly inhibited the expression of key osteoclast factor NFATc1, which is induced by RANKL-mediated upregulation of c-Fos and NFATc1 proteins during osteoclast formation. Additionally, ATEE inhibited RANKL expression and RANK-induced osteoclast formation to delay osteoclast differentiation. It also inhibited the expression of RANKL mRNA driven by VitD3 in MLO-Y4 cells, and reduced VitD3-induced osteoclast formation in a co-culture of BMM and MLO-Y4 cells.
7.6 Treating urinary system diseases
A.tataricus alleviates urological-related diseases and has been clinically proven to have satisfactory effects. There is a record in Materia Medica Tongxuan: A.tataricus is spicy but not dry, moist but not cold, and tonic but not stagnant. However, its use is typically not immediate. For patients experiencing difficulty in urinating, one or two are recommended for symptom relief (Yu et al., 2023). Clinically, A.tataricus has been shown to effectively treat haematuria (Huang, 1990).
ATE safeguards bladder urothelial cells against pyroapoptosis. Through its mechanism of decreasing bladder swelling and bleeding in SD rats, lowering inflammatory markers and histopathological damage, and suppressing the activity of cell proapoptosis-related proteins while highlighting the role of the NLRP3/GSDMD-N pathway, ATE demonstrates a protective function against bladder injury. This leads to an enhancement of cell viability, reduction in the rate of pyroapoptosis in SV-HUC-1 cells, and ultimately, a beneficial effect in mitigating urinary retention, showcasing its therapeutic potential (Wang X. et al., 2020).
Research into the mechanism by which ATE curtails testosterone propionate-induced benign prostatic hyperplasia (BPH) in rats has revealed that A.tataricus exhibits therapeutic potential in BPH by enhancing apoptosis and suppressing inflammatory responses, as evidenced by substantial reductions in prostate weight, serum testosterone, and dihydrotestosterone levels, alongside inhibiting prostate epithelial thickening and the upregulation of proliferating cell nuclear antigen in rats. It reduces the expression of Bcl-2, increasing the expression of Bax, and significantly decreases the Bcl-2/Bax ratio. Moreover, it attenuates pro-inflammatory cytokine levels, specifically IL-1β, IL-6, and TNF-α levels, and reduces the expression of COX-2 and iNOS (Rho et al., 2020).
7.7 Acute lung injury
Shionone reduced histopathological changes, pulmonary oedema, and MPO activity and increased the percentage of neutrophils and macrophages in BALF. In addition, it inhibited pro-inflammatory cytokines, enhanced anti-inflammatory cytokines, and converted the M1 phenotype into M2 phenotype macrophages. The overexpression of ECM1 in vivo and in vitro confirmed that the regulatory effect of shionone could be attributed to ECM1 signaling transduction. Shionone weakens the ECM1/STAT5/NF-κB pathway, thereby improving acute lung injury (ALI) caused by sepsis (Song et al., 2021). Another study pointed out that shionone could target pneumohaemolysin in vivo and in vitro, thereby improving ALI caused by Streptococcus pneumoniae (Du et al., 2022). Chen et al. (Chen et al., 2019) analyzed ALI induced by LPS-induced mice using network pharmacology and found that Fr-75 eluted by ATE reduced the number of white blood cells in BALF by 39.2%, macrophages by 51.8%, neutrophils by 63.8%, and lymphocytes by 43.6%. It protects against LPS-induced MPO by reducing the severity of lung lesions, pulmonary oedema, release of inflammatory cells, and expression of ALI. Fr-75 contains many regulatable targets and pathways that may regulate, of which 31 targets are related to multiple components, such as VEGFA and NF-κB pathways. In 2024, research demonstrated that shionone is a viable therapeutic agent for addressing pulmonary inflammation and fibrosis in silicotic mice. Shionone, through its activation of the Nrf2 pathway, safeguards against SO2-induced oxidative stress and inflammation in macrophages and prevents fibroblast-to-myofibroblast transition triggered by TGF-β (Wang et al., 2024).
7.8 Others
In addition to the above pharmacological effects, Wu et al. (2021) found that A.tataricus can relieve constipation by antagonizing the binding of Ach to the AChR and inhibiting Ca2+ influx. In South Korea, owing to differences in the growth environment of A.tataricus, its leaves have a good recovery effect on memory dysfunction. In their investigation of the pharmacological mechanisms, Lee S. E. et al. (2023) demonstrated that ATE exerts its effects through the modulation of hippocampal cholinergic activity and anti-apoptotic signaling pathways. Specifically, their research revealed that ATE regulates choline acetyltransferase activity and influences the expression of Bcl2-associated anti-apoptotic genes during scopolamine-induced cognitive deficits in murine models. Furthermore, the study identified significant alterations in neuroactive ligand-receptor interaction-related genes, including Npy2r, Htr2c, and Rxfp1. Importantly, ATE administration was shown to mitigate amyloid-β protein aggregation and prevent neuronal apoptosis in transgenic mouse models, resulting in substantial improvements in memory-related cognitive functions.
In vitro, ATE exerted a growth-promoting effect on Bifidobacterium and an inhibitory effect on Clostridium perfringens. Therefore, A.tataricus can have a probiotic effect on intestinal flora (Park et al., 1993). Furthermore, terpenoids in A.tataricus have certain antiviral activity, especially against HBV. Studies have found that (Zhou et al., 2013) astataricusones B exerts potent antiviral effects through dual mechanisms: primarily by suppressing the secretion of hepatitis B virus surface antigens (HBsAg and HBeAg) and subsequently inhibiting viral DNA replication. Furthermore, pharmacological investigations demonstrated that ATE-derived polyphenolic compounds exhibit remarkable metabolic regulatory properties, as evidenced by their ability to significantly attenuate body weight gain and modulate blood glucose levels in rodent models (Du et al., 2017).
8 Acute toxicology
A.tataricus has a history of over 2000 years and is generally considered clinically safe. Chp recommends a dosage of 5–10 g for A.tataricus (Commission N. P, 2020). S. Kosemura et al. (Morita et al., 1993; Yoshioka et al., 1973) found that Astin has a similar structure to that of cyclochloridine, the hepatotoxic metabolite of penicillin. Thus, A.tataricus could be toxic and may cause liver damage (Liu et al., 2012). Subsequently, refer to Table 4 for the related toxicity experiment of A.tataricus. For the toxicity component screening experiment of A.tataricus, the 90% ethanol extract of A.tataricus was separated into petroleum ether, ethyl acetate, n-butanol, and water fractions using an extraction method. Using acute toxicity in mice as the indicator, the results showed that the ethyl acetate fraction was the toxic fraction of A.tataricus, with an LD50 of 0.052 g/kg. After administration of this fraction, the mice exhibited markedly reduced activity, listlessness, unkempt and wet fur, closed eyes, and involuntary tremors until respiratory arrest (Zhang et al., 2007). Through acute toxicity experiments, Shao et al. (2009) showed that ATEE toxicity was greater than that of ATE, with toxicity levels increasing as ethanol concentration increased. Among the tested ethanol concentrations, 90% ethanol extract was found to be the most toxic. Acute toxicity tests were conducted on the water extract, 75% ethanol extract, and 80% ethanol extract of A.tataricus. Most mice exhibited abnormal behaviors post-administration, such as delayed movement, listlessness, and refusal to eat. The LD50 values were 31.61 g/kg, 15.74 g/(kg·bw), and 19.19 g/kg, respectively. For the 75% ethanol extract-treated mice, autopsy revealed darkened liver color, blunt margins, and obvious swelling, congestion, spots, and bleeding points (Liu et al., 2013; Peng, 2016). The toxicity in ATEE mainly came from the cancer fraction Fr-2 compound, which increased the levels of aminotransferase, aspartate aminotransferase, and alkaline phosphatase. A histopathological examination of the liver confirmed that the compound is hepatotoxic. Subsequent screening and analysis of the hepatotoxic compounds revealed that Fr-2 is primarily a peptide compounds (Liu et al., 2012). Fr-2 induces toxicity in human L-02 liver cells via oxidative stress. Intracellular ROS levels peak at 40 μg/mL. It triggers liver cell apoptosis through a mitochondrial-dependent pathway in a dose-dependent manner. At 100 μg/mL, the mitochondrial membrane potential drops from 5.29% to 39.83%, and apoptosis-related proteins are activated (Wang et al., 2014b). This is consistent with a previous discovery that Astin B can induce apoptosis and autophagy in human liver L-02 cells and is mediated by mitochondrial/caspase-dependent apoptosis in vivo and in vitro, showing hepatotoxicity results (Wang et al., 2014a). Studies on the combined toxicity of A.tataricus and T.farfara show that combining different solvent extracts of T.farfara with the 90% ethanol extract of A.tataricus in a 1:1 ratio by raw material weight can significantly reduce liver damage caused by A.tataricus (Zhang et al., 2007). In addition to cyclic peptides, 11 anti-HBV shionane-type triterpenoids in A.tataricus were studied to identify that astaricusones B, epifriedelan, and astershionones C were cytotoxic to HepG2.2.15 cells (Zhou et al., 2013; Zhou et al., 2014). The saponins in A.tataricus have a hemolytic effect, so its crude extract is not suitable for intravenous use (Wang, 1983).
Although extensively used in traditional medicine and generally considered safe, A.tataricus has potential hepatotoxicity and hemolytic properties that should not be overlooked. Its toxic effects are more pronounced at high doses or with long-term use. To ensure its clinical safety, more comprehensive and reliable data are needed to clarify its potential toxicity and explore more effective detoxification methods. Additionally, combining A.tataricus with other Chinese herbs like Tussilago farfara could be an effective way to reduce toxicity.
9 Comprehensive utilization
9.1 Traditional food applications
In countries such as China, North Korea, and Japan, A.tataricus is often consumed as a vegetable. According to documents issued by the National Health Commission of China and the State Administration of Market Supervision of China, A.tataricus is approved for use as both medicine and food within a limited scope of use and dose (Wang et al., 2018). Generally, young leaves harvested in the spring are generally consumed as vegetables. Fresh vegetables can be washed, blanched with boiling water, and soaked in clear water overnight. They can be dried and left for later consumption as dried vegetables, soaked and eaten directly as soups or dipped in sauce, and soaked in scented tea and made into Zi Wan tea (Chen and Huang, 2010). In South Korea, A.tataricus is among the most popular wild vegetables (Ahn et al., 2018) used to make traditional Korean dishes (Chung et al., 1993).
9.2 Essential oils
The concentrated essential oil of A.tataricus maintains the same taste as that of fresh plants, with a woody or herbal aroma (Choi, 2012). Chung et al. (1993) determined that monoterpenes and sesquiterpenes are the primary volatile components in A.tataricus extracts. Among these, myrene, limonene, and germanene D were identified as the predominant volatile compounds within A.tataricus essential oils.
9.3 Ornamental plants
A.tataricus can be used as an ornamental flower in the autumn. When its lavender flowers are in full bloom, they contrast the high and dark blue sky in autumn and have an extremely high ornamental value. In the gardens of European and American countries, A.tataricus is an important cultivated variety, in China, it is recommended for use in flowerbeds due to its rapid growth, ease of management, light preference, and drought tolerance (Yang, 2003). In South Korea and Japan, A.tataricus is a common autumn wildflower (Lee et al., 2021). For this reason, Japan has also developed the unique color of Aster-shion-iron (dyed by RA and Camellia japonica gray juice as a mordant). Considered a symbol of peace (Chen, 1985).
9.4 Others
Clonorchiasis sinensis is an important food-borne zoonotic parasite, highly associated with liver fibrosis and cholangiocarcinoma (Bao et al., 2024). Bao et al. (2024) observed the insecticidal effects of ATE on Clonorchiasis sinensis in vitro.
Additionally, oxidative rancidity is the main reason for the deterioration of the nutritional quality of grease and oily foods (Hu et al., 2022). Chen et al. (2012) found that ATE has a good antioxidant effect on peanut oil, and the increase in the concentration of the extract is positively correlated with the anti-oxidation effect of peanut oil. Therefore, the A.tataricus extract can be used instead of artificial antioxidants, which can not only extend the life of oil products, but also be harmless to human health.
10 Conclusion and prospects
Medicinal plants are esteemed for their pivotal role in human health, serving as a fundamental source of bioactive compounds that are harnessed to prevent, manage, and cure a wide array of common ailments (Dsouza et al., 2024). A.tataricus has a variety of chemical components, so its medicinal value is very high. As a medicinal and edible plant, A.tataricus has been used for over 1700 years since its medicinal effects were discovered. Ethnocpharmacology believes that it mainly has the effects of warming the lungs, lowering the qi, eliminating phlegm, and relieving cough. Modern clinical research has demonstrated its therapeutic effect against inflammatory diseases, particularly as an expectorant and antitussive. It is the preferred choice for many pharmaceutical companies to produce drugs to moisten the lungs, eliminate phlegm, relieve coughs, and treat asthma (Wei et al., 2023).
Although the root of A.tataricus is found in many proprietary Chinese medicines for relieving cough and asthma, with the progress of science and technology, modern studies have revealed that it contains more than 200 kinds of natural metabolites, among which triterpenoids (such as the iconic component shionone), flavonoids and cyclic peptides play anti-inflammatory, antioxidant and anti-tumor effects through multi-target synergies. Notably, ChP has designated shionone as a quality marker due to its role as a core anti-inflammatory component in A.tataricus, and the underlying mechanism has been extensively elucidated. Cyclopeptides exhibit “double-edged sword” properties, demonstrating significant anticancer effects; however, they share structural similarities with cyclochloroside, a hepatotoxic metabolite of penicillin, which can cause liver damage. Toxicological studies have also explored the mechanisms of liver damage associated with astin. To ensure clinical safety, more comprehensive and reliable data are required to clarify potential toxicity and develop more effective detoxification methods. Further research is essential to understand the efficacy of A.tataricus and its bioactive compounds fully. For instance, the antiviral properties of A.tataricus warrant additional validation. This will provide a novel model for integrating traditional medical theory with modern pharmacology.
As a natural herb, A.tataricus possesses edible aerial parts and valuable underground medicinal components, both of which exhibit excellent health benefits and thus represent a promising resource for the development of functional foods and innovative medicines. In this paper, we have reviewed the planting methods of A.tataricus; however, current research on its cultivation remains limited, and studies exploring the relationship between planting techniques and pharmacological activity are scarce. Factors such as the origin (Xiu et al., 2006), harvest period (Zhang et al., 2021), processing methods (Wang J. et al., 2023), and storage conditions of A.tataricus may lead to potential ‘deterioration’. To investigate the effects of planting time, density, and environmental factors on A.tataricus, further research is needed to ensure high-quality and stable sustainable development. Additionally, integrating network pharmacology with multi-omics approaches can facilitate the exploration of innovative drugs derived from A.tataricus, thereby promoting the modernization of A.tataricus research (Marmouzi et al., 2021; Zhang et al., 2024). In summary, this paper comprehensively reviews and analyzes botany, cultivation techniques, phytochemistry, ethnopharmacology, pharmacology, and comprehensive utilization of A.tataricus, providing valuable insights for its further development.
Author contributions
XnZ: Data curation, Writing – original draft. ML: Supervision, Writing – original draft. XaZ: Supervision, Writing – original draft. LM: Conceptualization, Data curation, Writing – original draft. SS: Investigation, Writing – original draft. MP: Data curation, Writing – review and editing. SH: Investigation, Writing – original draft. WR: Methodology, Writing – review and editing. WM: Funding acquisition, Resources, Writing – review and editing.
Funding
The author(s) declare that financial support was received for the research and/or publication of this article. This work was supported by Heilongjiang Province “double first-class” discipline collaborative innovation achievement project:Quality optimization and deep processing of characteristic Chinese medicinal materials in the lower cold region of great health industry, Project No. LJGXCG2023-058; Heilongjiang Touyan Innovation Team Program (Grant Number: [2019] No. 5); National Key Research and Development Program, Research and Demonstration of Collection, Screening and Breeding Technology of Ginseng and other Genuine Medicinal Materials, Project (Grant Number: 2021YFD1600901).
Acknowledgments
Gratitude is extended to every lab member for their valuable insights and feedback on the manuscript.
Conflict of interest
The authors declare that the research was conducted in the absence of any commercial or financial relationships that could be construed as a potential conflict of interest.
Generative AI statement
The author(s) declare that no Generative AI was used in the creation of this manuscript.
Publisher’s note
All claims expressed in this article are solely those of the authors and do not necessarily represent those of their affiliated organizations, or those of the publisher, the editors and the reviewers. Any product that may be evaluated in this article, or claim that may be made by its manufacturer, is not guaranteed or endorsed by the publisher.
Supplementary material
The Supplementary Material for this article can be found online at: https://www.frontiersin.org/articles/10.3389/fphar.2025.1581505/full#supplementary-material
References
Ahn, S. H., Moon, J. S., Lee, Y. M., Yang, J. H., Kim, J., and Kim, H. J. (2018). (Year) published. Selection of suitable wild herbs and vegetables for cultivation of sub-highland. Proceedings of the plant resources society of Korea conference. Plant Resour. Soc. Korea, 53.
Ai, K., and Li, Z. (2023). -The effects of shionone on nf-κb pathway and oxidative stress. Ovalbumin-Induced Asthmatic Immature Rats. Curr. Immunol. 43, 226–232.
Akihisa, T., Kimura, Y., Tai, T., Arai, K. J. C., and Bulletin, P. (1999). Astertarone B, a hydroxy-triterpenoid ketone from the roots of aster tataricus L. A Hydroxy-Triterpenoid Ket. Roots Of Aster Tataricus L 47, 1161–1163. doi:10.1248/cpb.47.1161
Asthma Group, R. M. B. (2022). Chinese medical association. Guidelines for the diagnosis and treatment of cough (2021). Chin. J. Of Tuberc. And Respir. 45, 13–46.
Bao, P., Wang, X., Zhang, X., Yu, Y., Ma, Y., Zhang, H., et al. (2024). Clonorchis sinensis aggravated liver fibrosis by activating parp-1 signaling to induce parthanatos via dna damage. Vet. Parasitol. 330, 110217. doi:10.1016/j.vetpar.2024.110217
Cai, Y., Shi, X., Liu, H., Wu, L., Gu, Y., and Wang, R. (2023). Eff. Components And Pharmacol. Eff. Of Asteris Radix J. Of Traditional Chin. Veterinary Med. 42, 39–42. doi:10.13823/J.Cnki.Jtcvm.2023.039
Chen, R., Liu, Y. F., and Huo, L. N. (2012). Antioxidant activity of extracts from aster tataricus. L.F. For oil technology and development of chemical industry, 41, 4–6.
Chen, T., Yang, P., Chen, H. J., and Huang, B. (2022a). A new biflavonoids from aster tataricus induced non-apoptotic cell death in A549 cells. Nat. Prod. Res. 36, 1409–1415. doi:10.1080/14786419.2021.1882456
Chen, W., Yang, Y., Fu, K., Zhang, D., and Wang, Z. (2022b). Progress in icp-ms analysis of minerals and heavy metals in traditional medicine. Front. Pharmacol. 13, 891273. doi:10.3389/Fphar.2022.891273
Chen, W. Z., Guo, W. N., and Wang, R. (2020). “Study on the dynamic accumulation of chief chemical constituents of aster tataricus L,” in F. ’Underground organs, 42. Journal Of Yichun University, 27–30.
Chen, Y., Dong, J., Liu, J., Xu, W., Wei, Z., Li, Y., et al. (2019). Network pharmacology-based investigation of protective mechanism of aster tataricus on lipopolysaccharide-induced acute lung injury. Int. J. Mol. Sci. 20, 543. doi:10.3390/Ijms20030543
Chen, Y. J. (2019). “Study on the pharmacodynamic material basis and mechanism of aster tataricus L. F. On moistening lung and relieving asthma,” in Doctor's degree. Beijing University Of Chinese Medicine.
Chen, Y. M., and Huang, H. B. (2010). Health protection and treatment of aster tataricuse L. F. Chin. Med. And Cult. 5, 47.
Cheng, D., and Shao, Y. (1994). Terpenoid glycosides from the roots of aster tataricus. Phytochemistry 35, 173–176.
Choi, H.-S. J. A. C. L. (2012). Comparison of the essential oil composition betweenAster tataricusandA. Koraiensis. Koraiensis 2, 138–151. doi:10.1080/22297928.2000.10648262
Chung, T. Y., Eiserich, J. P., and Shibamoto, T. (1993). Volatile Compd. Isol. Edible Korean Chamchwi (Aster Scaber Thunb) 41, 1693–1697.
Commission, N. P. (2020). Pharmacopoeia of the People's Republic of China, China China National Pharmaceutical Science And Technology Press.
Cozzolino, R., Palladino, P., Rossi, F., Calì, G., Benedetti, E., and Laccetti, P. (2005). Antineoplastic cyclic astin analogues kill tumour cells via caspase-mediated induction of apoptosis. Carcinogenesis 26, 733–739. doi:10.1093/Carcin/Bgi017
Deng, Z., Liu, Y., Zhou, S., and Liu, F. (2023). Master of traditional Chinese medicine Liu zuyi's experience in. Treat. And Prev. Chronic Bronchitis Asia-Pacific Traditional Med. 19, 92–94.
Ding, Z. (2021). “Study on geographical identification and the element distribution of Poria cocos based on multi-element combined chemometrics,” in Master's degree master's degree. Anhui University Of Chinese Medicine.
Dong, Y., Liu, Y., Tang, J., Du, J., Zhuang, X., Tan, S., et al. (2024). Zhisou powder displays therapeutic effect on chronic bronchitis through inhibiting pi3k/akt/hif-1α/vegfa signaling pathway and reprograming metabolic pathway of arachidonic acid. J. Ethnopharmacol. 319, 117110. doi:10.1016/J.Jep.2023.117110
Dsouza, A., Dixon, M., Shukla, M., and Graham, T. J. J. O. E. B. (2024). Harnessing controlled environment systems for enhanced production of medicinal plants. Erae248.
Du, H., Zhang, M., Yao, K., and Hu, Z. (2017). Protective effect of aster tataricus extract on retinal damage on the virtue of its antioxidant and anti-inflammatory effect in diabetic rat. Biomed. Pharmacother. 89, 617–622. doi:10.1016/J.Biopha.2017.01.179
Du, L., Mei, H. F., Yin, X., and Xing, Y. Q. (2014). Delayed growth of glioma by A polysaccharide from aster tataricus involve upregulation of bax/bcl-2 ratio, activation of caspase-3/8/9, and downregulation of the Akt. Tumor Biol. 35, 1819–1825. doi:10.1007/S13277-013-1243-8
Du, R., Wang, T., Lv, H., Zou, Y., Hou, X., Hou, N., et al. (2022). Shionone-targeted pneumolysin to ameliorate acute lung injury induced by Streptococcus pneumoniae in vivo and In Vitro. Molecules 27, 6258. doi:10.3390/Molecules27196258
Fan, L., Wang, X., Zhu, X. J., Liu, J., Yin, H., Luo, J. P., et al. (2018). Effects of different processing methods on content of extract and main components of A.tataricus. Mod. Chin. Med. 20, 1509–1514. doi:10.13313/J.Issn.1673-4890.20180630002
Fan, X., Qin, Z., Wen, J., Wang, Z., and Xiao, W. (2024). An updated and comprehensive review of the morphology, ethnomedicinal uses, phytochemistry, and pharmacological activity of aster tataricus L. F. Heliyon 10, E35267. doi:10.1016/J.Heliyon.2024.E35267
Gao, J. H., Wang, H. W., and Song, G. Q. (1994). Structure and stereochemistry analysis of two compounds from aster tataricus by nmr. Chin. J. Of Magnetic Reson., 391–398.
Gao, W. Y., Zhang, R., Jia, W., J, P. L., Lu, B., Zheng, Z. Y., et al. (2003). Determination of shionone content in root of aster tataricus by hplc Chinese traditional and herbal drugs, 92–93.
Guo, W. N., Cheng, L., and Fang, C. W. (2016). Studies on Structure,Accumulation of chief chemical constituents and content of aster tataricus L. F.Maternal root. Lishizhen Med. And Materia Medica Res. 27, 2614–2616.
Han, Y., Gao, F., Qin, P., Bai, R., Tian, J., Liu, X., et al. (2023). Advances in studies on asteris radix et rhizoma and prediction of its quality markers. Mod. Chin. Med. 25, 655–664. doi:10.13313/J.Issn.1673-4890.20211115004
Hu, G. (2017). “Study on inorganic elements and quality analysis of polygonum cuspidatum in shitai of Anhui Province,” in Master’s degree. Anhui University Of Traditional Chinese Medicine.
Hu, W. N., Xue, Y. T., Wang, X. L., Hao, X. Q., Wang, Z. Q., and Song, M. P. (2022). Research Progress In Edible oil storage modern chemical research, 16–18.
Huang, L. Q., Guo, L. P., and Zhan, Z. L. (2020). Compendium of standards for genuine medicinal materials. Beijing: Beijing Science And Technology Press.
Huang, M. (1990). Reuse aster to treat blood in. Urine Journal Of Shanxi University Of Traditional Chinese Medicine, 26.
Huang, S. S., Gao, Y., Li, W. M., and Guan, H. H. (2008). Determination of total triterpenes from radix aster is by spectrophoto metry lishizhen medicine and materia Medica research, 1406–1407.
Jahn, L., Schafhauser, T., Wibberg, D., Rueckert, C., Winkler, A., Kulik, A., et al. (2017). Linking secondary metabolites to biosynthesis genes in the fungal endophyte Cyanodermella asteris: the anti-cancer bisanthraquinone skyrin. Anti-Cancer Bisanthraquinone Skyrin 257, 233–239. doi:10.1016/j.jbiotec.2017.06.410
Jaiswal, V., and Lee, H. J. (2023). Pharmacological properties of shionone: potential anti-inflammatory phytochemical against different diseases. Molecules 29, 189. doi:10.3390/Molecules29010189
Jia, K., Zhang, X., Meng, Y., Liu, S., Liu, X., Yang, T., et al. (2023). Metabolomics and transcriptomics provide insights into the flavonoid biosynthesis pathway in the roots of developing aster tataricus. J. Plant Res. 136, 139–156. doi:10.1007/S10265-022-01426-4
Jia, S. X. (2015). Transforming the significance of medicinal substances, zhongguo zhong Yi yao chu ban she.
Jin, J., Moore, M. K., Wilson, W. K., and Matsuda, S. P. T. (2018). Astertarone A synthase from Chinese cabbage does not produce the C4-epimer: mechanistic insights. Org. Lett. 20, 1802–1805. doi:10.1021/Acs.Orglett.8b00302
Jin, J., Zhang, C. F., and Zhang, M. (2008). Study on chemical constituents from aster tataricus L.F. Mod. Chin. Med., 20–22. doi:10.13313/J.Issn.1673-4890.2008.06.012
Kang, K. B., Lee, D. Y., and Sung, S. H. (2021). LC–MS/MS-Based comparative investigation on chemical constituents of six aster species occurring in Korea. Korea. Nat. Product. Sci. 27, 257–263. doi:10.20307/nps.2021.27.4.257
K., D. (2023). Aster, A neglected family of compositae flowers China flowers and horticulture, 30–33.
Lanzotti, V. J. P. R. (2005). Bioactive saponins from allium and aster plants. Bioact. Saponins Allium And Aster Plants 4, 95–110. doi:10.1007/s11101-005-1254-1
Lee, J. S., Lee, C. K., Choi, Y. J., Shin, H. D., and Reports, J. N. D. (2021). Pucciniastrum verruculosum causing rust disease on aster tataricus in Korea. 44, Na-Na.
Lee, S. E., Park, S., Jang, G. Y., Lee, J., Moon, M., Ji, Y. J., et al. (2023a). Extract of aster koraiensis nakai leaf ameliorates memory dysfunction via anti-inflammatory action. Int. J. Mol. Sci. 24, 5765. doi:10.3390/ijms24065765
Lee, S. J., Yang, H., Kim, S. C., Gu, D. R., Ryuk, J. A., Jang, S. A., et al. (2023b). Ethanol extract of radix asteris suppresses osteoclast differentiation and alleviates osteoporosis. Int. J. Mol. Sci. 24, 16526. doi:10.3390/Ijms242216526
Li, H., Li, W., Ding, X., Zhang, D., Xue, Z., An, Q., et al. (2024a). Herbal textual research on asteris radix et rhizoma. Famous Class. Formulas Chin. J. Of Exp. Traditional Med. Formulae 30, 20–30. doi:10.13422/J.Cnki.Syfjx.20230356
Li, H. Y., Guo, S., Yan, H., Yang, T., Yu, D. X., Zhan, Z. L., et al. (2022a). Content and distribution of inorganic elements in laminaria japonica based on icp-ms and micro-xrf. Zhongguo Zhong Yao Za Zhi 47, 444–452. doi:10.19540/J.Cnki.Cjcmm.20210803.203
Li, J., Chen, C., Ma, M., Li, P., Yang, S., Guo, R., et al. (2024b). Five new secondary metabolites from aster tataricus. Fitoterapia 174, 105828. doi:10.1016/J.Fitote.2024.105828
Li, K. J., Liu, Y. Y., Wang, D., Yan, P. Z., Lu, D. C., and Zhao, D. S. (2022b). Radix asteris: traditional usage, phytochemistry and pharmacology of an important traditional Chinese medicine. Molecules 27, 5388. doi:10.3390/Molecules27175388
Li, L., Chang, X., Cui, H., Sun, H., and Cao, T. (2023a). Comparative analysis of inorganic elements in difference officinal parts of aster tataricus by icp-ms with statistical analysis. J. Of Chin. Med. Mater. 46, 1447–1453. doi:10.13863/J.Issn1001-4454.2023.06.021
Li, S., Hong, Z., Wang, Z., Li, F., Mei, J., Huang, L., et al. (2018). The cyclopeptide astin C specifically inhibits the innate immune cdn sensor sting. Cell Rep. 25, 3405–3421. doi:10.1016/J.Celrep.2018.11.097
Li, X., Gong, Y., Feng, L., Wang, X., Wang, J., Zhang, A., et al. (2023b). Neuropyrones A-E, five undescribed Α-pyrone derivatives with tyrosinase inhibitory activity from the endophytic fungus neurospora dictyophora wz-497. Phytochemistry 207, 113579. doi:10.1016/J.Phytochem.2022.113579
Li, Y., Luo, K. P., Chen, Y. Y., Lin, Y., and Chen, Q. B. (2010). A brief analysis of the current research situation of aster tataricus in China. Guangxi J. Of Light Industry 26, 7–8.
Liu, F., Li, L. F., Liu, G. J., Jiang, H., and Zhang, Q. Z. (2013). Study on the acute toxicity of water and alcohol extract of radix asteris. Res. And Pract. Chin. Med. 27, 38–39. doi:10.13728/J.1673-6427.2013.06.018
Liu, W., Feng, Y., Yu, S., Fan, Z., Li, X., Li, J., et al. (2021). The flavonoid biosynthesis network in plants. Int. J. Mol. Sci. 22, 12824. doi:10.3390/Ijms222312824
Liu, X. D., Cao, P. P., Zhang, C. F., Xu, X. H., Zhang, M., Pharmaceutical, J. J. O., et al. (2012). Screening and analyzing potential hepatotoxic compounds in. Ethanol Extr. Of Asteris Radix By Hplc/Dad/Esi-Msn Tech. 67, 51–62.
Liu, X. L., Liang, Y. X., and Hu, X. H. (2017). Impacts of the decoction of honey processed radix asteris on human breast cancer mcf-7 cells in. Intro Experimen World J. Of Integr. Traditional And West. Med. 12, 496–499+512. doi:10.13935/J.Cnki.Sjzx.170412
Liu, Z., Xi, R., Zhang, Z., Li, W., Liu, Y., Jin, F., et al. (2014). 4-hydroxyphenylacetic acid attenuated inflammation and edema via suppressing HIF-1α in seawater aspiration-induced lung injury in rats. Rats. Int J Mol Sci 15, 12861–12884. doi:10.3390/Ijms150712861
Lu, Y. H., Dai, Y., Wang, Z. T., and Xu, L. S. (1999). Aster tataricuse for expectorant and antitussive use, and its effective parts and active ingredients. Chin. Herb. Med., 360–362.
Lu, Y. H., Wang, Z. T., Ye, W. C., Xu, L. S., and Shu, Y. Z. (1998). Studies on the constituents of aster tataricus L.F. Journal of China pharmaceutical university, 19–21.
Luo, M., Zhao, F., Cheng, H., Su, M., and Wang, Y. (2024). Macrophage polarization: an important role in inflammatory diseases. Front. Immunol. 15, 1352946. doi:10.3389/Fimmu.2024.1352946
Marmouzi, I., Bouyahya, A., Ezzat, S. M., El Jemli, M., and Kharbach, M. J. J. O. E. (2021). The food plant Silybum marianum (L.) Gaertn.: phytochemistry, Ethnopharmacology and clinical evidence. Ethnopharmacol. And Clin. Evid. 265, 113303. doi:10.1016/j.jep.2020.113303
Morita, H., Nagashima, S., Takeya, K., and Itokawa, H. (1993). Astins A and B, antitumor cyclic pentapeptides from aster tataricus. Chem. Pharm. Bull. (Tokyo) 41, 992–993. doi:10.1248/Cpb.41.992
Morita, H., Nagashima, S., Uchiumi, Y., Kuroki, O., Takeya, K., and Itokawa, H. (1996). Cyclic peptides from higher plants. Xxviii. Antitumor activity and hepatic microsomal biotransformation of cyclic pentapeptides, astins, from aster tataricus. Chem. Pharm. Bull. (Tokyo) 44, 1026–1032. doi:10.1248/Cpb.44.1026
Morris, S. M. (2016). Arginine metabolism revisited. J. Nutr. 146, 2579S–2586S. doi:10.3945/Jn.115.226621
Nagao, T., Hachiyama, S., Okabe, H., and Yamauchi, T. (1989). Stud. Const. Of Aster Tataricus Lf Ii. Struct. Of Aster Saponins Isol. Root 37, 1977–1983.
Ng, T. B., Liu, F., Lu, Y., Cheng, C. H., and Wang, Z. (2003). Antioxidant activity of compounds from the medicinal herb aster tataricus. Comp. Biochem. Physiol. C Toxicol. Pharmacol. 136, 109–115. doi:10.1016/S1532-0456(03)00170-4
Park, J. H., Han, N. S., Yoo, J. Y., and Kwon, D. J. (1993). Effect Of Aster Scaber Extract On The Growth Of Bifidobacteria And Clostridium Perfringens 3, 285–291.
Peng, W. J. (2016). Studies on isolated Guinea-pig tracheal smooth muscle and toxic effects of aster tataricus L. F. Master, Chin. Acad. Of Agric. Sci. Diss.
Peng, W. J., Xin, R. H., Liu, Y., Luo, Y. J., Wang, G. B., Luo, C. Y., et al. (2016a). Effects of alcohol extract of aster tataricus L.F. Contract. Of Guin. Pig Tracheal Smooth Muscle China Animal Husb. And Veterinary Med. 43, 1572–1578. doi:10.16431/J.Cnki.1671-7236.2016.06.026
Peng, W. J., Xin, R. H., Luo, Y. J., Liang, G., Ren, L. H., Liu, Y., et al. (2016b). Evaluation of the acute and subchronic toxicity of Aster tataricus L. F. Afr. J. Tradit. Complement. Altern. Med. 13, 38–53. doi:10.21010/Ajtcam.V13i6.8
Prockop, D. J., and Oh, J. Y. (2012). Mesenchymal stem/stromal cells (mscs): role as guardians of inflammation. Mol. Ther. 20, 14–20. doi:10.1038/Mt.2011.211
Qian, S. S., Peng, L., Yang, Y. Y., Qin, T. T., Liu, L. J., Bai, S. M., et al. (2015). Effects of honey-fried radix asteris decoction (hfrad) on cell apoptosis and migration in. Colorectal Cancer Lovo Cells 33, 2146–2150. doi:10.13193/J.Issn.1673-7717.2015.09.027
Ren, X., Yun, X., Yang, T., Xu, T., Shi, D., Li, X., et al. (2023). Epifriedelanol delays the aging of porcine oocytes matured invitro. Vitro 233, 107256. doi:10.1016/j.toxicon.2023.107256
Rhee, J. K., Woo, K. J., Baek, B. K., and Ahn, B. J. (1981). Screening of the wormicidal Chinese raw drugs on Clonorchis sinensis. Screen. Of Wormicidal Chin. Raw Drugs Clonorchis Sinensis 9, 277–284. doi:10.1142/s0192415x81000366
Rho, J., Seo, C. S., Park, H. S., Jeong, H. Y., Moon, O. S., Seo, Y. W., et al. (2020). Asteris radix et rhizoma suppresses testosterone-induced benign prostatic hyperplasia in rats by regulating apoptosis and inflammation. J. Ethnopharmacol. 255, 112779. doi:10.1016/J.Jep.2020.112779
Rossi, F., Zanotti, G., Saviano, M., Iacovino, R., Palladino, P., Saviano, G., et al. (2004). New antitumour cyclic astin analogues: synthesis, conformation and bioactivity. J. Pept. Sci. 10, 92–102. doi:10.1002/Psc.506
Sawai, S., Uchiyama, H., Mizuno, S., Aoki, T., Akashi, T., Ayabe, S., et al. (2011). Molecular characterization of an oxidosqualene cyclase that yields shionone, A unique tetracyclic triterpene ketone of aster tataricus. Febs Lett. 585, 1031–1036. doi:10.1016/J.Febslet.2011.02.037
Schafhauser, T., Jahn, L., Kirchner, N., Kulik, A., Flor, L., Lang, A., et al. (2019). Antitumor astins originate from the fungal endophyte Cyanodermella asteris living within the medicinal plant Aster tataricus. Antitumor Astins Orig. Fungal Endophyte Cyanodermella Asteris Living Within Med. Plant Aster Tataricus 116, 26909–26917. doi:10.1073/pnas.1910527116
Shao, J., Jin, J., Zhang, M., Huang, F., Dou, C. G., and Wei, Y. Y. (2009). Toxic Part Of radix asteris and antagonizing Part Of flos farfarae in pair-drug of ff and Ra. Lishizhen Med. And Materia Medica Res. 20, 1308–1310.
Silbermann, R., Bolzoni, M., Storti, P., Guasco, D., Bonomini, S., Zhou, D., et al. (2014). Bone Marrow monocyte-/macrophage-derived activin A mediates the osteoclastogenic effect of il-3 in multiple myeloma. Leukemia 28, 951–954. doi:10.1038/Leu.2013.385
Song, C., Yu, S., and Zhou, Y. (2024a). The experience of traditional Chinese medicine in treating cough variant asthma was discussed based on data mining technology. Asia-Pacific Tradit. Med. 20, 196–201.
Song, L., Hou, J. P., Li, H. B., Zhang, Y., and G., Y. X. (2009). Determ ination of quercetin in traditional Mongolian medicine astern by hplc. J. Of Inn. Mong. Med. Coll. 31, 571–574. doi:10.16343/J.Cnki.Issn.2095-512x.2009.06.007
Song, N. N., Du, L. J., Li, X. J., Tang, X., and Ke, J. H. (2024b). Research progress on the chemical components, pharmacological effects, and cultivation techniques of aster tataricus. South China Agric. 18, 109–115. doi:10.19415/J.Cnki.1673-890x.2024.15.023
Song, Y., Wu, Q., Jiang, H., Hu, A., Xu, L., Tan, C., et al. (2021). The effect of shionone on sepsis-induced acute lung injury by the ecm1/stat5/nf-κb pathway. Front. Pharmacol. 12, 764247. doi:10.3389/Fphar.2021.764247
Song, Y., Wu, Q., Jiang, H. J., Hu, A. H., Xu, L. Q., Tan, C. P., et al. (2022). The effect of shionone on sepsis-induced acute lung injury by the ECM1/STAT5/NF-κB pathway. Eff. Of Shionone Sepsis-Induced Acute Lung Inj. By Ecm1/Stat5/Nf-Κb Pathw. 12, 764247. doi:10.3389/fphar.2021.764247
Stonik, V. A., Kicha, A. A., Malyarenko, T. V., and Ivanchina, N. V. (2020). Asterosaponins: structures, taxonomic distribution, biogenesis and biological activities. Mar. Drugs 18, 584. doi:10.3390/md18120584
Su, X. D., Jang, H. J., Li, H. X., Kim, Y. H., and Yang, S. Y. (2019a). Identification of potential inflammatory inhibitors from aster tataricus. Bioorg Chem. 92, 103208. doi:10.1016/J.Bioorg.2019.103208
Su, X. D., Jang, H. J., Wang, C. Y., Lee, S. W., Rho, M. C., Kim, Y. H., et al. (2019b). Anti-inflammatory potential of saponins from aster tataricus via nf-κb/mapk activation. J. Nat. Prod. 82, 1139–1148. doi:10.1021/Acs.Jnatprod.8b00856
Su, X. D., Yang, S. Y., Shrestha, S. K., and Soh, Y. (2022). Aster saponin A(2) inhibits osteoclastogenesis through mitogen-activated protein kinase-C-Fos-Nfatc1 signaling pathway. J. Vet. Sci. 23, E47. doi:10.4142/Jvs.21246
Sun, Y., Li, L., Liao, M., Su, M., Wan, C., Zhang, L., et al. (2018). A systematic data acquisition and mining strategy for chemical profiling of aster tataricus rhizoma (ziwan) by uhplc-Q-tof-ms and the corresponding anti-depressive activity screening. J. Pharm. Biomed. Anal. 154, 216–226. doi:10.1016/J.Jpba.2018.03.022
Tanaka, R., Nagao, T., Okabe, H., and Yamauchi, T. (1990). Studies On The Constituents Of Aster Tataricus L. F. Iv.: Structures Of Aster Saponins Isolated From The Herb 38, 1153–1157.
Tang, X. W., Liu, X. X., Tang, Y. L., Liu, Y. L., Xu, K. H., and Med, J. J. T. C. V. (2006). Analysis of effective constituents from aster tataricus L. And extracting of alkaloid and its antibacterial test. Vitro 1, 16–18.
Tian, H., Xu, K. Y., Bi, C. R., Hang, J. S., and Piao, C. L. (2021). On Clinical Application And Dosage Of Tatarian Aster Root Jilin Journal Of Chinese Medicine 41, 99–102. doi:10.13463/J.Cnki.Jlzyy.2021.01.027
Tian, R. M., Meng, Y. J., Li, W. Y., and Ge, S. J. (2012). Evaluation and analysis on germplasm resources of aster tataricus L. F. J. Of Plant Genet. Resour. 13, 984–991. doi:10.13430/J.Cnki.Jpgr.2012.06.012
Tian, Y. P., Jing, X. J., Xu, L., Zhou, Y. N., and Zhang, L. T. (2008). Determination of ferulic acid, quercetin and kaempferol in aster tataricus by hplc. Chinese traditional and herbal drugs, 926–928.
Wan, C. C., Liu, Y. Y., Yang, H. T., Zhang, Q. Y., Liao, M., Zhang, X., et al. (2016). Simultaneous determination of nine constituents in asteris radix by hplc-ms/ms. Chin. Traditional And Herb. Drugs 47, 2534–2539.
Wang, F., Ren, G., Xiong, Y. A., Zhao, H. P., and Yang, M. (2015). Effect of shionone on il-1β,Tnf-Α and No release of macrophages induced by lipopolysaccharide. Chin. J. Of Exp. Traditional Med. Formulae 21, 123–125. doi:10.13422/J.Cnki.Syfjx.2015240123
Wang, G., Xie, W., Deng, L., Huang, X., Sun, M., Liu, W., et al. (2024). Nrf2 mediates the effects of shionone on silica-induced pulmonary fibrosis. Chin. Med. 19, 88. doi:10.1186/S13020-024-00947-5
Wang, G. Y., Wu, T., Lin, P. C., Chou, G. X., and Wang, Z. T. (2003). Phenolic compounds isolated from rhizoma of aster tataricus. China J. Of Chin. Materia Medica, 52–54.
Wang, J., Liu, Y., Li, R., Chen, H. M., and Li, P. (2023a). Effects of different processing methods on chemical constituents of ziwan. Pharm. And Clin. Of Chin. Materia Medica 14, 17–20+34.
Wang, J., Peng, S. M., Zhu, S. Q., Su, W. Y. Y., and Q, L. (2018). Ethnobotanical study on wild medicinal and edible plants in jinfo mountain region of chongqing. J. Of Plant Resour. And Environ. 27, 100–111.
Wang, L., Li, M. D., Cao, P. P., Zhang, C. F., Huang, F., Xu, X. H., et al. (2014a). Astin B, a cyclic pentapeptide from Aster tataricus, induces apoptosis and autophagy in human hepatic L-02 cells. Hum. Hepatic L-02 Cells 223, 1–9. doi:10.1016/j.cbi.2014.09.003
Wang, L., Li, M. D., Lu, Y., Zhang, C. F., Xu, X. H., and Zhang, M. (2014b). “Mechanism of apoptosis induced by peptide-rich fraction from the root of aster tataricus,” in Liver L-02 cells (Journal Of China Pharmaceutical University), 45, 469–474.
Wang, L., Zhang, M., Jin, J., Huang, F., and Zhang, C. F. (2010). Toxic fraction of radix asteris and its acute hepatotoxicity to mice. Lishizhen Med. And Materia Medica Res. 21, 2526–2528.
Wang, R., Li, F. R., Guo, W. N., and Shi, X. L. (2020a). “Simultaneous determination of chlorogenic acids in,” in Aster by uplc, 33. Journal Of Wenshan University, 19–22.
Wang, R., Xiao, S., and Niu, Z. Y. (2017). Anti-cancer activity Of Aster Tataricus on Scc-9 human oral squamous carcinoma. Anti-Cancer Activity Of Aster Tataricus Scc-9 Hum. Oral Squamous Carcinoma 14, 142–147. doi:10.21010/ajtcam.v14i2.14
Wang, R., Zhao, M. Y., Guo, W. N., Cheng, L., and Fang, C. W. (2020b). “Effects of different medicinal parts and processing methods on the content of medicinal ingredients,” in Aster, 33. Journal Of Wenshan University, 12–17.
Wang, X., Fan, L., Yin, H., Zhou, Y., Tang, X., Fei, X., et al. (2020c). Protective effect of aster tataricus extract on nlrp3-mediated pyroptosis of bladder urothelial cells. J. Cell Mol. Med. 24, 13336–13345. doi:10.1111/Jcmm.15952
Wang, X., Liu, Y., Li, R., Chen, H. M., and Li, P. (2023b). LncRNA BCRT1 depletion suppresses cervical cancer cell growth via sponging miR-432-5p/CCR7 axis. Tataricus. Pharmacy And Clinics Of Chinese Materia Medica 14, 17–20+34. doi:10.1007/s13205-023-03863-x
Wang, X., Yin, H., Fan, L., Zhou, Y., Tang, X., Fei, X., et al. (2021). Shionone alleviates Nlrp3 inflammasome mediated pyroptosis in interstitial cystitis injury. Int. Immunopharmacol. 90, 107132. doi:10.1016/J.Intimp.2020.107132
Wang, X. X., Xu, H. X., Wang, B., Wang, X., Li, Q., Meng, Y. L., et al. (2022). Effect of aster tataricus aqueous extract on pathological changes, apoptosis and expression tgf- Β1 in lung tissue of mice infected with Mycoplasma pneumoniae. Chin. J. Of Traditional Med. Sci. And Technol. 29, 975–977+1001.
Wang, Y. S. (1983). Pharmacology and application of Chinese herbs, China, People’s health publishing house.
Wątroba, M., Dudek, I., Skoda, M., Stangret, A., Rzodkiewicz, P., and Szukiewicz, D. J. A. R. R. (2017). Sirtuins, epigenetics and longevity. Epigenetics And Longev. 40, 11–19. doi:10.1016/j.arr.2017.08.001
Wei, P., Luo, Q., Hou, Y., Zhao, F., Li, F., Meng, Q., et al. (2023). Houttuynia cordata thunb.: a comprehensive review of traditional applications, phytochemistry, pharmacology and safety. 155195.
Wu, D., Lin, X., Lei, S., Jiang, F., and Tan, J. (2023). “Intervention effects of ziwan powder on airway inflammation,” in Chronic bronchitis based on tlr4/myd88/nf-κb signaling pathway, 43. Journal Of Hunan University Of Chinese Medicine, 1180–1187.
Wu, H., Chen, Y., Huang, B., Yu, Y., Zhao, S., Liu, J., et al. (2021). Aster tataricus alleviates constipation by antagonizing the binding of acetylcholine to muscarinic receptor and inhibiting Ca(2+) influx. Biomed. Pharmacother. 133, 111005. doi:10.1016/J.Biopha.2020.111005
Wu, M., Chen, W., Lu, Y., Zhu, G., Hao, L., and Li, Y. P. (2017). Gα13 negatively controls osteoclastogenesis through inhibition of the Akt-GSK3β-NFATc1 signalling pathway. Nat. Commun. 8, 13700. doi:10.1038/Ncomms13700
Wu, N., Huang, Y. X., Shi, X., Chen, J. L., Peng, L. F., Yang, L. L., et al. (2020). Effect of lysionotus pauciflorus maxim on signaling pathway of tlr4-myd88-nf-κb in lung tissue of rats with chronic bronchitis, 45. Journal Of Guizhou Medical University, 1283–1288. doi:10.19367/J.Cnki.2096-8388.2020.11.009
Wu, T., Chen, Z. J., Hu, Y. J., Xiu, Y. F., and Cheng, X. M. (2006). Experimental study on phlegm- resolving action of different prepared products of radix asteris. Acta Univ. Tradit. Medicalis Sin. Pharmacol., 55–57. doi:10.16306/J.1008-861x.2006.03.019
Wu, T., Wang, G. Y., Chou, G. X., Gu, L. H., Wang, Z. T., and Hu, Z. B. (2003). Determination of shionone in radix asteris by hplc. Zhongguo Zhong Yao Za Zhi 28, 738–740.
Xi, R. Y., Bai, S. P., Sun, X. D., and Dong, L. (2003). Determination of mineral elements and amino acids in aster tataricus L. F. Amino Acids &Biotic Resour., 8–9.
Xiu, Y. F., Cheng, X. M., Liu, L., Wu, T., and Wang, Z. T. (2006). Comparison of shionone content in different slices of prepared radix asteris. Acta Univ. Tradit. Medicalis Sin. Pharmacol. Shanghai, 59–61. doi:10.16306/J.1008-861x.2006.02.021
Xu, H. M., Yi, H., Zhou, W. B., He, W. J., Zeng, G. Z., Xu, W. Y., et al. (2013). Tataricins A and B, two novel cyclotetrapeptides from Aster tataricus, and their absolute configuration assignment. And Their Absol. Configuration Assign. 54, 1380–1383. doi:10.1016/j.tetlet.2012.12.111
Xu, M., Yang, Z., Zhang, W., and Zhang, X. (2024). Shionone improve tnbs-induced Crohn's disease like colitis by inhibiting apoptosis of intestinal epithelial cells. Journal Of Shanxi Medical University 55, 319–325. doi:10.13753/J.Issn.1007-6611.2024.03.007
Yang, B., Xiao, Y. Q., Liang, R. X., Wang, R. J., Li, W., Zhang, C., et al. (2008). Studies on expectorant compounds in volatile oil from root and rhizome of aster tataricus. Zhongguo Zhong Yao Za Zhi 33, 281–283.
Yang, D., Wang, S., Huang, X., Ma, W., Xue, Z., Zhao, L., et al. (2021). Pharmacokinetic comparison of 15 active compositions in rat plasma after oral administration of raw and honey-processed aster tataricus extracts. J. Sep. Sci. 44, 908–921. doi:10.1002/Jssc.202001020
Yang, H. H., Son, J.-K., Jung, B., Zheng, M., and Kim, J.-R. J. P. M. (2011). Epifriedelanol from the root bark of Ulmus davidiana inhibits cellular senescence in human primary cells. Hum. Prim. Cells 77, 441–449. doi:10.1055/s-0030-1250458
Yang, T. (2003). An excellent perennial flower–aster tataricus landscape architecture academic journal, 63.
Yang, X., Dai, L., Yan, F., Ma, Y., Guo, X., Jenis, J., et al. (2024). The phytochemistry and pharmacology of three rheum species: a comprehensive review with future perspectives. 155772.
Yang, Y. Y., Qin, T. T., Qian, S. S., Peng, L., Liu, L. J., Bai, S. J., et al. (2015). Effects of honey-fried radix asteris decoction(hfrad) on cell proliferation and cell cycle of colorectal cancer lovo cells. World Chin. Med. 10, 1755–1759.
Yao, J., Cheng, L., Zhu, Y. J., Ma, L., Cao, Z. Z., and Zhi, H. (2019). Research progress on evaluation index of seed and seedling standardization system of aster tataricus. Modern agricultural science and technology, 67–68+71.
Yao, P. B., Liu, Y. L., Zhu, Z. G., Zhao, H., and Zhang, J. X. (2022). Effects and aster water extract on proliferation and invasion of human lung cancer A549 Cells,And tumorigenesis ability of nude mice. J. Of Pharm. Analysis 42, 380–386. doi:10.16155/J.0254-1793.2022.03.03
Ye, J. (2007). Aster tataricus and semiaquilegia adoxoides. Master's degree, school of pharmaceutical science and technology (Tianjin University).Study on the constituents and their primary antitumor effect
Yin, D. F., Zhou, K., Liu, J. T., Hu, L., Liu, Y., Deng, J., et al. (2016). Development and validation of an lc/ms/ms method for simultaneous determination of shionone and epi-friedelinol in rat plasma for pharmacokinetic study after oral administration of aster tataricus extract. Biomed. Chromatogr. 30, 1112–1117. doi:10.1002/Bmc.3658
Yoshioka, H., Nakatsu, K., Sato, M., and Tatsuno, T. (1973). The molecular structure of cyclochlorotine,. A Toxic. Chlorine-Containing Cycl. Pentapeptide 2, 1319–1322. doi:10.1246/cl.1973.1319
Yu, L. H., and Liu, G. T. (2009). The structure-activity relationship of dibenzo(A.C)cyclooctene lignans isolated from fructus schizandrae and innovation of novel ant-I hepatitis drugs progress in. Chemistry 21, 66–76.
Yu, P., Cheng, S., Xiang, J., Yu, B., Zhang, M., Zhang, C., et al. (2015a). Expectorant, antitussive, anti-inflammatory activities and compositional analysis of aster tataricus. J. Ethnopharmacol. 164, 328–333. doi:10.1016/J.Jep.2015.02.036
Yu, P., Cheng, S., Xiang, J., Yu, B., Zhang, M., Zhang, C. F., et al. (2015b). Expectorant, antitussive, anti-inflammatory activities and compositional analysis of Aster tataricus. Anti-Inflammatory Activities And Compos. Analysis Of Aster Tataricus 164, 328–333. doi:10.1016/j.jep.2015.02.036
Yu, Z., Ma, G., and Liang, H. (2023). Herbal textual research on the traditional Chinese medicine aster tataricuse. J. Of Chin. Med. Mater. 46, 2346–2352. doi:10.13863/J.Issn1001-4454.2023.09.041
Zhang, G., Hao, E., Xiao, J., Yao, C., Wang, Y., and Luo, H. (2024). Abrus cantoniensis hance: ethnopharmacology, phytochemistry and pharmacology of A promising traditional Chinese medicine. J. Ethnopharmacol. 334, 118543. doi:10.1016/J.Jep.2024.118543
Zhang, J. L., Jin, X., and Wang, H. W. (2012a). Comparative analysis of volatile constituents in herbal pair flos farfarae-tatarian aster root and its single herb by gc-ms. Fine Chem. 29, 254–257. doi:10.13550/J.Jxhg.2012.03.005
Zhang, J. W., Dou, C. G., Zhang, M., Ma, S. P., and Huang, F. (2007). Toxicity of radix asteris flos farfarae and their combination. Chin. J. Of Clin. Pharmacol. And Ther., 405–411.
Zhang, L. Q., Zhang, Z. J., Wang, X. C., Yang, F., and Xue, C. S. (2022). Determination of shionone in roots. Stems And Flowers Of Aster By Hplc Ginseng Res. 34, 28–31. doi:10.19403/J.Cnki.1671-1521.2022.05.008
Zhang, T., and Zhou, X. (2014). Clinical application of expectorant therapy in chronic inflammatory airway diseases (review). Exp. Ther. Med. 7, 763–767. doi:10.3892/Etm.2014.1494
Zhang, X. L., Meng, Y. J., Jia, K. X., Bai, X. R., and Ge, S. J. (2021). Temporal and spatial variation of asterone content in aster tataricuse. Jiangsu Agric. Sci. 49, 174–179. doi:10.15889/J.Issn.1002-1302.2021.16.032
Zhang, Y., Wang, Q., Wang, T., Zhang, H., Tian, Y., Luo, H., et al. (2012b). Inhibition of human gastric carcinoma cell growth in vitro by A polysaccharide from aster tataricus. Int. J. Biol. Macromol. 51, 509–513. doi:10.1016/J.Ijbiomac.2012.06.019
Zhao, D. X., Hu, B. Q., Zhang, M., Zhang, C. F., and Xu, X. H. (2015). Simultaneous separation and determination of phenolic acids, pentapeptides, and triterpenoid saponins in the root of aster tataricus by high-performance liquid chromatography coupled with electrospray ionization quadrupole time-of-flight mass Spectrometry. J. Sep. Sci. 38, 571–575. doi:10.1002/Jssc.201401008
Zhou, W. B. (2010). Studies on chemical constituents from aster tataricus L.F. Master's degree. Hubei University Of Chinese Medicine.
Zhou, W. B., Tao, J. Y., Xu, H. M., Chen, K. L., Zeng, G. Z., Ji, C. J., et al. (2010). Three New Antivir. Triterpenes Aster Tataricus 65, 1393–1396.
Zhou, W. B., Zeng, G. Z., Xu, H. M., He, W. J., and Tan, N. H. (2013). Astataricusones A-D and astataricusol A, five new anti-hbv shionane-type triterpenes from aster tataricus L. F. Molecules 18, 14585–14596. doi:10.3390/Molecules181214585
Zhou, W. B., Zeng, G. Z., Xu, H. M., He, W. J., Zhang, Y. M., and Tan, N. H. (2014). Astershionones A-F, six new anti-hbv shionane-type triterpenes from aster tataricus. Fitoterapia 93, 98–104. doi:10.1016/J.Fitote.2013.12.021
Zou, C., Zhang, R. P., Zhao, B. T., Ao, X., Hao, X. J., and Zhou, J. (1999). A bioactive amide from roots of aster tartaricus acta botanica yunnanica, 123–126.
Keywords: Aster tataricus L.f., phytochemistry, ethnopharmacology, pharmacological activity, toxicology, comprehensive utilization
Citation: Zhang X, Liu M, Zhang X, Ma L, Song S, Pan M, Huang S, Ren W and Ma W (2025) Aster tataricus L. f.: a review on the botany, phytochemistry, ethnopharmacology, pharmacology, toxicology and comprehensive utilization. Front. Pharmacol. 16:1581505. doi: 10.3389/fphar.2025.1581505
Received: 22 February 2025; Accepted: 26 March 2025;
Published: 09 April 2025.
Edited by:
Irina Ielciu, University of Medicine and Pharmacy Iuliu Hatieganu, RomaniaReviewed by:
Patricia Quintero Rincón, University of Antioquia, ColombiaKwanchayanawish Machana, Nakhon Ratchasima College, Thailand
Ali Raza, University of Engineering and Technology, Taxila, Pakistan
Davinder Kumar, Pandit Bhagwat Dayal Sharma University of Health Sciences, India
Vibha Kumari, Rajiv Academy for Pharmacy, India
Copyright © 2025 Zhang, Liu, Zhang, Ma, Song, Pan, Huang, Ren and Ma. This is an open-access article distributed under the terms of the Creative Commons Attribution License (CC BY). The use, distribution or reproduction in other forums is permitted, provided the original author(s) and the copyright owner(s) are credited and that the original publication in this journal is cited, in accordance with accepted academic practice. No use, distribution or reproduction is permitted which does not comply with these terms.
*Correspondence: Weichao Ren, cmVud2VpY2hhb0BobGp1Y20uZWR1LmNu; Wei Ma, bWF3ZWlAaGxqdWNtLmVkdS5jbg==