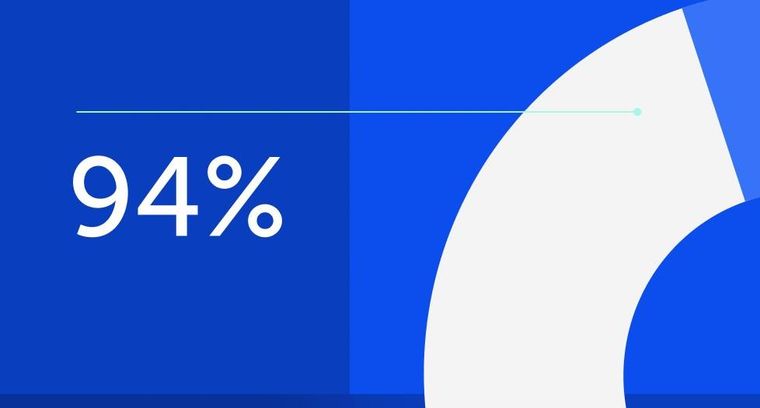
94% of researchers rate our articles as excellent or good
Learn more about the work of our research integrity team to safeguard the quality of each article we publish.
Find out more
REVIEW article
Front. Pharmacol., 16 April 2025
Sec. Experimental Pharmacology and Drug Discovery
Volume 16 - 2025 | https://doi.org/10.3389/fphar.2025.1580805
This article is part of the Research TopicInsights in Experimental Pharmacology and Drug Discovery: 2024View all 9 articles
Chemical overexposure is a significant concern in both environmental and occupational settings, often leading to oxidative stress and subsequent cellular damage. The review covers the oxidative stress induced by chemical overexposure to substances such as pesticides (including pyrethroid, deltamethrin, β-cyfluthrin, malathion, triflumuron, methomyl, diquat, cypermethrin, thiamethoxam, and profenofos), medications (acetaminophen), nanoparticles (including zinc oxide, iron, silver, and titanium dioxide), heavy metals (including cadmium, vanadium), and some organic chemicals (including diethylnitrosamine and benzo(a)pyrene). Focusing on preclinical evidence from animal models and cell-based studies, essential oils have been shown to significantly enhance antioxidative enzyme activities, including superoxide dismutase, catalase, and glutathione peroxidase, while also increasing levels of non-enzymatic antioxidants such as glutathione and uric acid. Additionally, essential oils contribute to the restoration of biochemical parameters, including creatinine, uric acid, and urea levels, while mitigating oxidative damage by reducing cell membrane injury, apoptosis, and histopathological alterations. Additionally, this review evaluates the protective benefits of essential oils against chemical overexposure in animal models. The underlying mechanism is involved in alleviating hepatorenal damage. This review underscores the considerable promise of essential oils as candidates for medicinal applications in functional foods or medicines, particularly in preventing oxidative stress induced by various chemical overexposure.
Overexposure to pesticides, medicines, environmental pollutants, UV radiation, cigarette smoke, and other various chemicals are significant external sources of reactive oxygen species (ROS) (Sule et al., 2022). When ROS production overwhelms the body’s antioxidant defenses, it leads to cellular and molecular damage, affecting lipids, proteins, and DNA, and potentially disrupting normal cellular functions (Tian et al., 2025), contributing to chronic diseases such as cancer, cardiovascular disease, neurodegenerative disorders, and metabolic syndromes (Tiwana et al., 2024). For instance, occupational exposure to benzene and polycyclic aromatic hydrocarbons has been associated with elevated risks of leukemia and respiratory diseases (Chiavarini et al., 2024). Additionally, high levels of heavy metals like cadmium and lead have been correlated with kidney dysfunction and cognitive decline (Howard et al., 2024; Yang et al., 2025). These findings underscore the urgent need for effective antioxidant strategies to mitigate oxidative damage induced by chemical exposure.
Essential oils (EOs), as secondary metabolites, belong to the group of biologically active volatile organic compounds (Ivanova et al., 2025). They have been widely used in various fields including medicine, agriculture, cosmetics, perfumes, and as food condiment (He et al., 2023; Agnihotry et al., 2024). EOs offer health benefits to prevent infectious and chronic diseases, thus improving digestion, appetite, as well as regulating gut microbiota (Aziz et al., 2024). There is an increasing interest in utilizing EOs due to their bioherbicide potential (Poveda et al., 2025), antibacterial efficacy (Khwaza and Aderibigbe, 2025), and insecticidal properties (Li et al., 2025). In the food industry, studies have found that adding EOs from medicinal and aromatic plants can introduce various bioactive compounds exhibiting a range of medicinal potentials such as antiviral (Misra et al., 2024), antifungal (Zhou et al., 2025), antibiofilm (Devi et al., 2024), antibacterial activities (Khwaza and Aderibigbe, 2025), and anti-inflammatory effect (Huang et al., 2025), which help reduce fat oxidation and improve both food shelf-life and aromatic flavor (Liñán-Atero et al., 2024). Furthermore, many commodities using the essential oils as raw material are widely employed in aromatherapy, where they could relieve some psychological and/or physical disorders (Murugesh et al., 2024), such as anxiety and depression (Chavda et al., 2025).
EOs derived from plants have shown considerable medicinal potential as antioxidants in various conditions that involve oxidative stress. Their diverse bioactive compounds exhibit strong antioxidant properties through multiple mechanisms, including free radical scavenging (Messaoudi et al., 2024), metal ion chelation (Mohammed et al., 2024), and modulation of endogenous antioxidant systems (Kashyap, 2024). Compared to synthetic antioxidants or single-compound natural antioxidants, EOs offer a complex mixture of phytochemicals that may exert synergistic effects. EOs have been widely used in traditional medicine and functional foods, making them promising candidates for mitigating oxidative stress-related damage induced by chemical overexposure. Additionally, EOs derived from plants are natural, environmentally friendly, and sustainable candidates, offering a renewable resource with potential applications in health and wellness.
The aim of this review is to summarize the antioxidant potential of essential oils and their potential therapeutic effects on cellular oxidative damage induced by chemical overexposure. Additionally, this review analyzes the antioxidant mechanisms reported in different studies, highlighting the multifaceted actions of essential oils, which involve various bioactive compounds that scavenge free radicals, chelate metal ions, activate endogenous antioxidant systems, and regulate inflammation and autophagy. This review highlights their potential applications in functional foods and medicinal formulations for preventing oxidative stress.
Overexposure to pesticides presents an ongoing health risk. It will lead to severe impairments to spleen, liver, kidney, and brain (Hussain et al., 2025). Overexposure could cause cognitive and neurological damage by modulating the permeability of voltage-gated sodium channels in nerve cells, causing repetitive neural impulses (Saravi and Dehpour, 2016; Erdman, 2016). In addition, pesticides exhibit more neurobiological affects, impacting cholinergic neurotransmission, dopaminergic, noradrenergic, and central γ-aminobutyric acid (GABA) (Huang et al., 2024; Menzikov et al., 2024). EOs extracted from plants have shown their antioxidative effects on tissues damage caused by pesticides overexposure (Table 1).
Table 1. The source of essential oils and their antioxidative effects on tissues damage caused by pesticides overexposure.
Origanum majorana essential oil demonstrated significant protection by normalizing marker enzymes, as well as replenishing of antioxidant levels, indicating the benefits in hepatic oxidative damage rats caused by pyrethroid (Mossa et al., 2013). Deltamethrin, an artificial pyrethroid possessing strong insecticidal properties has been shown to induce noteworthy elevated biochemical parameters including hepatic lipid peroxidation (LP), alanine aminotransferase (ALT), alkaline phosphatase (ALP), aspartate aminotransferase (AST), carbonyl protein, and advanced oxidized protein products (AOPP), along with a decreased glutathione (GSH), glutathione peroxidase (GPX), catalase (CAT) and SOD levels, which were validated by histological studies (Ncir et al., 2020). However, Allium sativum EO notably alleviated the hepatotoxicity in deltamethrin-treated rats (Ncir et al., 2020). Artemisia. campestris EO also showed its capability to normalize the modified acetylcholinesterase (AChE), acid, uric, urea and creatinine concentrations, and to reduce LP in deltamethrin-intoxicated rats (Saoudi et al., 2017).
Similarly, β-cyfluthrin, another pyrethroid insecticide, caused severe increase in LP parameters (H2O2 and thiobarbituric acid reactive substances (TBARS)), protein oxidation (hydroxyproline (HYP), AOPP, and protein carbonyl (PC)), proinflammatory gene expressions (interleukin (IL)-6, tumor necrosis factor-α (TNF-α)), cell cycle arrest in the G0/G1 phase of hepatocyte, a reduced cell number in G2/M and S phase, and DNA damage (Jebur et al., 2022). Ocimum basilicum essential oil could effectively abolish these adverse effects, restoring enzymatic (glutathione S-transferase (GST), glutathione reductase (GR), CAT, GPx, and superoxide dismutase (SOD)) and non-enzymatic antioxidants (such as GSH), as well as enzyme activities (lactate dehydrogenase (LDH), ALP, ALT, and AST), weights of liver and body, hematological markers, total bilirubin levels, globulin, and albumin (Jebur et al., 2022). This indicates that O. basilicum essential oil has a beneficial effect on liver protection.
Cypermethrin (CP) is extensively utilized in agriculture to protect crops against insects especially in cotton fields (Farhan et al., 2024). Essential oil from Origanum vulgare (OVEO) rich in phytochemicals like p-cymene, γ -terpinene, carvacrol, and thymol, possesses significant antioxidative capacity (Alagawany et al., 2020; Oniga et al., 2018). Dietary supplemented with OVEO resulted in a notable reduction in creatinine, urea, ALP, AST, and ALT levels (Khafaga et al., 2020). Apoptosis and histopathologic changes were notably diminished, accompanied by a simultaneous reduction of DNA damage in Cyprinus carpio overexposed to CP (Khafaga et al., 2020).
Besides, many research has found that EOs possess benefits in protecting against diverse insecticide overexposure. Lavandula stoechas essential oils (LSEO) has been shown to mitigate hepatic and renal oxidative stress induced by malathion including an increase in the levels of malondialdehyde (MDA) and H2O2, a reduction in antioxidants including total SOD, Cu/Zn-SOD, Mn-SOD, Fe-SOD, GPx, CAT, and sulfhydril (-SH) group level in the kidney and liver, suggesting potential nephro- and hepatoprotective effects in mice (Selmi et al., 2015).
Profenofos (PFF), one of organophosphate insecticides widely utilized to control various crop insects such as cotton, paddy, and tobacco (Majumder et al., 2024). Supplementation with Geranium essential oil (GEO) improved PFF-induced increases in ALT, AST, ALP, MDA, triglycerides (TG), cholesterol, urea, creatinine, and LDH levels (Rahman et al., 2020). It also mitigated the decline in antioxidative enzyme activities and GSH, NO, complement3 (C3), lysozyme activity, glucose (Glu), globulins, and total protein (TP) (Rahman et al., 2020). Consequently, GEO improved the histological structure in kidney and liver in PFF-exposed fish.
Pelargonium graveolens Essential Oil (EO) have been found the protective effect on the hippocampus of methomyl-intoxicated rats, including histopathological alterations, as well as mended memory impairments due to its potential antioxidant actions (Amine et al., 2020).
In aquaculture, EOs have also shown protective effects against various insecticide overexposure. Similarly, the concurrent addition of Thymus vulgaris essential oil to the diet of African catfish (Clarias garipenus) alleviated histomorphometric and histopathological damage due to thiamethoxam (TMX) toxication, one of neonicotinoid insecticides widely used for controlling cotton pests and various crop pests (El Euony et al., 2020). It modulated immunotoxicity, oxidative stress, and hepatorenal damage, and increased caspase-3 immunopositive splenocytes and proliferating cell nuclear antigen (PCNA) levels (El Euony et al., 2020).
Similarly, fennel essential oil (FEO) pretreatment for 2 h resulted in modulation in CAT and SOD activities, reduction in the mitochondrial membrane potential loss DNA damage, MDA levels, and ROS production, thus elevating cell viability in human carcinoma cells (HCT116) subjected to oxidative stress induced by triflumuron (TFM) (Timoumi et al., 2020).
In an experiment, Oregano (O. vulgare L.) EO could counteract oxidative damage in jejunum of diquat-intoxicated rats, thus preserving its structure (Wei et al., 2015). The underlying mechanism may be associated with modulating specific enteric microorganism, inhibiting inflammatory cytokines level, consequent elevating occludin content (Wei et al., 2015).
Thus, EOs are promising candidates due to their benefits performed in pesticide overexposure. They have shown excellent ameliorative effects on toxic impacts related to oxidative stress, immunological markers, and hepato-renal functions.
Although effective medications with therapeutic doses are commonly hold in households, administering them in an overdose can lead to grievous acute hepatic failure, cell death, hepatic injury, and even high mortality within both animal and human (Greydanus et al., 2023). Paracetamol (PCM), also known as acetaminophen (N-acetyl-p-aminophenol, APAP), is one of the most widely used analgesics. APAP overdose will lead to hepatic damage, leading to acute liver failure (Cardia et al., 2021; Dadkhah et al., 2015). Its toxic effect occurs when taken in a single or repeated high dose or after chronic ingestion (Dear, 2024). Herbal EOs have shown their antioxidative effects on tissues damage caused by overdoses medications (Table 2).
Table 2. Essential oils plant name and their antioxidative effects on tissues damage caused by overdoses medications and nanoparticles.
Studies on paracetamol-intoxicated rats have revealed significant decreases in total protein and albumin, along with marked hyperglycemia and elevation in serum transaminases, urea and creatinine (Khattab et al., 2020). These toxic effects were significantly ameliorated in groups receiving cardamom oil or silymarin (Khattab et al., 2020). Administration of cardamom essential oil significantly improved hepato-renal profiles, elevated total antioxidative activity, and protected livers and kidneys from histopathological alterations within PCM-intoxicated rats due to its potent antioxidant effect (Khattab et al., 2020). Additionally, essential oil from Salvia officinalis L. (sage) alleviated liver damages in Co-amoxiclav-intoxicated rat models by enhancing antioxidant enzymes levels, limiting LP and hence reducing cell membrane injuries (El-Hosseiny et al., 2016).
The protective role of Lavandula officinalis essential oil on APAP-intoxicated hepatic injury in mice demonstrated a decrease within gamma-glutamyl transferase (γ-GT), ALP, AST, and ALT contents, as well as reductions within pro-inflammatory cytokines, NO, and myeloperoxidase (MPO) contents (Cardia et al., 2021). Furthermore, pre-treatment with L. officinalis essential oil demonstrated notable antioxidative activity through reducing ROS, carbonylated proteins, and MDA contents, while enhancing oxidized GSH, CAT, and SOD contents in the liver (Cardia et al., 2021). This suggests that this essential oil improves hepatic function via suppressing inflammatory responses and oxidative stress (Cardia et al., 2021). In rats with APAP-induced liver damages, Hypericum scabrum essential oils reversed enhanced LP contents in the liver, along with the ferric reducing ability of plasma (FRAP), and restored depleted GSH levels, as confirmed by the histological examination of liver biopsies (Dadkhah et al., 2014). Similar to H. scabrum essential oils, Achillea wilhelmsii C. Koch (Asteraceae) EOs significantly mitigated increased contents of FRAP and LP, compensated SOD and GSH levels, as indicated by histological examination showing reversal of hepatic necrosis (Dadkhah et al., 2015). In another APAP-overdosed mice, pretreatment with Schisandra chinensis (Turcz.) Baill. (Magnoliaceae) (SC) EO reduced IL-6, TNF-a, ALT, and AST contents in serum, mended GSH depletion and MDA accumulation, and inhibited cytochrome P450 2E1 (CYP2E1) in the liver (Zhao et al., 2022). This mitigation of liver injury is linked to increased expression of nuclear factor erythroid 2 (NFE2)-related factor 2 (Nrf2), glutamate-cysteine ligase (GCL), and heme oxygenase-1 (HO-1), along with the activation of autophagy through upregulation of hepatic light chain 3 (LC3)-Ⅱ and decreased p62 (Zhao et al., 2022).
These findings propose that EOs mediate their hepatoprotective effect through activation of antioxidant defense and/or autophagy, suggesting their potential use in the treatment of medications overdoses.
In recent years, nanoparticles (NPs) have been utilized for delivering nucleic acids, polypeptides, vaccines, genes, proteins, and drugs because of the unique physicochemical characteristics (Souto et al., 2024; Tenchov et al., 2025; Yuan et al., 2025). In another hand, concerns regarding the toxicity and/or safety in nanoparticles are raising such as zinc oxide nanoparticles (ZnO-NPs), iron nanoparticles (Fe-NPs), silver nanoparticles (AgNPs) and titanium dioxide nanoparticles (TiO2NPs).
Studies have shown that ZnO-NPs may induce histological changes, ctyogenetic abnormalities, biochemical alterations, DNA damage, and oxidative stress (Hassan et al., 2021). Thyme essential oil (TEO) has been found to alleviate these disturbances, showing protective effects against the hazards of ZnO-NPs (Hassan et al., 2021).
Similarly, rats exhibited notable elevation in DNA damage, lipid profiles, oxidative stress indicators, biochemical markers, cytokines, and regulation in antioxidative enzymes expression in liver and kidney tissues induced by Fe-NPs (El-Nekeety et al., 2021). The applications of Fe-NPs combined with basil essential oil (BEO) was shown to alleviate these disturbances, with high doses normalizing most indicators and improving the histological architecture in kidney and liver tissues (El-Nekeety et al., 2021).
Administration with AgNPs might enhance a harmful effect on the environment and human health. AgNPs have been found to disrupt renal functions and histological architecture, increase NO, MDA, TNF-α and serum electrolytes levels, decrease the antioxidative enzymes, downregulate P53 and Bax, upregulate Bcl-2, thus inducing histomorphometric damage (Abdel-Wahhab et al., 2020). Chenopodium murale essential oil (CMEO) has also been shown to protect renal functions encountering silver nanoparticles in a dose-dependent manner (Abdel-Wahhab et al., 2020).
Recently, titanium dioxide nanoparticles (TiO2NPs) have been widely used in various industries. These nanoparticles can lead to cell apoptosis, DNA damage, inflammatory response, and oxidative stress (Li and Tang, 2024; Manzoor et al., 2024). Numerous studies have reported that the administration of TiO2NP causes significant disturbances in liver and kidney function, lipid profile, inflammatory cytokines, gene expressions, and antioxidant properties in the liver and kidney (Abdel-Wahhab et al., 2021; Sallam et al., 2022; Salman et al., 2021). Additionally, there is an increase in sperm abnormalities, DNA damage in hepatic cells, chromosomal aberrations in bone marrow and germ cells, and histomorphometric alteration in the testis, kidney, and liver (Abdel-Wahhab et al., 2021; Sallam et al., 2022; Salman et al., 2021). In contrast, thyme EO has been found to improve all these parameters (Abdel-Wahhab et al., 2021; Sallam et al., 2022). Moreover, the co-administration of TiO2NPs and cinnamon essential oil (CEO) has been shown to alleviate these disturbances, enhance antioxidant capacity, and protect against TiO2NPs-induced oxidative damage and genotoxicity in male mice (Salman et al., 2021).
Thus, caution is advised when utilizing synthesized metal nanoparticles in various applications, as they may induce hepatic and renal toxicity and oxidative damage. In this context, EOs appear to be promising and safe candidates for mitigating hepatotoxicity and nephrotoxicity, providing protection against the oxidative stress and health hazards associated with metal nanoparticles. This suggests that EOs can be effectively applied in both food and pharmaceutical industries.
Essential oils from plant exert their antioxidative effects on tissues damage caused by environmental contaminants (Table 3).
Table 3. Essential oils plant name and their antioxidative effects on tissues damage caused by environmental contaminants, and hepatoprotective.
Overexposure to chemicals in environment continues to be significant global public health issues. Cadmium (Cd) overexposure, an ecologically dangerous toxic metal, has increased in the biosphere from both anthropogenic and natural sources (Eka Putri et al., 2025; Mohammed et al., 2025). Previous studies have reported that Cd toxicity leads to irreversible renal tubule dysfunction, impairing the removal of toxic chemicals and drugs, which can result in acute or chronic kidney failure, and if untreated, may lead to death (Lang and Schiffl, 2024; Satarug, 2024). Thymus serrulatus essential oil (TSAEO) could significantly improve elevated LP, non-enzymatic antioxidants, as well as kidney function biomarkers (Ansari et al., 2021). It also downregulated the increased expression of inducible nitric oxide synthase (iNOS), nuclear factor kappa-light-chain-enhancer of activated B cells (NF-κB) p65, and Smad2 while ameliorating alteration in renal tissue of Cd-overexposure rat models (Ansari et al., 2021). Similarly, chromium hexavalent (CrVI)-intoxicated rats exhibited increased oxidative damage profiles (H2O2, TBARS), kidney function parameters (uric acid (UA), creatinine, urea), along with significant declined enzymatic antioxidants (GST, GPx, CAT, and SOD), total protein level, and GSH, as well as altered histology of kidneys (El-Demerdash et al., 2021). Treatment with Rosmarinus officinalis essential oil (REO) before or after CrVI exposure observably mended most of the biomarkers and improved kidney tissue architecture (El-Demerdash et al., 2021). In another experiment, administration of vanadium (NH4VO3) in rats enhanced LDH, ALP, AST, and ALT activities, as well as NO, triglyceride, bilirubin, and cholesterol contents, while reducing antioxidant enzyme activities in the liver (Koubaa et al., 2021). However, this disturbance was markedly restored by application of Salvia officinalis (SO) EO, which also mitigated histopathological alteration and heat shock protein (HSP)72/73 overexpression, suggesting a protective effect of EO from S. officinalis on oxidative stress in liver of vanadium-intoxicated rats (Koubaa et al., 2021).
Diethylnitrosamine (DENA) is a toxic organic compound and potent carcinogen present in air, water, and soil (Limbu and Dakshanamurthy, 2023). The EO of Elettaria cardamomum (cardamom) has been found to enhance antioxidative capacities (CAT, SOD, GPx, GR, GST) in DENA-intoxicated rats’ brains and kidneys (Elguindy et al., 2018). Additionally, administration of cardamom and geraniol, a primary component of cardamom essential oil, resulted in a reduction of oxidative stress markers such as LP and the activity of ornithine decarboxylase (ODC) in the brain and kidney (Elguindy et al., 2018). It also increased GSH level of brain and kidney, and decreased AChE capacity of brain (Elguindy et al., 2018). Benzo(a)pyrene (BaP), a well-known environmental pollutant, is typically produced from organic materials’ incomplete combustion such as notably cigarette smoke, fossil fuel, and automobile exhaust (Ezugwu et al., 2024). BaP could be metabolized within cells, and its metabolites may contribute to carcinogenic processes (Hu et al., 2025). The administration of lemongrass (Cymbopogon citratus, Stapf) essential oil (LEO) to human embryonic lung fibroblast (HELF) cells exposed to BaP has been shown to enhance SOD and CAT activities and reduce MDA levels (Jiang et al., 2017). Furthermore, this EO can mitigate DNA damages and reductions in cell viability, as indicated by decreased 8-hydroxy-deoxyguanosine (8-OHdG) content (Jiang et al., 2017).
Particulate matter micrometers or less in diameter increases MDA, NF-κB, nicotinamide adenine dinucleotide phosphate (NADPH) oxidase 2 (Nox2) and ROS levels, whereas reducing levels of SOD and HO-1, resulting in brain’s oxidative damage (Amin et al., 2025; Kim et al., 2020). Mentha piperita essential oil could inhibit asthma subjected to PM10 by regulating the IL-6/JAK2/STAT3 signaling pathway (Kim et al., 2020). Compound essential oils (CEOs) showed protective benefits of alleviating PM2.5-induced autophagy and oxidative damage through inhibiting the 5′-adenosine monophosphate-activated protein kinase (AMPK)/mammalian target of rapamycin (mTOR) signaling pathway, offering a promising therapy in PM2.5-induced brain and lung damage (Ren et al., 2021).
The above results reveal that EOs have beneficial effects in ameliorating injuries in the brain, liver, kidney, lung, and bone marrow induced by toxic metals, organic compounds, and other environmental pollutants. This is attributed to their ability to modulate detoxification enzymes, enhance anti-oxidant stress capacities, and free radical scavenging. These results offer a novel opportunity for the prevention or treatment in environmental contaminant-induced damage.
Various animal models are employed to observe protective benefits of essential oils for treating hepatic injury, aiming to identify potential hepatoprotective agents. Carbon tetrachloride (CCl4), commonly utilized for inducing experimental hepatic damage in laboratory, is a known environmental pollutant (Fareed et al., 2024). Essential oils from Heracleum persicum have been shown to modulate oxidative stress/antioxidant disturbance, reduce hepatic lipid peroxides, and regulate levels of GSH and GST concomitant, alongside adapting plasma FRAP, AST and ALT levels in CCl4-treated rat models (Roshanaei et al., 2017). Cymbopogon citratus essential oil (CCEO) has also demonstrated protective effects against hepatic and renal damage and genotoxicity, reversing the increase in oxidative damage parameters (MDA), creatinine, blood urea, and hepatic enzyme biomarkers (γ-GT, ALP, AST, ALT) in rats induced by CCl4 (Fahmy et al., 2020).
In other models, EO from Pimpinella diversifolia has been shown its hepatoprotective effect in acute hepatic damage mouse models using lipopolysaccharide (LPS) and D-galactosamine (D-GalN) (Hua et al., 2023). The findings revealed that administration of PDREO dramatically decreased serum ALT and AST levels, and effectively alleviated hemorrhage, edema, necrosis, and apoptosis in hepatic cells (Hua et al., 2023). This effect might result from the notable anti-inflammatory actions (limiting monocyte-derived neutrophils and macrophages infiltration, and reducing inflammatory chemokines and cytokines contents) and its regulation in mending oxidative damage (boosting antioxidative enzyme expressions including CAT, SOD1, GPX4) (Hua et al., 2023). Additionally, the EO at 150 mg/kg could entirely prevent LPS/D-GalN-induced mortality in mice (Hua et al., 2023). Administration of EO from garlic and diallyl disulfide (DADS) notably limited pro-inflammatory cytokine secretions in the liver, along with enhanced antioxidative ability that is inhibiting cytochrome P450 2E1 formation in obesity mice with nonalcoholic fatty liver disease (NAFLD) induced by long-term high-fat diet (HFD) (Lai et al., 2014). The actions might be mediated through downregulating 3-hydroxy-3-methylglutaryl-coenzyme A reductase, acetyl-CoA carboxylase, fatty acid synthase, and sterol regulatory element-binding protein-1c, accompanied by stimulating carnitine palmitoyltransferase-1and peroxisome proliferator-activated receptor α (Lai et al., 2014).
Ongoing external chemical substances exposed eventually results in numerous diseases. Xenobiotic toxicity primarily affects the liver (Rovira et al., 2024). Due to its capacity for concentrating xenobiotics and the predominant function in metabolism, the liver is more susceptible to damage from these chemical substances than any other organs (Rovira et al., 2024). EO components are rapidly absorbed through oral, pulmonary, or dermal routes (Al-Harrasi et al., 2022). After absorption, they are either metabolized or distributed into adipose tissues and organs (Sadgrove et al., 2021). At low doses, plasma levels return to baseline within 1–3 h (Sadgrove et al., 2021). However, at higher doses, plasma concentrations can be sustained for several days due to buffering from body tissues (Sadgrove et al., 2021). The distribution hierarchy is typically adipose tissue > liver > kidneys > cerebrospinal fluids and brain (Sadgrove et al., 2021). The solubility of EO constituents significantly influences their absorption in the gastrointestinal tract, where they may interact with digested food, potentially reducing solubilization and absorption (Horky et al., 2019). Once absorbed, most EOs undergo extensive metabolism, primarily in the liver (Dhifi et al., 2016). Some EOs, such as terpenes and phenolic compounds, can interact with hepatic cytochrome P450 enzymes, potentially affecting drug metabolism by either inhibiting or inducing enzymatic activity (Zehetner et al., 2019). This modulation can influence the metabolism and detoxification of xenobiotics, thereby affecting their bioactivation or clearance. A substantial portion of EO compounds is excreted via the kidneys, as indicated by increased urinary metabolites (Horky et al., 2019). EO compounds are generally characterized by rapid metabolism and short half-lives, minimizing the risk of accumulation in body tissues (Al-Harrasi et al., 2022).
While current research demonstrates the antioxidant potential and the underlying mechanism of EOs (Figure 1), many mechanisms remain unknown. Generally, EOs, once absorbed, undergo extensive metabolism in the liver, where they can modulate oxidative stress, influencing cellular survival and tissue integrity (Dhifi et al., 2016). One key mechanism involves the regulation of autophagy. Certain EOs activate autophagy via p53 signaling, leading to the upregulation of hepatic light chain 3 (LC3)-Ⅱ, which facilitates the clearance of necrotic cells (Zhao et al., 2022). Conversely, other EOs inhibit autophagy through the AMPK/mTOR pathway and p62, promoting liver cell survival under stress conditions (Zhao et al., 2022). EOs also exert antioxidant effects by enhancing the expression of anti-oxidative genes such as heme oxygenase-1 (HO-1). This occurs through the activation of the Keap1/Nrf2 or Smad2/NF-κB p65 pathways, which subsequently reduce oxidative stress and inhibit inflammatory responses (Hua et al., 2023; Zhao et al., 2022). Additionally, mitochondrial protection is achieved by the suppression of P53 and Bax, the upregulation of Bcl-2, preventing mitochondrial dysfunction and associated cellular damage (Huang et al., 2025). Moreover, some EOs have been shown to reduce chemical overexposure-induced fibrosis, thereby mitigating morphological damage in the liver (Fareed et al., 2024). Through the combined effects of the above factors, DNA damage, lipid peroxidation, and protein damage are reduced, ultimately alleviating cellular damage. This reduction in cellular damage helps minimize tissue injury and preserve both the function and structure of the tissue.
Figure 1. The underlying protective mechanism of EOs against oxidative damage induced by chemical overexposure. (1) Mitochondrial Protection: EOs suppress the expression of P53 and Bax, and upregulate Bcl-2 expression, thereby preventing mitochondrial dysfunction and reducing cellular damage. (2) Autophagy Modulation: Certain EOs activate autophagy via the p53 signaling pathway, leading to the upregulation of hepatic LC3-Ⅱ, which facilitates the clearance of necrotic cells. Conversely, other EOs inhibit autophagy through the AMPK/mTOR pathway and p62, promoting liver cell survival under stress conditions. (3) Antioxidant gene transcription: EOs enhance the expression of anti-oxidative genes such as HO-1 through activation of the Keap1/Nrf2 or Smad2/NF-κB p65 pathways, thereby leading to increase in SOD, CAT, GPX, GR, GST, GSH, GCL and decrease in MDA, LP, FRAP, ALT, ALP, AST, AOPP, HYP, PC, γ-GT, and MPO. (4) Anti-inflammatory Effects: EOs modulate signaling pathways to suppress the expression of IL-6 and TNF-α, thereby inhibiting inflammation. (5) Alleviating cellular damage: through the combined effects of the above factors, DNA damage, lipid peroxidation, and protein damage are reduced, ultimately alleviating cellular damage. (6) Alleviating tissue damage: All the mechanisms work together to mitigate tissue injury and maintain both its structural integrity and functional capacity. ALP, alkaline phosphatase; ALT, alanine aminotransferase; AOPP, advanced oxidized protein products; AST, aspartate aminotransferase; CAT, catalase; CYP2E1, cytochrome P450 2E1; FRAP, ferric reducing ability of plasma; GCL, glutamate-cysteine ligase; GPX, glutathione peroxidase; GR, glutathione reductase; GST, glutathione S-transferases; GSH, glutathione; HO-1, heme oxygenase-1; HYP, hydroxyproline; IL, interleukin; LC3, light chain 3; LP, lipid peroxidation; MAPK, mitogen-Activated Protein Kinase; MDA, malondialdehyde; MPO, myeloperoxidase; mTOR, mammalian target of rapamycin; NF-κB, nuclear factor kappa-light-chain-enhancer of activated B cells; Nox2, nicotinamide adenine dinucleotide phosphate (NADPH) oxidase 2; NOS, nitric oxide synthase; Nrf2, nuclear factor erythroid 2 (NFE2)-related factor 2; PC, protein carbonyl; ROS, reactive oxygen species; SOD, superoxide dismutase; TNF, tumor necrosis factor; γ-GT, gamma-glutamyl transferase.
An analysis of the structure-function relationship of these EOs may help to reveal how their distinct structural features contribute to their diverse biological activities. For example, the main compositions of EOs from plants (Table 4) that have shown potential in alleviating pesticide-induced hepatorenal damage include a variety of bioactive compounds. Notable constituents are γ-terpinene, α-terpinene, and terpinen-4-ol, which have potent antioxidant and anti-inflammatory properties (Kakouri et al., 2022). Sulfur compounds like diallyl trisulfide and diallyl disulfides provide protective effects, particularly against oxidative stress (Herrera-Calderon et al., 2021). Monoterpenes such as linalool, 1,8-cineole, and thymol offer anti-inflammatory and antimicrobial benefits, while α-thujone and β-sesquiphellandrene have notable hepatoprotective activity (Abou El-Soud et al., 2015; Fayoumi et al., 2022; Lombrea et al., 2020; Pokajewicz et al., 2021; Rehman et al., 2022). Other compounds like citronellol, geraniol, and trans-anethole are known for their detoxifying and antioxidant effects, collectively contributing to the mitigation of pesticide-induced hepatorenal damage (Galovičová et al., 2021; Kačániová et al., 2023; Sharopov et al., 2017). The structures of these main compositions are showed in Figure 2.
Table 4. The main compositions and contents of essential oils from plants that have shown potential in alleviating pesticide-induced hepatorenal damage.
Figure 2. The structures of the main compositions which demonstrate the antioxidant potential in response to chemical overexposure.
At optimal doses, EOs can exert maximal therapeutic benefits (Table 1–3). While most EOs exhibit dose-dependent effects, where their antioxidant and protective properties vary with concentration. Some research reports conflicting findings regarding their efficacy and safety. There is still a need for well-designed in vivo studies and human clinical trials to validate the efficacy and safety of EOs in preventing chemical-induced oxidative stress. Some essential oils, such as eucalyptus and camphor, have been reported to exhibit toxicity at high doses, causing adverse effects like neurotoxicity and hepatotoxicity (Lemmens-Gruber, 2020; Soni et al., 2023). One study focusing on acute, developmental, and reproductive toxicity using alternative in vitro and in vivo models suggested that certain EOs, including rosemary, citrus, and eucalyptus oils could exhibit dose-dependent toxicity, oxidative stress induction, and mucous membrane irritation (Lanzerstorfer et al., 2021). Nonetheless, studies have suggested that the toxicity concerns associated with EOs could be leveraged for their development as plant-based pesticides (Nonci et al., 2025). Therefore, while EOs show promise as natural detoxification agents by protecting the liver against oxidative stress, inflammation, and lipid metabolic disorders, careful consideration of their dosage is essential to maximize benefits while minimizing adverse effects.
There is limited research specifically on combining EOs with pharmaceutical antioxidants. However, the observed synergistic interactions among various natural compounds suggest potential benefits in such combinations. For example, mixtures containing oregano and thyme oils, as well as cranberry and rosemary extracts, have shown synergistic antioxidant effects, effectively extending the shelf life of meat products and improving sensory acceptance (Khodaei et al., 2023; Latoch et al., 2023). Given the promising results of natural compound combinations, further research is warranted to explore the synergistic potential of EOs with pharmaceutical antioxidants.
Clinical trials should assess the bioavailability, dosage, and long-term effects of EOs in humans, particularly in populations at high risk of chemical exposure. As chemical exposures continue to diversify with emerging industrial processes and pollutants, future research should expand the range of chemicals studied in relation to EO protective benefits. Investigating the efficacy of EOs against newer pollutants, such as microplastics, endocrine disruptors, and novel nanomaterials, will be essential for staying ahead of evolving environmental and occupational risks. Additionally, considering the inherent instability of EOs under environmental stresses such as temperature and light, the exploration of novel technologies to safeguard and enhance their characteristics and biological activities becomes imperative. To improve the bioavailability and stability of EO components, strategies such as microencapsulation have been explored (Ambrosio et al., 2020). This approach enhances oxidative stability, thermostability, photostability, shelf life, biological activity, and ensures targeted delivery of EOs. Finally, the sustainable production and harvesting of plants for essential oil extraction must be addressed. Establishing standardized protocols for EO composition and quality control is vital to ensure consistent therapeutic outcomes and minimize environmental impacts.
This review highlights the promising role of EOs as protective agents against oxidative stress induced by chemical overexposure. The evidence from studies on various chemicals-including pesticides, medications, nanoparticles, heavy metals, and organic compounds-demonstrates that EOs can mitigate oxidative damage through their antioxidant properties. Among them, EOs from Origanum species have shown notable efficacy in mitigating oxidative damage of pesticide overexposure. Lavandula stoechas EOs have exhibited protective properties against both pesticide-induced oxidative damage and overdoses of medications. Similarly, Salvia officinalis L. EOs have been identified for their potential to counteract the harmful effects of medication overdoses and organic chemical exposure. Furthermore, Thymus vulgaris L. EOs have demonstrated beneficial effects in mitigating oxidative damage resulting from medication overdoses and heavy metal exposure. The application of EOs in functional foods and pharmaceuticals presents significant potential for preventive and therapeutic use. However, further research is necessary to deepen our understanding of their mechanisms, optimize delivery systems, and confirm their efficacy in clinical settings. As chemical exposures continue to diversify in both environmental and occupational contexts, EOs represent a valuable avenue for future development in safeguarding human health. In conclusion, essential oils stand out as viable candidates for combating oxidative stress from chemical overexposure, paving the way for their integration into health-promoting interventions in both food and medicine.
FJ: Conceptualization, Funding acquisition, Project administration, Supervision, Writing – original draft, Writing – review and editing. XH: Conceptualization, Funding acquisition, Methodology, Visualization, Writing – original draft, Writing – review and editing. XC: Formal Analysis, Investigation, Methodology, Writing – review and editing. YX: Methodology, Resources, Visualization, Writing – review and editing.
The author(s) declare that financial support was received for the research and/or publication of this article. This work was supported by Yibin Vocational and Technical College, Initial funding for doctor (ybzysc23bk01), Natural Science Funds of Yibin Vocational and Technical College (ZRYB24-05), Professional Committee for the Integration of Industry and Education in Chinese Society for Educational Development Strategy (CJRHZWH2024-87).
The authors thank Yibin Sub-Center of Key Laboratory of Sichuan Province for Bamboo Pests Control and Resource Development for support local collaboration.
The authors declare that the research was conducted in the absence of any commercial or financial relationships that could be construed as a potential conflict of interest.
The author(s) declare that no Generative AI was used in the creation of this manuscript.
All claims expressed in this article are solely those of the authors and do not necessarily represent those of their affiliated organizations, or those of the publisher, the editors and the reviewers. Any product that may be evaluated in this article, or claim that may be made by its manufacturer, is not guaranteed or endorsed by the publisher.
Abdel-Wahhab, M. A., Ahmed, H., El-Nekeety, A. A., Abdel-Aziem, S. H., Shara, H. A., Abdelaziz, M., et al. (2020). Chenopodium murale essential oil alleviates the genotoxicity and oxidative stress of silver nanoparticles in the rat kidney. Egypt J. Chem. 63 (7), 0–2646. doi:10.21608/EJCHEM.2019.18341.2128
Abdel-Wahhab, M. A., El-Nekeety, A. A., Mohammed, H. E., Elshafey, O. I., Abdel-Aziem, S. H., and Hassan, N. S. (2021). Elimination of oxidative stress and genotoxicity of biosynthesized titanium dioxide nanoparticles in rats via supplementation with whey protein-coated thyme essential oil. Environ. Sci. Pollut. Res. 28, 57640–57656. doi:10.1007/s11356-021-14723-7
Abou El-Soud, N. H., Deabes, M., Abou El-Kassem, L., and Khalil, M. (2015). Chemical composition and antifungal activity of Ocimum basilicum L. essential oil. Open Access Maced. J. Med. Sci. 3 (3), 374–379. doi:10.3889/oamjms.2015.082
Agnihotry, S., Chopra, D., Singh, J., Negi, S., Srivastav, A. K., Upadhyay, J., et al. (2024). Aromatherapy evolution and blending basics of essential oils. Aromather. Sci. Essent. Oils 1, 1–30. doi:10.2174/9789815136203124010005
Alagawany, M., Abd El-Hack, M. E., Farag, M. R., Shaheen, H. M., Abdel-Latif, M. A., Noreldin, A. E., et al. (2020). The applications of and its derivatives in human, ruminant and fish nutrition–a review. Ann. Anim. Sci. 20 (2), 389–407. doi:10.2478/aoas-2020-0004
Al-Harrasi, A., Bhatia, S., Ahmed, M. M., Anwer, K., and Sharma, P. B. (2022). “Pharmacokinetics of essentials oils,” in Role of essential oils in the management of COVID-19 (Boca Raton, FL: CRC Press), 359–370.
Ambrosio, C. M., Alvim, I. D., Contreras Castillo, C. J., and Da Gloria, E. M. (2020). Microencapsulation enhances the in vitro antibacterial activity of a citrus essential oil. J. Essent. Oil Bear. Plants 23 (5), 985–997. doi:10.1080/0972060X.2020.1833763
Amin, M., Ramadhani, A. A. T., Putri, R. M., Auliani, R., Torabi, S. E., Hanami, Z. A., et al. (2025). A review of particulate matter (PM) in Indonesia: trends, health impact, challenges, and options. Environ. Monit. Assess. 197 (1), 11–19. doi:10.1007/s10661-024-13426-z
Amine, K. M. H., Kahina, C., Nawel, H., Faiza, Z., Jean, G., Mohamed, T., et al. (2020). Protective effects of Pelargonium graveolens essential oil on methomyl-induced oxidative stress and spatial working memory impairment in association with histopathological changes in the Hippocampus of male wistar rats. Basic Clin. Neurosci. 11 (4), 433–446. doi:10.32598/bcn.11.4.1402.1
Ansari, M. N., Rehman, N. U., Karim, A., Imam, F., and Hamad, A. M. (2021). Protective effect of Thymus serrulatus essential oil on cadmium-induced nephrotoxicity in rats, through suppression of oxidative stress and downregulation of NF-κB, iNOS, and Smad2 mRNA expression. Molecules 26 (5), 1252. doi:10.3390/molecules26051252
Aziz, T., Hussain, N., Hameed, Z., and Lin, L. (2024). Elucidating the role of diet in maintaining gut health to reduce the risk of obesity, cardiovascular and other age-related inflammatory diseases: recent challenges and future recommendations. Gut Microbes 16 (1), 2297864. doi:10.1080/19490976.2023.2297864
Cardia, G. F. E., de Souza Silva-Comar, F. M., Silva, E. L., da Rocha, E. M. T., Comar, J. F., Silva-Filho, S. E., et al. (2021). Lavender (Lavandula officinalis) essential oil prevents acetaminophen-induced hepatotoxicity by decreasing oxidative stress and inflammatory response. Res. Soc. Dev. 10 (3), e43410313461. doi:10.33448/rsd-v10i3.13461
Chavda, V., Balar, P., and Apostolopoulos, V. (2025). A review on essential oils: a potential tonic for mental wellbeing in the aging population? Maturitas 192, 108158. doi:10.1016/j.maturitas.2024.108158
Chiavarini, M., Rosignoli, P., Sorbara, B., Giacchetta, I., and Fabiani, R. (2024). Benzene exposure and lung cancer risk: a systematic review and meta-analysis of human studies. Int. J. Environ. Res. public health 21 (2), 205. doi:10.3390/ijerph21020205
Dadkhah, A., Fatemi, F., Alipour, M., Ghaderi, Z., Zolfaghari, F., and Razdan, F. (2015). Protective effects of Iranian Achillea wilhelmsii essential oil on acetaminophen-induced oxidative stress in rat liver. Pharm. Biol. 53 (2), 220–227. doi:10.3109/13880209.2014.913298
Dadkhah, A., Fatemi, F., Farsani, M. E., Roshanaei, K., Alipour, M., and Aligolzadeh, H. (2014). Hepatoprotective effects of Iranian Hypericum scabrum essential oils against oxidative stress induced by acetaminophen in rats. Braz Arch. Biol. Technol. 57, 340–348. doi:10.1590/S1516-89132014005000012
Devi, L. S., Das, B., Dutta, D., and Kumar, S. (2024). Essential oils as functional agents in biopolymer-based sustainable food packaging system: a review. Sustain Chem. Pharm. 39, 101563. doi:10.1016/j.scp.2024.101563
Dhifi, W., Bellili, S., Jazi, S., Bahloul, N., and Mnif, W. (2016). Essential oils’ chemical characterization and investigation of some biological activities: a critical review. Medicines 3 (4), 25. doi:10.3390/medicines3040025
Eka Putri, W. A., Meiyerani, J., Ulqodry, T. Z., Aryawati, R., Purwiyanto, A., Rozirwan, R., et al. (2025). Ecological risk assessment through heavy metal concentrations of lead, copper, cadmium, zinc, and nickel in marine sediments of Maspari Island, South Sumatra Indonesia. J. Ecol. Eng. 26 (2), 297–310. doi:10.12911/22998993/197413
El-Demerdash, F. M., El-Sayed, R. A., and Abdel-Daim, M. M. (2021). Rosmarinus officinalis essential oil modulates renal toxicity and oxidative stress induced by potassium dichromate in rats. J. Trace Elem. Med. Biol. 67, 126791. doi:10.1016/j.jtemb.2021.126791
El Euony, O. I., Elblehi, S. S., Abdel-Latif, H. M., Abdel-Daim, M. M., and El-Sayed, Y. S. (2020). Modulatory role of dietary Thymus vulgaris essential oil and Bacillus subtilis against thiamethoxam-induced hepatorenal damage, oxidative stress, and immunotoxicity in African catfish (Clarias garipenus). Environ. Sci. Pollut. Res. 27, 23108–23128. doi:10.1007/s11356-020-08588-5
Elguindy, N. M., Yacout, G. A., and El Azab, E. F. (2018). Amelioration of DENA-induced oxidative stress in rat kidney and brain by the essential oil of Elettaria cardamomum. Beni-Suef Univ. J. Basic Appl. Sci. 7 (3), 299–305. doi:10.1016/j.bjbas.2018.02.005
El-Hosseiny, L. S., Alqurashy, N. N., and Sheweita, S. (2016). Oxidative stress alleviation by sage essential oil in co-amoxiclav induced hepatotoxicity in rats. Int. J. Biomed. Sci. 12 (2), 71–78. doi:10.59566/ijbs.2016.12071
El-Nekeety, A. A., Hassan, M. E., Hassan, R. R., Elshafey, O. I., Hamza, Z. K., Abdel-Aziem, S. H., et al. (2021). Nanoencapsulation of basil essential oil alleviates the oxidative stress, genotoxicity and DNA damage in rats exposed to biosynthesized iron nanoparticles. Heliyon 7 (7), e07537. doi:10.1016/j.heliyon.2021.e07537
Erdman, N. R. (2016). Creation of a pioneer-neuron axonal pathfinding model for future use in developmental neurotoxicity testing applications. Doctoral dissertation, Clemson, SC: Clemson University.
Ezugwu, A. L., Agbasi, J. C., Egbueri, J. C., Abugu, H. O., Aralu, C. C., Ucheana, I. A., et al. (2024). Mechanism, formation and transport of polycyclic aromatic hydrocarbons (PAHs) in fruits, vegetables and fresh fish species in africa: a systematic review of its health risk. Chem. Afr. 7, 2321–2344. doi:10.1007/s42250-024-00926-1
Fahmy, M. A., Aly, F. A. E., Hassan, E. M., Farghaly, A. A., Hassan, E. E., and Sâmea, N. S. A. (2020). Cymbopogon citratus essential oil alleviates the genotoxicity and oxidative stress of carbon tetrachloride in mice. Comun. Sci. 11, 1–8. doi:10.14295/cs.v11i0.3219
Fareed, M. M., Khalid, H., Khalid, S., and Shityakov, S. (2024). Deciphering molecular mechanisms of carbon tetrachloride- induced hepatotoxicity: a brief systematic review. Curr. Mol. Med. 24, 1124–1134. doi:10.2174/0115665240257603230919103539
Farhan, M., Zhao, C., Akhtar, S., Ahmad, I., Jilong, P., and Zhang, S. (2024). Assessment of nano-formulated conventional insecticide-treated sugar baits on mosquito control and the effect on non-target aphidophagous Coccinella septempunctata. Insects 15 (1), 70. doi:10.3390/insects15010070
Fayoumi, L., Khalil, M., Ghareeb, D., Chokr, A., Bouaziz, M., and El-Dakdouki, M. H. (2022). Phytochemical constituents and therapeutic effects of the essential oil of rose geranium (Pelargonium hybrid) cultivated in Lebanon. South Afr. J. Bot. 147, 894–902. doi:10.1016/j.sajb.2022.03.039
Galovičová, L., Borotová, P., Valková, V., Vukovic, N. L., Vukic, M., Štefániková, J., et al. (2021). Thymus vulgaris essential oil and its biological activity. Plants 10 (9), 1959. doi:10.3390/plants10091959
Greydanus, D. E., Kukreti, P., and Pemde, H. K. (2023). Substance use and abuse in adolescents: an overview. N. T. J. Child. Adolesc. Health 16 (1), 21–51.
Hassan, M. E., Hassan, R. R., Diab, K. A., El-Nekeety, A. A., Hassan, N. S., and Abdel-Wahhab, M. A. (2021). Nanoencapsulation of thyme essential oil: a new avenue to enhance its protective role against oxidative stress and cytotoxicity of zinc oxide nanoparticles in rats. Environ. Sci. Pollut. Res. 28 (37), 52046–52063. doi:10.1007/s11356-021-14427-y
He, R., Wu, H., Liu, J., Chen, W., Chen, W., Chen, H., et al. (2023). Mechanism of membrane damage to Shigella sonnei by linalool from plant essential oils: a driver of oxidative stress. Ind. Crops Prod. 193, 116255. doi:10.1016/j.indcrop.2023.116255
Herrera-Calderon, O., Chacaltana-Ramos, L. J., Huayanca-Gutiérrez, I. C., Algarni, M. A., Alqarni, M., and Batiha, G. E. S. (2021). Chemical constituents, in vitro antioxidant activity and in silico study on NADPH oxidase of Allium sativum L.(garlic) essential oil. Antioxidants 10 (11), 1844. doi:10.3390/antiox10111844
Horky, P., Skalickova, S., Smerkova, K., and Skladanka, J. (2019). Essential oils as a feed additives: pharmacokinetics and potential toxicity in monogastric animals. Animals 9 (6), 352. doi:10.3390/ani9060352
Howard, J. A., David, L., Lux, F., and Tillement, O. (2024). Low-level, chronic ingestion of lead and cadmium: the unspoken danger for at-risk populations. J. Hazard. Mater. 478, 135361. doi:10.1016/j.jhazmat.2024.135361
Hu, M., Lu, J., Mei, H., Ding, H., Cai, K., Zhou, H., et al. (2025). Role of calcium salts in modulating benzo [a] pyrene levels in barbecued pork sausages: a study on quality attributes and safety. Food Chem. 463, 141381. doi:10.1016/j.foodchem.2024.141381
Hua, C., Jiang, F., Wang, L., Peng, S., Gao, H., Mo, W., et al. (2023). Protective effects of Pimpinella diversifolia DC. root essential oil against lipopolysaccharide/D-galactosamine-induced acute liver injury in mice through inhibiting inflammation and reducing oxidative stress. Arab. J. Chem. 16 (9), 105017. doi:10.1016/j.arabjc.2023.105017
Huang, S., Zeng, Z., Chu, Y., Zhang, S., Zhou, J., Hu, Z., et al. (2025). Mitigation of lipopolysaccharide-induced intestinal injury in rats by Chimonanthus nitens Oliv. essential oil via suppression of mitochondrial fusion protein mitofusin 2 (MFN2)-mediated mitochondrial-associated endoplasmic reticulum membranes (MAMs) formation. J. Ethnopharmacol. 337, 118856. doi:10.1016/j.jep.2024.118856
Huang, Z., Guo, L., Huang, J., Chen, X., Sun, J., Ye, Y., et al. (2024). Neurotoxicity and brain metabolic dysfunction induced by long-term food-derived arsenic exposure. Food Biosci. 59, 103804. doi:10.1016/j.fbio.2024.103804
Hussain, A. W., Mumtaz, M., Saeed, A. U., and Mumtaz, M. (2025). Toxicokinetics of chemical mixture exposure in the environment. Toxicol. Assess. Comb. Chem. Environ., 13–28. doi:10.1002/9781394158355.ch02
Ivanova, S., Gvozdeva, Y., Staynova, R., Grekova-Kafalova, D., Nalbantova, V., Benbassat, N., et al. (2025). Essential oils–a review of the natural evolution of applications and some future perspectives. Pharmacia 72, 1–12. doi:10.3897/pharmacia.72.e140059
Jebur, A. B., El-Sayed, R. A., and El-Demerdash, F. M. (2022). Ocimum basilicum essential oil modulates hematotoxicity, oxidative stress, DNA damage, and cell cycle arrest induced by β-cyfluthrin in rat liver. Front. Pharmacol. 12, 784281. doi:10.3389/fphar.2021.784281
Jiang, J., Xu, H., Wang, H., Zhang, Y., Ya, P., Yang, C., et al. (2017). Protective effects of lemongrass essential oil against benzo (a) pyrene-induced oxidative stress and DNA damage in human embryonic lung fibroblast cells. Toxicol. Mech. Methods 27 (2), 121–127. doi:10.1080/15376516.2016.1266541
Kačániová, M., Vukic, M., Vukovic, N. L., Čmiková, N., Verešová, A., Schwarzová, M., et al. (2023). An in-depth study on the chemical composition and biological effects of Pelargonium graveolens essential oil. Foods 13 (1), 33. doi:10.3390/foods13010033
Kakouri, E., Daferera, D., Kanakis, C., Revelou, P. K., Kaparakou, E. H., Dervisoglou, S., et al. (2022). Origanum majorana essential oil—a Review of its chemical profile and pesticide activity. Life 12 (12), 1982. doi:10.3390/life12121982
Kashyap, R. (2024). Exploring the molecular mechanisms and therapeutic potentials of essential oils: a systems biology approach. Future Integr. Med. 3 (2), 116–131. doi:10.14218/FIM.2023.00071
Khafaga, A. F., Naiel, M. A., Dawood, M. A., and Abdel-Latif, H. M. (2020). Dietary Origanum vulgare essential oil attenuates cypermethrin-induced biochemical changes, oxidative stress, histopathological alterations, apoptosis, and reduces DNA damage in Common carp (Cyprinus carpio). Aquat. Toxicol. 228, 105624. doi:10.1016/j.aquatox.2020.105624
Khattab, A. A., Taweek, A. M., Abo-EL-Sooud, K., Ahmed, K. A., El-Gendy, A. N., and Ahmed, A. R. (2020). Elettaria cardamomum essential oil rescues paraceta-mol-induced hepatorenal damage via modulating oxidative stress in rats. Adv. Anim. Vet. Sci. 8 (s2), 24–33. doi:10.17582/journal.aavs/2020/8.s2.24.33
Khodaei, N., Houde, M., Bayen, S., and Karboune, S. (2023). Exploring the synergistic effects of essential oil and plant extract combinations to extend the shelf life and the sensory acceptance of meat products: multi-antioxidant systems. J. Food Sci. Technol. 60 (2), 679–691. doi:10.1007/s13197-022-05653-4
Khwaza, V., and Aderibigbe, B. A. (2025). Antibacterial activity of selected essential oil components and their derivatives: a review. Antibiotics 14 (1), 68. doi:10.3390/antibiotics14010068
Kim, M. H., Park, S. J., and Yang, W. M. (2020). Inhalation of essential oil from Mentha piperita ameliorates PM10-exposed asthma by targeting IL-6/JAK2/STAT3 pathway based on a network pharmacological analysis. Pharmaceuticals 14 (1), 2. doi:10.3390/ph14010002
Koubaa, F. G., Chaabane, M., Turki, M., Ayadi, F. M., and El Feki, A. (2021). RETRACTED ARTICLE: anti-oxidant and hepatoprotective effects of Salvia officinalis essential oil against vanadium-induced oxidative stress and histological changes in the rat liver. Environ. Sci. Pollut. Res. 28, 11001–11015. doi:10.1007/s11356-020-11303-z
Lai, Y. S., Chen, W. C., Ho, C. T., Lu, K. H., Lin, S. H., Tseng, H. C., et al. (2014). Garlic essential oil protects against obesity-triggered nonalcoholic fatty liver disease through modulation of lipid metabolism and oxidative stress. J. Agric. Food Chem. 62 (25), 5897–5906. doi:10.1021/jf500803c
Lang, S. M., and Schiffl, H. (2024). Smoking status, cadmium, and chronic kidney disease. Ren. Replace. Ther. 10 (1), 17. doi:10.1186/s41100-024-00533-3
Lanzerstorfer, P., Sandner, G., Pitsch, J., Mascher, B., Aumiller, T., and Weghuber, J. (2021). Acute, reproductive, and developmental toxicity of essential oils assessed with alternative in vitro and in vivo systems. Archives Toxicol. 95 (2), 673–691. doi:10.1007/s00204-020-02945-6
Latoch, A., Czarniecka-Skubina, E., and Moczkowska-Wyrwisz, M. (2023). Marinades based on natural ingredients as a way to improve the quality and shelf life of meat: a review. Foods 12 (19), 3638. doi:10.3390/foods12193638
Lemmens-Gruber, R. (2020). “Adverse effects and intoxication with essential oils,” in Handbook of essential oils (Boca Raton, FL: CRC Press), 517–541.
Li, C., and Tang, M. (2024). The toxicological effects of nano titanium dioxide on target organs and mechanisms of toxicity. J. Appl. Toxicol. 44 (2), 152–164. doi:10.1002/jat.4534
Li, H., Qiao, S., and Zhang, S. (2025). Essential oils in grain storage: a comprehensive review of insecticidal and antimicrobial constituents, mechanisms, and applications for grain security. J. Stored Prod. Res. 111, 102537. doi:10.1016/j.jspr.2024.102537
Limbu, S., and Dakshanamurthy, S. (2023). Predicting dose-dependent carcinogenicity of chemical mixtures using a novel hybrid neural network framework and mathematical approach. Toxics 11 (7), 605. doi:10.3390/toxics11070605
Liñán-Atero, R., Aghababaei, F., García, S. R., Hasiri, Z., Ziogkas, D., Moreno, A., et al. (2024). Clove essential oil: chemical profile, biological activities, encapsulation strategies, and food applications. Antioxidants 13 (4), 488. doi:10.3390/antiox13040488
Lombrea, A., Antal, D., Ardelean, F., Avram, S., Pavel, I. Z., Vlaia, L., et al. (2020). A recent insight regarding the phytochemistry and bioactivity of Origanum vulgare L. essential oil. Int. J. Mol. Sci. 21 (24), 9653. doi:10.3390/ijms21249653
Majumder, S., Reddey, B. R., Pandey, J., Paul, A., Kumar, A., and Banerjee, K. (2024). Pesticide residue and bio-pesticides in vegetable crops. Veg. Sci. 51, 77–96. doi:10.61180/vegsci.2024.v51.spl.08
Manzoor, Q., Sajid, A., Ali, Z., Nazir, A., Sajid, A., Imtiaz, F., et al. (2024). Toxicity spectrum and detrimental effects of titanium dioxide nanoparticles as an emerging pollutant: a review. Desalination Water Treat. 317, 100025. doi:10.1016/j.dwt.2024.100025
Menzikov, S. A., Zaichenko, D. M., Moskovtsev, A. A., Morozov, S. G., and Kubatiev, A. A. (2024). Phenols and GABAA receptors: from structure and molecular mechanisms action to neuropsychiatric sequelae. Front. Pharmacol. 15, 1272534. doi:10.3389/fphar.2024.1272534
Messaoudi, K., Benmeddour, T., and Flamini, G. (2024). First report on the chemical composition and the free radical scavenging and antimicrobial activities of the essential oil of Ononis aurasiaca, an endemic plant of Algeria. Nat. Prod. Res. 38 (24), 4355–4367. doi:10.1080/14786419.2023.2282113
Misra, P., John, S. A., Marker, S., Agnihotry, S., Srivastav, A. K., Sagar, A., et al. (2024). Therapeutic applications and pharmacological practices of essential oils. Aromather. Sci. Essent. Oils 207, 207–275. doi:10.2174/9789815136203124010011
Mohammed, H. A., Sulaiman, G. M., Khan, R. A., Al-Saffar, A. Z., Mohsin, M. H., Albukhaty, S., et al. (2024). Essential oils pharmacological activity: chemical markers, biogenesis, plant sources, and commercial products. Process Biochem. 144, 112–132. doi:10.1016/j.procbio.2024.05.021
Mohammed, H. E., El-Nekeety, A. A., Rashwan, H. M., Abdel-Aziem, S. H., Hassan, N. S., Hassan, E. E., et al. (2025). Screening of bioactive components in Ferula assafo dried oleo-gum resin and assessment of its protective function against cadmium-induced oxidative damage, genotoxicity, and cytotoxicity in rats. Toxicol. Rep. 14, 101853. doi:10.1016/j.toxrep.2024.101853
Mossa, A. T. H., Refaie, A. A., Ramadan, A., and Bouajila, J. (2013). Amelioration of prallethrin-induced oxidative stress and hepatotoxicity in rat by the administration of Origanum majorana essential oil. Biomed. Res. Int. 2013, 859085. doi:10.1155/2013/859085
Murugesh, V., Shetty, D., Kurmi, A., Tirlotkar, S., Shetty, S., and Solanke, S. (2024). A comprehensive review of essential oils for depression management. J. Pharmacogn. Phytochem. 13 (2), 60–70. doi:10.22271/phyto.2024.v13.i2a.14871
Ncir, M., Ben Ali, M., Sellami, H., Allagui, M. S., Lahyani, A., Makni Ayadi, F., et al. (2020). Protective effects of Allium sativum essential oil rich in disulfides against deltamethrin induced oxidative stress and hepatotoxicity in rats. J. Food Meas. Charact. 14, 2667–2675. doi:10.1007/s11694-020-00513-1
Nonci, N., Noveriza, R., Meilin, A., Melati, M., Manzila, I., Pustika, A. B., et al. (2025). Toxicity test of compound essential oil nanoemulsion on fall armyworm (Spodoptera frugiperda JE Smith) eggs. Cogent Food and Agric. 11 (1), 2435584. doi:10.1080/23311932.2024.2435584
Oniga, I., Pușcaș, C., Silaghi-Dumitrescu, R., Olah, N. K., Sevastre, B., Marica, R., et al. (2018). Origanum vulgare ssp. vulgare: chemical composition and biological studies. Molecules 23 (8), 2077. doi:10.3390/molecules23082077
Pokajewicz, K., Białoń, M., Svydenko, L., Fedin, R., and Hudz, N. (2021). Chemical composition of the essential oil of the new cultivars of Lavandula angustifolia Mill. Bred in Ukraine. Molecules 26 (18), 5681. doi:10.3390/molecules26185681
Poveda, J., Vítores, D., Sánchez-Gómez, T., Santamaría, Ó., Velasco, P., Zunzunegui, I., et al. (2025). Use of by-products from the industrial distillation of lavandin (Lavandula x intermedia) essential oil as effective bioherbicides. J. Environ. Manage 373, 123723. doi:10.1016/j.jenvman.2024.123723
Rahman, A. N. A., Mohamed, A. A. R., Mohammed, H. H., Elseddawy, N. M., Salem, G. A., and El-Ghareeb, W. R. (2020). The ameliorative role of geranium (Pelargonium graveolens) essential oil against hepato-renal toxicity, immunosuppression, and oxidative stress of profenofos in common carp, Cyprinus carpio (L.). Aquaculture 517, 734777. doi:10.1016/j.aquaculture.2019.734777
Rehman, N. U., Salkini, M. A. A., Alanizi, H. M., Alharbi, A. G., Alqarni, M. H., and Abdel-Kader, M. S. (2022). Achillea fragrantissima essential oil: composition and detailed pharmacodynamics study of the bronchodilator activity. Separations 9 (11), 334. doi:10.3390/separations9110334
Ren, F., Xu, X., Xu, J., Mei, Y., Zhang, J., Wang, X., et al. (2021). Compound essential oils relieve oxidative stress caused by PM2. 5 exposure by inhibiting autophagy through the AMPK/mTOR pathway. Environ. Toxicol. 36 (9), 1765–1774. doi:10.1002/tox.23297
Roshanaei, K., Dadkhah, A., Fatemi, F., and Dini, S. (2017). Heracleum persicum Essential oil administration in CCL 4 treated Rat sustains antioxidant/oxidative stress statue. Adv. Bioresearch 8 (1). doi:10.15515/abr.0976-4585.8.1.93101
Rovira, A. R. I., Cattley, R. C., and Brown, D. (2024). “Liver and gall bladder,” in Haschek and rousseaux's handbook of toxicologic pathology (Academic Press), 149–247. doi:10.1016/B978-0-12-415759-0.00045-5
Sadgrove, N. J., Padilla-González, G. F., Leuner, O., Melnikovova, I., and Fernandez-Cusimamani, E. (2021). Pharmacology of natural volatiles and essential oils in food, therapy, and disease prophylaxis. Front. Pharmacol. 12, 740302. doi:10.3389/fphar.2021.740302
Sallam, M. F., Ahmed, H. M., Diab, K. A., El-Nekeety, A. A., Abdel-Aziem, S. H., Sharaf, H. A., et al. (2022). Improvement of the antioxidant activity of thyme essential oil against biosynthesized titanium dioxide nanoparticles-induced oxidative stress, DNA damage, and disturbances in gene expression in vivo. J. Trace Elem. Med. Biol. 73, 127024. doi:10.1016/j.jtemb.2022.127024
Salman, A. S., Al-Shaikh, T. M., Hamza, Z. K., El-Nekeety, A. A., Bawazir, S. S., Hassan, N. S., et al. (2021). Matlodextrin-cinnamon essential oil nanoformulation as a potent protective against titanium nanoparticles-induced oxidative stress, genotoxicity, and reproductive disturbances in male mice. Environ. Sci. Pollut. Res. 28, 39035–39051. doi:10.1007/s11356-021-13518-0
Saoudi, M., Badraoui, R., Bouhajja, H., Ncir, M., Rahmouni, F., Grati, M., et al. (2017). Deltamethrin induced oxidative stress in kidney and brain of rats: protective effect of Artemisia campestris essential oil. Biomed. Pharmacother. 94, 955–963. doi:10.1016/j.biopha.2017.08.030
Saravi, S. S. S., and Dehpour, A. R. (2016). Potential role of organochlorine pesticides in the pathogenesis of neurodevelopmental, neurodegenerative, and neurobehavioral disorders: a review. Life Sci. 145, 255–264. doi:10.1016/j.lfs.2015.11.006
Satarug, S. (2024). Is chronic kidney disease due to cadmium exposure inevitable and can it Be reversed? Biomedicines 12 (4), 718. doi:10.3390/biomedicines12040718
Selmi, S., Jallouli, M., Gharbi, N., and Marzouki, L. (2015). Hepatoprotective and renoprotective effects of lavender (Lavandula stoechas L.) essential oils against malathion-induced oxidative stress in young male mice. J. Med. Food 18 (10), 1103–1111. doi:10.1089/jmf.2014.0130
Sharopov, F., Valiev, A., Satyal, P., Gulmurodov, I., Yusufi, S., Setzer, W. N., et al. (2017). Cytotoxicity of the essential oil of fennel (Foeniculum vulgare) from Tajikistan. Foods 6 (9), 73. doi:10.3390/foods6090073
Soni, V., Bharti, D., Bharadwaj, M., Soni, V., Saxena, R., and Arora, C. (2023). “Chapter 12 Toxicity of essential oils,” in Essential oils: sources, 253. Production and Applications, (Berlin, Germany: De Gruyter), 253–268. doi:10.1515/9783110791600-012
Souto, E. B., Blanco-Llamero, C., Krambeck, K., Kiran, N. S., Yashaswini, C., Postwala, H., et al. (2024). Regulatory insights into nanomedicine and gene vaccine innovation: safety assessment, challenges, and regulatory perspectives. Acta Biomater. 180, 1–17. doi:10.1016/j.actbio.2024.04.010
Sule, R. O., Condon, L., and Gomes, A. V. (2022). A common feature of pesticides: oxidative stress-the role of oxidative stress in pesticide-induced toxicity. Oxid. Med. Cell Longev. 2022 (1), 5563759. doi:10.1155/2022/5563759
Tenchov, R., Hughes, K. J., Ganesan, M., Iyer, K. A., Ralhan, K., Lotti Diaz, L. M., et al. (2025). Transforming medicine: cutting-edge applications of nanoscale materials in drug delivery. ACS Nano 19, 4011–4038. doi:10.1021/acsnano.4c09566
Tian, S., Li, R., Li, J., and Zou, J. (2025). Polystyrene nanoplastics promote colitis-associated cancer by disrupting lipid metabolism and inducing DNA damage. Environ. Int. 195, 109258. doi:10.1016/j.envint.2025.109258
Timoumi, R., Salem, I. B., Amara, I., Annabi, E., and Abid-Essefi, S. (2020). Protective effects of fennel essential oil against oxidative stress and genotoxicity induced by the insecticide triflumuron in human colon carcinoma cells. Environ. Sci. Pollut. Res. 27, 7957–7966. doi:10.1007/s11356-019-07395-x
Tiwana, J. K., Shah, A. K., and Dhalla, N. S. (2024). The involvement of reactive oxygen species in causing chronic cardiovascular and neurodegenerative diseases and some cancers. Scr. Medica 55 (2), 199–217. doi:10.5937/scriptamed55-48730
Wei, H. K., Chen, G., Wang, R. J., and Peng, J. (2015). Oregano essential oil decreased susceptibility to oxidative stress-induced dysfunction of intestinal epithelial barrier in rats. J. Funct. Foods 18, 1191–1199. doi:10.1016/j.jff.2015.02.035
Yang, Y., Hassan, M. F., Ali, W., Zou, H., Liu, Z., and Ma, Y. (2025). Effects of cadmium pollution on human health: a narrative review. Atmosphere 16 (2), 225. doi:10.3390/atmos16020225
Yuan, H., Jiang, M., Fang, H., and Tian, H. (2025). Recent advances in poly (amino acids), polypeptides, and their derivatives in drug delivery. Nanoscale 17, 3549–3584. doi:10.1039/D4NR04481A
Zehetner, P., Höferl, M., and Buchbauer, G. (2019). Essential oil components and cytochrome P450 enzymes: a review. Flavour Fragr. J. 34 (4), 223–240. doi:10.1002/ffj.3496
Zhao, J., Ding, K., Hou, M., Li, Y., Hou, X., Dai, W., et al. (2022). Schisandra chinensis essential oil attenuates acetaminophen-induced liver injury through alleviating oxidative stress and activating autophagy. Pharm. Biol. 60 (1), 958–967. doi:10.1080/13880209.2022.2067569
Keywords: essential oil, oxidative stress, chemical overexposure, hepatorenal protective, medicinal potential
Citation: Jiang F, Huang X, Chen X and Xian Y (2025) Hepatorenal protective effects of essential oils against chemical overexposure induced oxidative damage. Front. Pharmacol. 16:1580805. doi: 10.3389/fphar.2025.1580805
Received: 21 February 2025; Accepted: 10 April 2025;
Published: 16 April 2025.
Edited by:
Manuela Oliverio, Magna Græcia University, ItalyReviewed by:
Nasra Ayuob, Damietta University, EgyptCopyright © 2025 Jiang, Huang, Chen and Xian. This is an open-access article distributed under the terms of the Creative Commons Attribution License (CC BY). The use, distribution or reproduction in other forums is permitted, provided the original author(s) and the copyright owner(s) are credited and that the original publication in this journal is cited, in accordance with accepted academic practice. No use, distribution or reproduction is permitted which does not comply with these terms.
*Correspondence: Faming Jiang, amlhbmdmYW1pbmc5ODExQHNpbmEuY29t; Xiaoyan Huang, aHVhbmd4aWFveWFuODIxMUAxNjMuY29t
Disclaimer: All claims expressed in this article are solely those of the authors and do not necessarily represent those of their affiliated organizations, or those of the publisher, the editors and the reviewers. Any product that may be evaluated in this article or claim that may be made by its manufacturer is not guaranteed or endorsed by the publisher.
Research integrity at Frontiers
Learn more about the work of our research integrity team to safeguard the quality of each article we publish.