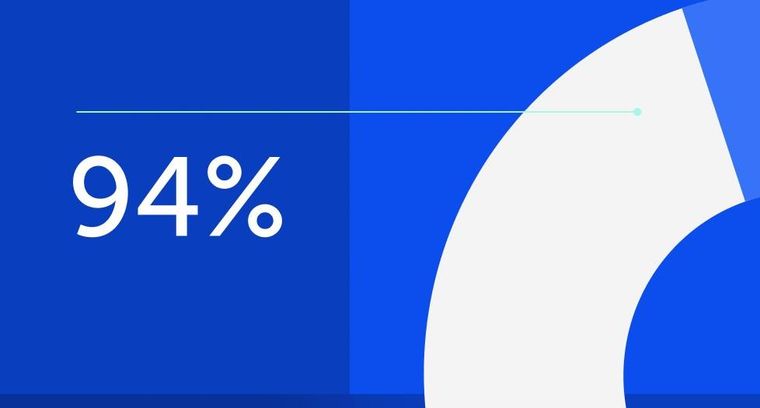
94% of researchers rate our articles as excellent or good
Learn more about the work of our research integrity team to safeguard the quality of each article we publish.
Find out more
REVIEW article
Front. Pharmacol., 09 April 2025
Sec. Experimental Pharmacology and Drug Discovery
Volume 16 - 2025 | https://doi.org/10.3389/fphar.2025.1573684
This article is part of the Research TopicAdvances in Bioactive Compounds: Mechanisms and Therapeutic PotentialsView all 4 articles
Ulcerative colitis (UC), a chronic inflammatory bowel disease, is driven by dysregulated immune responses and persistent intestinal inflammation. Pyroptosis, a caspase/gasdermin-mediated inflammatory cell death that exacerbates mucosal damage through excessive cytokine release and epithelial barrier disruption. Although pyroptosis is considered to be a key mechanism in the pathogenesis of UC, the systematic assessment of the role of natural products in targeting the pyroptosis pathway remains a critical research gap. The purpose of this review is to investigate the regulatory effects of natural products on pyroptosis in UC and elucidate the mechanisms of action and potential therapeutic effects. Key findings highlight polyphenols (e.g., resveratrol), flavonoids (e.g., Quercetin), and terpenoids as promising agents that inhibit NLRP3 inflammasome activation, suppress gasdermin D cleavage, and restore barrier integrity, thereby reducing pro-inflammatory cytokine release in preclinical UC models. Current evidence shows enhanced efficacy and safety when these compounds are combined with standard therapies, but clinical translation requires overcoming three key barriers: limited human trial data, uncharacterized polypharmacology, and suboptimal pharmacokinetics needing formulation refinement. Future research should prioritize standardized animal-to-human translational models, mechanistic studies on synergistic pathways, and rigorous clinical validation to harness the full potential of natural products in pyroptosis-targeted UC therapies.
Ulcerative colitis (UC) is a persistent inflammatory condition of the bowel distinguished by the ulceration and inflammation of the mucosal lining of the colon (Wangchuk et al., 2024). Recent trends in epidemiology indicate a concerning global rise in prevalence, with estimates suggesting that approximately 5 million people may be affected worldwide by 2023 (Le Berre et al., 2023). UC typically manifests with a range of symptoms including abdominal pain, diarrhea, rectal bleeding, and urgency, significantly impairing the quality of life for patients (Gros and Kaplan, 2023; Wangchuk et al., 2024). The disease often follows a relapsing-remitting course, wherein periods of exacerbation are interspersed with remission, making its management particularly challenging (Gajendran et al., 2019). The etiology of UC remains multifactorial, involving genetic predisposition, dysregulation of the immune response, and environmental factors, including diet and gut microbiota (Kazmi et al., 2024; Long et al., 2024a). Current therapeutic strategies aim to induce and maintain remission through the use of anti-inflammatory agents, immunosuppressants, and biologics (Liu et al., 2024). Although these current treatments can achieve some therapeutic effect, they are often accompanied by significant side effects (Zhao L. et al., 2024). Therefore, there is an urgent need for new, safer and more effective treatment strategies.
Recent research has begun to unravel the complex role of pyroptosis, a highly inflammatory form of programmed cell death, in the pathogenesis of UC (Zhen and Zhang, 2019). Pyroptosis is characterized by cell swelling, membrane rupture, and the release of pro-inflammatory cytokines, which can exacerbate tissue damage and inflammation. This process is primarily mediated by inflammasomes, multi-protein complexes that detect pathogenic stimuli and activate caspase-1, leading to the cleavage of gasdermin D (GSDMD) and subsequent cell lysis (Vasudevan et al., 2023). In the context of UC, pyroptosis contributes to the inflammatory response and mucosal injury, highlighting its potential as a therapeutic target.
Natural products have long been a cornerstone of medicine, with many modern pharmaceuticals deriving from compounds found in plants, fungi, and other natural sources. The importance of natural products as an important source for the development of new drugs is highlighted (Ekiert and Szopa, 2020; Zhang L. et al., 2020). As an example, salicylic acid, which is obtained from willow bark, was instrumental in paving the way for aspirin’s creation (Peng B. et al., 2024). Natural products are of interest due to their wide range of biological activities and studies have found that they are often able to enhance their therapeutic potential by modulating multiple targets and pathways (Abdulhakeem Mansour Alhasbary et al., 2025). Recent studies have demonstrated that specific natural compounds can modulate inflammatory responses by influencing pyroptosis pathways, indicating the potential for these compounds to exert their anti-inflammatory effects through a novel mechanism (Zhang et al., 2023; Sun J. et al., 2024). The interplay between natural products and pyroptosis in UC represents a promising area for further research and therapeutic development. By understanding how these compounds modulate the pyroptosis process may lead us to novel approaches to mitigate the inflammatory process in UC. In summary, the close association between pyroptosis and UC, and the therapeutic promise of natural products, make this a new area of widespread interest. Therefore, this review summarizes the role of pyroptosis in UC, and highlights the potential pharmacological mechanisms of natural products targeting pyroptosis in the treatment of UC, and discusses their therapeutic potential in UC to pave the way for future research and clinical application.
A systematic literature search spanning the decade from 2014 to 2024, was conducted across ScienceDirect, PubMed, and Web of Science. The search strategy integrated controlled vocabulary and free-text keywords with Boolean operators, focusing on four conceptual clusters: (1) UC-related terms (“ulcerative colitis” OR “UC”), (2) pyroptosis (“pyroptosis” OR “gasdermin D” OR “caspase-1”), (3) NLRP3 (“NLRP3” OR “NOD-like receptor protein 3”), and (4) therapeutic interventions (“natural products” OR “traditional Chinese medicine” OR “TCM” OR “herb”). The search was restricted to English-language original research and review articles, while excluding non-peer-reviewed publications and conference proceedings during preliminary screening.
Pyroptosis is a form of programmed cell death (Coll et al., 2022). This process is fundamentally different from other types of cell death, such as apoptosis and necrosis, owing to its inflammatory consequences and its role in host defense mechanisms (Ketelut-Carneiro and Fitzgerald, 2022). Traditionally recognized as a response to infection, pyroptosis has been implicated in a range of pathologies, including inflammatory disorders (Dai Z. et al., 2023), cancer (Yang et al., 2022), and neurodegenerative diseases (Voet et al., 2019). Thus, a comprehensive understanding of the molecular mechanisms governing pyroptosis could provide new opportunities to find natural products that target pyroptosis for the treatment of UC.
The onset of pyroptosis is mainly regulated by the activation of inflammasomes, which are multiprotein complexes (Man and Kanneganti, 2015). These inflammasomes are formed in response to various stimuli, including pathogen-associated molecular patterns (PAMPs) and damage-associated molecular patterns (DAMPs) (Zhan et al., 2022a). The most well-studied inflammasomes include NLRP3, absent in melanoma 2 (AIM2), and NLRC4, each responding to distinct signals. Upon detection of these signals, pattern recognition receptors (PRRs) such as NOD-like receptors (NLRs) and AIM2 oligomerize and recruit apoptosis-associated speck-like protein containing a CARD (ASC), forming a large protein scaffold. This recruitment facilitates the activation of caspase-1, an essential protease in the pyroptotic pathway. Activated caspase-1 cleaves pro-inflammatory cytokines such as pro-IL-1β and pro-IL-18 into their active forms, leading to their secretion and subsequent inflammatory response. Additionally, caspase-1 cleaves GSDMD, leading to the formation of pores in the cell membrane that characterize pyroptosis (Zhang W. J. et al., 2021; Zhan et al., 2022a).
The gasdermin family of proteins plays a pivotal role in the execution of pyroptosis. Among them, GSDMD has emerged as the central player. Upon cleavage by caspase-1, the N-terminal domain of GSDMD translocates to the plasma membrane, where it oligomerizes and forms large pores. These pores facilitate the influx of water and ions, causing cell swelling and eventual lysis (Shi et al., 2017; Broz et al., 2020). This contrasts with apoptosis, where cell shrinkage and membrane blebbing occur without lysis. Recent studies have also identified other gasdermin family members, such as GSDME (gasdermin E), which can mediate pyroptosis in a caspase-3 dependent manner, linking apoptosis and pyroptosis in certain contexts. Gasdermins serve a dual function in apoptosis and pyroptosis, showcasing a complex interaction that can affect cell fate, which is dependent on the surrounding microenvironment and the nature of the cellular stress encountered (Jiang et al., 2020).
The release of pro-inflammatory cytokines during pyroptosis not only contributes to local inflammation but also influences systemic responses. IL-1β and IL-18 are the hallmark cytokines released during pyroptosis, and their secretion can amplify inflammatory responses, recruit immune cells, and promote further pyroptotic cell death in neighboring cells (Zheng et al., 2023). Cytokines play a key role in the inflammatory response, but their activation and release are tightly controlled to maintain organismal homeostasis. Disruption of this regulation may play a role in the emergence of several pathological conditions, such as autoinflammatory syndromes and chronic inflammatory diseases (Tan et al., 2021; Atabaki et al., 2023).
In essence, the process of pyroptosis is typically initiated through the assembly of inflammasomes, which are critical components of the innate immune system. The activation of these inflammasomes may take place through two major routes: the canonical pathway (Figure 1A) and the non-canonical pathway (Figure 1B). The canonical route is chiefly marked by the identification of PAMPs or DAMPs, which leads to the activation of caspase-1. Conversely, the non-canonical route can be initiated by the intracellular detection of specific bacterial lipopolysaccharides, which activate caspase-4, -5, or -11. Ultimately, both pathways converge, resulting in a pro-inflammatory response that stimulates the maturation and secretion of cytokines like IL-1β and IL-18, alongside the disruption of the cell membrane, culminating in cell death. This mechanism not only acts as a defense mechanism against infections but also contributes to the modulation of the inflammatory environment in various disease conditions.
Figure 1. Mechanisms of pyroptosis activation. This diagram illustrates the two primary pathways involved in the initiation of pyroptosis: the canonical and non-canonical pathways. The canonical pyroptosis pathway is activated by the detection of pathogen-associated molecular patterns (PAMPs) or damage-associated molecular patterns (DAMPs), leading to the assembly of inflammasomes and the activation of caspase-1 (A). In contrast, the non-canonical pyroptosis pathway is triggered by the intracellular sensing of bacterial lipopolysaccharides (LPS), resulting in the activation of caspase-4, -5, or -11 (B). Both pathways converge to elicit a robust pro-inflammatory response characterized by the maturation and secretion of cytokines such as IL-1β and IL-18. This inflammatory response ultimately leads to the permeabilization of the cell membrane and the execution of cell death through pyroptosis.
The pathogenesis of UC is multifactorial and includes genetic susceptibility, environmental triggers, and dysregulated immune responses (Digby-Bell et al., 2020; Peng J. et al., 2024). Increasing evidence suggests that pyroptosis plays an important role in the inflammatory process associated with UC (Yang et al., 2024).
Pyroptosis plays a critical role in the pathogenesis and inflammation of UC, as evidenced by elevated levels of caspase-1 and IL-1β in the colonic tissues of UC patients, indicating active pyroptotic processes (Gong et al., 2018). The activation of the NLRP3 inflammasome plays a crucial role in worsening colonic inflammation by triggering the release of pro-inflammatory cytokines and chemokines, which sustains the inflammatory process (Bian et al., 2024a). Additionally, dysregulation of pyroptosis has been linked to impaired epithelial barrier function in UC, allowing for increased microbial translocation and further immune activation (Yang et al., 2024). This relationship extends to the gut microbiome, which is crucial for maintaining intestinal homeostasis; dysbiosis is commonly associated with UC. Pyroptosis can change the composition and function of the gut microbiota by releasing inflammatory cytokines and damage-associated signals, which may lead to a cycle of inflammation and dysbiosis. On the other hand, certain microbial metabolites can influence inflammasome activation and pyroptosis, demonstrating a bidirectional relationship (Pan H. et al., 2022; Zhang Y. et al., 2024). Upon pathogen invasion, inflammasomes such as NLRP6, NLRC4, and AIM2 in epithelial cells are activated, leading to the secretion of IL-18. This cytokine stimulates intestinal epithelial or immune cells to produce IL-22, which promotes the production of antimicrobial peptides and proteins necessary for intestinal epithelial repair. The activation of inflammasomes can also induce pyroptosis through the assembly of GSDMD protein, forming membrane pores that cause cell swelling and death, thereby releasing intracellular bacteria and stimulating an immune response to enhance mucosal defense. The NLRP6 inflammasome in goblet cells not only facilitates IL-18 secretion and pyroptosis but also regulates autophagy to promote mucin protein secretion, helping to maintain intestinal epithelial integrity. Meanwhile, the NLRP12 inflammasome primarily modulates immune signaling pathways to prevent excessive inflammation. The NLRP3 inflammasome plays a dual role in lamina propria immune cells, activating inflammatory responses to bolster mucosal defense under homeostatic conditions, yet contributing to pathological damage during excessive infections (Figure 2) (Rathinam et al., 2019; Liu M. et al., 2023; Liu X. et al., 2023). The complex relationship among pyroptosis, immune regulation and inflammation highlights the potential for targeting these pathways in the development of therapeutic strategies for UC.
Figure 2. Inflammasomes and intestinal mucosal immunity. This illustration depicts the activation of inflammasomes (NLRP6, NLRC4, AIM2, NLRP12, NLRP3) in response to pathogens. Key processes include IL-18 secretion, IL-22 production, pyroptosis, and mucin secretion, highlighting their roles in antimicrobial defense and maintaining intestinal epithelial integrity.
Potential biomarkers discovery is essential for prognosis, diagnosis, and monitoring of disease activity in UC (Wangchuk et al., 2024). Recent studies have identified several potential biomarkers linked to pyroptosis that may be useful in clinical settings. Elevated levels of active caspase-1 in colonic tissue have been associated with increased inflammation in UC (Zhou et al., 2023b). Detection of caspase-1 activity provides insight into the extent of pyroptosis and inflammation and helps to grade patients according to the severity of the disease. Pro-inflammatory cytokines such as IL-1β and IL-18 are released during pyrolysis and are frequently elevated in patients with UC. Monitoring serum levels of these cytokines could serve as a reliable biomarker for assessing disease activity and response to therapy (Wang et al., 2020). For instance, a significant decrease in IL-1β levels following treatment might indicate effective modulation of the inflammatory process (Dai Y. et al., 2023; Ren et al., 2024). GSDMD as a key executor of pyroptosis, the presence of cleaved GSDMD in stool samples or plasma could serve as a novel biomarker for pyroptosis in UC. Its measurement could help determine the degree of pyroptotic activity in the intestinal epithelium, providing a direct link to epithelial cell death and inflammation (Lin et al., 2024a). The gut microbiome plays a pivotal role in regulating inflammation through the production of various metabolites. Certain microbial metabolites, such as short-chain fatty acids (SCFAs), may influence pyroptosis and could be explored as biomarkers reflecting the state of the microbiome and its interaction with the host immune response (Lv et al., 2024). Assessing the infiltration of specific immune cell types, such as neutrophils and Th17 cells, in colonic biopsies may also provide indirect biomarkers of pyroptotic activity. The presence of these cells correlates with inflammation and could indicate ongoing pyroptotic processes (Stakenborg et al., 2020).
Targeting the pyroptotic pathway offers a promising strategy for developing new therapeutic interventions for UC. Several potential therapeutic targets have emerged, including caspase-1 inhibitors, the preclinical studies have shown that inhibiting caspase-1 can reduce the severity of colitis in animal models, suggesting that pharmacological modulation of this enzyme may provide therapeutic benefits (Cao et al., 2024). Additionally, targeting the NLRP3 inflammasome directly presents another avenue for intervention. Compounds that inhibit NLRP3 activation could potentially reduce the inflammatory response in UC. For instance, small molecules or natural compounds that disrupt the assembly of the inflammasome complex might prevent downstream cascades leading to pyroptosis (Chen et al., 2021). Moreover, since GSDMD is a crucial executor of pyroptosis, therapies aimed at inhibiting its activity may help control excessive inflammation. Understanding the structural dynamics of GSDMD and its interaction with caspases could lead to the development of targeted inhibitors that prevent pore formation and subsequent cell lysis (Wang et al., 2021). Given the central role of IL-1β and IL-18 in promoting inflammation, targeted therapies that block these cytokines could be beneficial. Existing IL-1β antagonists (e.g., anakinra) and IL-18 binding proteins are being explored in various inflammatory conditions and could be repurposed for UC treatment (Peiró et al., 2017; Liso et al., 2022; Park et al., 2022). Furthermore, restoring a healthy gut microbiome through probiotics, prebiotics, and dietary interventions may influence pyroptosis indirectly by balancing immune responses (Darb Emamie et al., 2021; LeBlanc et al., 2021). In short, combination therapies targeting multiple components of the pyroptotic pathway, alongside traditional immunosuppressive agents, may offer a more effective treatment approach than single-agent therapies.
In recent years, natural products derived from plants have attracted much attention because of their potential to target pyroptosis in the treatment of UC (Xue et al., 2023; Chen B. et al., 2024). Among them, alkaloids, such as berberine, also contribute to UC management through their gut-protective and anti-inflammatory actions (Zhao X. et al., 2022). Polyphenols, abundant in fruits, vegetables, and so on, demonstrate significant anti-inflammatory effects, with notable examples being resveratrol (Wang S. et al., 2022). Additionally, flavonoids have been extensively studied for their notable anti-inflammatory and antioxidant properties (Fan et al., 2022). The mechanisms by which these natural products act include inhibition of inflammasome activation, particularly the NLRP3 inflammasome, thereby reducing the release of proinflammatory cytokines such as IL-1β and IL-18. In addition, they can regulate the release of inflammatory cytokines by down-regulating the NF-κB signaling pathway, thereby promoting a more balanced immune response. Additionally, many natural products enhance epithelial cell survival and intestinal barrier function, protecting against oxidative stress and inflammation, which helps maintain gut integrity and prevent exacerbation of inflammatory responses. Collectively, these findings underscore the promising role of natural products in the therapeutic management of UC through the modulation of pyroptosis. Therefore, this part summaries the role and regulatory mechanisms of several classes of natural products, including flavonoids, polyphenols and alkaloids, among others, in the treatment of UC through influencing pyroptosis. The detailed dosages and mechanisms of these natural products are summarized in Table 1 and their mechanisms are summarized in Figure 4A.
Flavonoids are a diverse group of polyphenolic compounds with a wide range of biological activities. Figure 3A illustrates the currently reported flavonoids that target pyroptosis for the treatment of UC. Among them, Quercetin is particularly notable for its multifaceted beneficial properties, which include antioxidant, antiviral, anticancer, anti-inflammatory, and antimicrobial effects. Notably, Quercetin has been shown to protect macrophages from pyroptosis, partially by inhibiting inflammasome activity (Luo et al., 2022). Furthermore, in models of UC, Quercetin has been reported to reduce the expression of TLR4, inhibit cellular pyroptosis, and ameliorate the intestinal inflammatory response, particularly during the active phase of the disease (Zhao Y. et al., 2024). Another significant flavonoid is Hydroxysafflor yellow A (HSYA), a primary water-soluble chalcone glycoside derived from Carthamus tinctorius L. Previous studies have demonstrated HSYA’s exceptional anti-inflammatory and antioxidant properties (Luo et al., 2020). It has been shown to decrease pro-inflammatory cytokines, such as IL-1β, IL-6, and TNF-α, while also inhibiting NLRP3/GSDMD-mediated pyroptosis in vitro. Additionally, HSYA downregulates hexokinase 1 (HK1), and in vivo studies indicate that it alleviates UC symptoms, including body weight loss and inflammatory infiltration, while modulating gut microbiota by reducing Proteobacteria and increasing Bacteroidetes (Chen et al., 2023b). Jaceosidin, a flavonoid extracted from Artemisia princeps, is recognized for its antioxidant, anti-inflammatory, and anticancer properties. It has been found to significantly inhibit LPS-induced oxidative stress and the accumulation of inflammatory cytokines, as well as NLRP3-mediated cell lysis in LPS-induced NCM460 cells. Moreover, jaceosidin activates the SIRT1/NRF2 pathway, leading to the inhibition of NLRP3-mediated pyroptosis, thereby protecting NCM460 cells from this form of cell death (Lv et al., 2023). Lonicerin, a flavonoid glycoside derived from Lonicera japonica Thunb, has been identified as a major component with anti-inflammatory and immunomodulatory activities (Lin et al., 2024b). Research indicates that lonicerin targets EZH2 to alleviate UC symptoms through the autophagy-mediated inactivation of NLRP3 inflammatory vesicles (Lv et al., 2021). Phloretin, a dihydrochalcone flavonoid primarily found in apples and strawberries, effectively suppresses pro-inflammatory cytokines by modulating the NF-κB, TLR4, and PPARγ signaling pathways. It also inhibits NLRP3 inflammasome activation and reduces markers of oxidative stress. Phloretin is shown to regulate the levels of zonula occludens-1 (ZO-1) and occludin, lower serum LPS concentrations, and rebalance the gut microbiota by modulating the populations of Escherichia coli and Lactobacillus (Zhang et al., 2019). Pelargonidin-3-galactoside (Pg3gal), derived from purple sweet potatoes, has been found to significantly alleviate DSS-induced UC in mice. Its protective effects include reducing inflammation, inhibiting pyroptosis in intestinal epithelial cells, and restoring the balance of gut microbiota (Chen J. et al., 2024). In addition to these key flavonoids, several other natural products have shown promise in the treatment of UC. Such as Naringin, Oroxindin, Rutin, Trifolirhizin, Carthamin yellow, Galangin, and Nicotiflorin. Collectively, these flavonoids demonstrate significant potential as therapeutic agents in the management of UC, highlighting their multifaceted roles in modulating inflammatory pathways and gut microbiota.
Figure 3. Types of natural products and representative compounds targeting pyroptosis for UC treatment. (A) Flavonoids; (B) Phenylpropanoids; (C) Polyphenols; (D) Alkaloids; (E) Terpenoids; (F) Steroids; (G) Others.
Figure 3B illustrates the currently reported phenylpropanoids that target pyroptosis for the treatment of UC. Honokiol, an active natural compound sourced from Magnolia officinalis, has a historical use in traditional medicine for addressing infections, inflammation, and gastrointestinal disorders (Lee et al., 2011). Recent studies indicate that honokiol significantly alleviates UC severity by reducing inflammatory responses and restoring colonic integrity. It has been shown to lower the levels of pro-inflammatory cytokines such as TNF-α, IL-6, IL-1β, and IFN-γ, while enhancing the expression of PPAR-γ. Furthermore, Honokiol inhibits the TLR4-NF-κB signaling pathway, a critical pathway in inflammation, and reduces gasdermin-D-mediated pyroptosis, indicating a robust anti-inflammatory profile that may offer a promising therapeutic strategy for UC management (Wang N. et al., 2022). Isofraxidin, possesses a diverse array of beneficial properties, including anti-inflammatory, antioxidant, anti-cancer, and anti-fatigue effects (Shen et al., 2017). Recent studies have demonstrated that Isofraxidin can alleviate DSS-induced UC by modulating the S1PR1 and IL-17 signaling pathways (Huang Y. et al., 2024). Furthermore, it has been shown to inhibit pyroptosis by upregulating the Nrf2 protein, facilitating its translocation into the nucleus, and reducing ROS levels (He et al., 2024). Schisandrin B, the primary active component of Schisandra chinensis, has exhibited a range of biological activities, including anticancer, anti-inflammatory, hepatoprotective, and antibacterial effects. Notably, Schisandrin B has been found to inhibit the activation of the NLRP3 inflammasome, leading to reduced levels of IL-1β and pyroptosis in intestinal epithelial cells within UC models. This protective effect is mediated through the activation of AMPK and Nrf2-dependent signaling pathways, as well as the alleviation of ROS-induced mitochondrial damage. These findings suggest that Schisandrin B represents a promising therapeutic strategy for the treatment of acute colitis (Zhang W. et al., 2021). In addition to the phenylpropanoids listed above, phenylpropanoids such as Schisandrin, Chlorogenic acid, Nodakenin, Fraxetin, Cinnamaldehyde, Ferulic acid and Sinapic acid, have shown promising potential for improving UC. Collectively, these phytochemicals collectively illuminate pyroptosis inhibition as a strategic axis in treating UC, while underscoring the therapeutic value of natural compounds in modulating inflammasome-immune crosstalk.
Emerging evidence highlights the therapeutic potential of polyphenols in alleviating intestinal inflammation, particularly in the context of UC. Figure 3C illustrates the currently reported polyphenols that target pyroptosis for the treatment of UC. Resveratrol, a stilbene-polyphenol predominantly found in the skin of grapes and certain berries, possesses significant health benefits, including anti-inflammatory, neuroprotective, antioxidative, and anticancer properties (Zhang L. X. et al., 2021). Research has shown that Resveratrol can effectively inhibit LPS and ATP-induced sepsis in HT29 cells. This inhibition occurs through the downregulation of the NF-κB signaling pathway, leading to reduced expression of sepsis-associated proteins and inflammatory mediators. Such findings underscore the therapeutic potential of Resveratrol for managing UC (Zhao P. et al., 2024). Gallic acid (GA), a polyphenolic compound known for its broad pharmacological activities, particularly its anti-inflammatory effects, has shown promise in ameliorating DSS-induced UC by inhibiting the NLRP3 inflammasome (Yu T. Y. et al., 2023). Atranorin, a secondary metabolite derived from lichens, demonstrates significant anti-inflammatory properties by inhibiting nitric oxide production, leukotriene B4 biosynthesis, and cyclooxygenase-1 activity. Toxicity assays in rats have confirmed its safety for in vivo use. Atranorin has been identified as a novel inhibitor of the NLRP3 inflammasome, effectively blocking ASC oligomerization and cytokine release in macrophages and dendritic cells. In various disease models driven by inflammasome activation, such as acute inflammation, gouty arthritis, and UC, Atranorin has been shown to significantly reduce levels of IL-1β and IL-18, underscoring its therapeutic potential (Wang H. Y. et al., 2023). Forsythia suspensa polyphenols have demonstrated efficacy through a multifaceted approach, including the inhibition of M1 macrophage polarization, promotion of thermal apoptosis, and modulation of overall apoptosis. Additionally, these polyphenols facilitate M2 polarization in J774A.1 cells and exhibit protective effects against DSS-induced UC in murine models. They also play a critical role in regulating intestinal homeostasis by enhancing gut microbiota and increasing SCFAs production, key metabolites for gut health (Lv et al., 2024). Apple polyphenols extract (APE), sourced from red Fuji apples, has been attributed with multiple health-promoting effects, including anti-inflammatory, antioxidant, and anti-tumor properties. Recent studies have demonstrated that APE administration can ameliorate acute UC by preserving intestinal barrier integrity and inhibiting both apoptosis and pyroptosis (Liu et al., 2021). In addition to the polyphenols listed above, polyphenolic compounds such as Thyme (Thymus vulgaris L.) polyphenols and Polyphenol extracts from Ziziphus jujuba Mill. have shown promising potential for improving intestinal health and reducing intestinal inflammation. Collectively, these natural polyphenols provide new ideas for the treatment of UC through various mechanisms, such as modulation of immune response, improvement of microbiota, and antioxidant activity.
Figure 3D illustrates the currently reported alkaloids that target pyroptosis for the treatment of UC. Oxymatrine, a principal alkaloid derived from Sophora flavescens Aiton of the Leguminosae family, exhibits a range of pharmacological effects, notably analgesic and anti-inflammatory properties (Chen et al., 2017; Sun J. et al., 2024). Recent studies have demonstrated that Oxymatrine effectively alleviates UC in TNBS-induced rat models by significantly reducing colonic ulcers and inhibiting pyroptosis via the NLRP3 inflammasome pathway. Specifically, treatment with Oxymatrine at a dosage of 80 mg/kg resulted in a notable decrease in levels of NLRP3, active caspase-1, and cleaved IL-1β in lesion tissues within a mere 24 h. In vitro experiments revealed that Oxymatrine at concentrations of 100 and 250 μM diminished cell death and lowered levels of active caspase-1, GSDMD, and cleaved IL-1β in RAW264.7 cells and peritoneal macrophages, underscoring its potential as a therapeutic strategy for UC (Sun J. et al., 2024). Another promising alkaloid, Nigeglanine, has been shown to inhibit pyroptosis in colonic epithelial cells by obstructing the NF-κB and MAPK signaling pathways, along with the NLRP3 inflammatory vesicles. Notably, treatment with Nigeglanine resulted in increased levels of ZO-1 and occludin proteins, which are crucial for maintaining intestinal barrier integrity, thereby potentially slowing the progression of UC (Gao et al., 2019). Berberine (BBR), recognized for its anti-inflammatory properties, is particularly relevant in the context of colitis management. Research indicates that Berberine can inhibit the activation of the Wnt/beta-catenin pathway through the modulation of the miR-103a-3p/BRD4 axis. This mechanism contributes to the attenuation of colitis-induced pyroptosis and aids in the restoration of intestinal mucosal barrier integrity (Zhao X. et al., 2022). Evodiamine (EVO), extracted from the traditional Chinese medicinal plant Evodia rutaecarpa, has been documented to possess a spectrum of pharmacological activities, including anti-inflammatory and anti-tumor effects (Li D. et al., 2022). EVO has been observed to significantly reduce the production of pro-inflammatory cytokines such as TNF-α, IL-1β, and IL-6, thereby suppressing inflammation associated with UC. This anti-inflammatory effect is mediated through the modulation of NF-κB signaling and the NLRP3 inflammatory vesicles (Shen et al., 2019). Additionally, Nigakinone, the primary active component of Ramulus et Folium Picrasmae, a traditional Chinese medicine (TCM) commonly used for treating dysentery, colitis, and gastroenteritis (Liu F. et al., 2020). Nigakinone exerts its protective effects by attenuating the inflammatory response linked to NLRP3 activation and by preserving the integrity of the intestinal mucosal barrier. Furthermore, it regulates bile acid metabolism and mitigates bile acid accumulation through FXR-mediated modulation of cholesterol hydroxylase. Molecular docking studies have suggested that FXR serves as a target for Nigakinone, and inhibition of FXR diminishes the protective effects of Nigakinone, highlighting its role in the modulation of the FXR/NLRP3 signaling pathway in the context of colitis (Liu F. et al., 2023). Gentianine (GTN), a prominent active compound derived from Gentiana scabra, has been recognized for its anti-inflammatory and anti-diabetic properties. Studies indicate that GTN effectively mitigates DSS-induced UC in mice and exhibits protective effects on inflamed colonic tissues in vitro. Notably, Toll-like receptor 4 (TLR4) has been identified as a direct target of GTN, suggesting that its therapeutic effects may stem from the modulation of TLR4, which subsequently reduces the activation of the NLRP3 inflammasome signaling pathway (Li Y. X. et al., 2024). Additionally, alkaloids such as 8-oxypalmatine, Aegeline, Sanguinarine, Coptisine, Brtaine, Betaine, and Demethyleneberberine have shown potential therapeutic effects in the context of UC and related inflammatory conditions. Collectively, this emerging evidence highlights that plant-derived alkaloids, including Oxymatrine, Nigeglanine, Berberine, and Evodiamine, exert therapeutic effects on UC by targeting pyroptosis through multi-pathway modulation of NLRP3 inflammasomes, NF-κB/MAPK signaling, and intestinal barrier restoration, offering novel strategies for inflammation resolution and mucosal repair.
Figure 3E illustrates the currently reported terpenoids that target pyroptosis for the treatment of UC. The triterpene Celastrol (CSR) exhibits potent anti-inflammatory activities across multiple experimental models by disrupting NF-κB signaling pathways, which results in the downregulation of pro-inflammatory cytokines, including IL-1β. Combination therapy using CSR and the compoundCP-456773 has been shown to significantly improve colonic health by reducing colon shortening, disease activity index, and markers of inflammation. This combined treatment enhances antioxidant defenses, inhibits NLRP3 inflammasome activation through modulation of NF-κB and heat shock protein 90 (HSP-90), and promotes autophagy. These findings highlight the considerable potential of celastrol in treating inflammatory bowel disease and other conditions associated with NLRP3 inflammasome activation (Saber et al., 2020). In parallel, emerging studies highlight the therapeutic potential of Ginsenoside Rg3 in the management of DSS-induced UC. This compound alleviates colitis by inhibiting the activation of the NLRP3 inflammasome, as well as mitigating pyroptosis and apoptosis. Notably, Ginsenoside Rg3 also partially restores the disrupted gut microbiota at both the phylum and genus levels, indicating its modulatory impact on microbial composition. Additionally, Ginsenoside Rg3 appears to influence intestinal metabolism, suggesting a complex interplay between microbiota remodeling and inflammasome inhibition in its therapeutic effects against DSS-induced UC (Liu D. et al., 2023). Artemisinin, derived from Artemisia annua, exhibits potent anti-inflammatory and immunosuppressive effects against autoimmune diseases. Its novel water-soluble analog, SM934 (β-aminoarteether maleate), shows strong immunosuppressive activity with reduced side effects. In a study involving TNBS-induced colitis, SM934 effectively restored body weight and colon length while improving intestinal pathology. It protects intestinal barrier function by decreasing permeability, maintaining tight junction proteins and prevents epithelial cell apoptosis and pyroptosis. Furthermore, SM934 has been shown to inhibit the key cleavage-associated factors and block the MAPK and NF-kB signaling pathways in colonic tissues, providing a potential therapeutic avenue for treating UC (Shao et al., 2022). In addition, terpenoids components such as Brusatol, Bryodulcosigenin, 20(S)-Protopanaxadiol saponins, Mogrol, Pulsatilla chinensis saponins, and 1,8-cineole likewise exhibited significant anti-inflammatory and protective effects, which further enriches the potential of natural products in the treatment of UC diseases. In summary, these terpenoids demonstrate significant promise as therapeutic agents in the management of UC. Their various mechanisms, such as the modulation of important signaling pathways and the inhibition of pro-inflammatory cytokine production, provide a solid basis for further research and potential clinical use in treating inflammatory bowel diseases.
Figure 3F illustrates the currently reported steroids that target pyroptosis for the treatment of UC. β-Sitosterol, a primary compound found in phytosterols, is a plant-derived natural product prevalent in various regions. Research indicates that β-sitosterol offers protective effects against (DSS-induced UC by mitigating weight loss and colon length reduction, alleviating macroscopic damage, decreasing pro-inflammatory factor production, and inhibiting NLRP3/caspase-1/GSDMD-mediated pyroptosis and inflammation. Furthermore, it enhances colonic barrier integrity, positioning it as a promising therapeutic agent for UC (Zhang et al., 2023). Ruscogenin, a significant steroidal glycoside extracted from Radix Ophiopogon japonicus, is recognized for its protective effects against inflammatory responses associated with various inflammatory diseases. Recent studies elucidate the potent protective effects of Ruscogenin against DSS-induced colitis, demonstrating its ability to alleviate the condition in mice by inhibiting the activation of the NLRP3 inflammasome and the caspase-1-dependent canonical pyroptosis. This protective mechanism is likely mediated through the inhibition of the TLR4/NF-κB signaling pathway (Li J. et al., 2024). Physalin B, a primary active withanolide extracted from Physalis alkekengi L. var. franchetii (Mast.) Makino, has demonstrated significant anti-inflammatory effects at non-cytotoxic doses. In LPS-stimulated cells, Physalin B notably reduced the levels of pro-inflammatory cytokines, including TNF-α, IL-6, and IL-1β. Furthermore, in a DSS-induced UC model in mice, Physalin B alleviated weight loss, improved clinical signs, and mitigated pathological damage. This effect is attributed to its ability to inhibit the activation of NF-κB, signal transducer and activator of transcription 3 (STAT3), β-arrestin1, and the NLRP3 inflammasome (Zhang Q. et al., 2020). Similarly, other steroids, such as Dioscin (Cai et al., 2021) has also been implicated in anti-inflammatory activities and may contribute to the therapeutic landscape for UC. Together, these findings highlight the importance of steroids such as Dioscin, Ruscogenin, and β-Sitosterol in treating UC, showing their diverse mechanisms of action involving both immune regulation and microbiota management.
Figure 3G illustrates the currently reported other compounds that target pyroptosis for the treatment of UC. Diacetylrhein (DAR), an anti-inflammatory compound traditionally used for osteoarthritis, has demonstrated efficacy in targeting the NLRP3 inflammasome. Recent findings indicate that DAR inhibits inflammasome assembly and reduces caspase-1 activity, along with the inflammatory cytokines IL-1β and IL-18, thereby curbing the pyroptosis process. Additionally, DAR exhibits notable anti-apoptotic effects by modulating the balance of pro-apoptotic and anti-apoptotic proteins. It disrupts NF-κB signaling, leading to improved microscopic features in inflamed colon tissue and a reduced colon weight-to-length ratio, indicative of diminished disease activity and macroscopic damage. Furthermore, DAR is associated with decreased myeloperoxidase activity, IL-6, and TGF-β levels, as well as an increased expression of tight junction proteins, including occludin and ZO-1 (Zohny et al., 2022).
Salidroside, an active compound derived from Rhodiola rosea L., exhibits a spectrum of pharmacological activities, including anti-inflammatory, anti-cancer, and antioxidant properties (Xue et al., 2019). Recent research indicates that salidroside significantly slows the progression of UC by inhibiting pyroptosis in intestinal macrophages, enhancing the diversity of the intestinal microbial community, and modulating the Th17/Treg cell ratio (Liu X. et al., 2023). Furthermore, salidroside effectively alleviates dextran sulfate sodium (DSS)-induced colitis in murine models, primarily through the reduction of inflammation, the inhibition of pro-inflammatory pathways, the upregulation of PPARγ expression, and the restoration of intestinal barrier function (Liu et al., 2019).
Pectic polysaccharides (PPs) derived from Rauwolfia verticillata var. Hainanensis have also been shown to inhibit caspase-1 and IL-1β expression while promoting cellular pyroptosis, thereby impeding the progression of UC (Cui et al., 2022). Another study reported that PPs limit miR-124-3p and target RBP4, reducing the binding affinity of RBP4 to NLRP3. This inhibition of NLRP3-mediated pyroptosis leads to a decrease in pyroptosis of colonic epithelial cells and alleviates mucosal damage in UC (Yuan et al., 2023).
Trans-10-Hydroxy-2-Decenoic Acid (10-HDA) has been shown to effectively alleviate DSS-induced UC in mice by modulating the NLRP3 inflammasome-mediated pyroptotic pathway and enhancing colonic barrier function. Administration of 10-HDA significantly reduces pathological damage, ROS accumulation, neutrophil infiltration, and cytokine production in colonic tissues. It lowers the expression levels of several inflammatory markers, including TXNIP, NLRP3, ASC, caspase-1, GSDMD, IL-1β, and IL-18. Additionally, 10-HDA enhances colonic barrier integrity by increasing the expression of tight junction proteins ZO-1 and occludin. In vitro studies indicate that pretreatment with 10-HDA inhibits inflammasome-mediated pyroptosis in LPS/ATP-stimulated THP-1 cells, supporting its protective effects against colitis (Huang et al., 2022). In summary, various natural compounds like DAR, Salidroside, PPs, 10-HDA, and others have shown promising results in reducing UC by inhibiting NLRP3 inflammasome-mediated pyroptosis and inflammation. These compounds provide potential therapeutic strategies for treating UC by improving colonic barrier function, decreasing oxidative stress, and regulating inflammatory pathways.
TCM has been explored as a therapeutic option. Table 2 illustrates the currently reported TCM targeting pyroptosis for the treatment of UC, including formulas, extracts and other classes. Among various TCM formulations, the Xianglian pill (XLP) has gained attention for its effectiveness in UC treatment. Xianglian pill (XLP) is a TCM widely used in the treatment of UC (Zhang J. X. et al., 2024). Studies have reported that XLP could suppress myeloperoxidase (MPO) activity and decreased serum levels of inflammatory cytokines, including IL-1β, IL-6, TNF-α, and IL-18. Furthermore, XLP could inhibit the expression of key proteins associated with inflammation and apoptosis, such as GSDMD-N, TLR4, NLRP3, active-caspase-1, MyD88, and p-NF-κB/NF-κB in the colon tissues (Dai Y. et al., 2023). Another formulation, Kuijieling decoction (KJL), has been clinically utilized for several years, demonstrating significant therapeutic effects in UC management (Xiao et al., 2024). KJL treatment has been shown to markedly attenuate colonic injury while reducing inflammatory markers such as ASC, caspase-1, IL-1β, IL-18, NLRP3, and GSDMD-N in both in vivo and in vitro models of UC (Jie et al., 2021). Similarly, Xuanbi Yuyang decoction has been reported to ameliorate DSS-induced UC by inhibiting pyroptosis through blockade of IL-17 pathway activation (Huang X. et al., 2024). Kui Jie Tong (KJT) is recognized for its ability to restore body weight and colon length in DSS-induced rat models of UC. KJT significantly reduces Disease Activity Index (DAI) scores, alleviates colon damage, and inhibits key components of the NEK7-NLRP3/caspase-1 inflammatory pathway while also modulating intestinal microbiota. These findings underscore KJT’s potential to induce remission in UC (Xue et al., 2022).
The Shen-ling-bai-zhu-san (SLBZS), referenced in the “Tai Ping Hui Min He Ji Ju Fang” has shown efficacy against various gastrointestinal disorders. Recent studies reveal that SLBZS reduces pro-inflammatory factor production, regulates MAPK and NF-κB signaling pathways, and inhibits pyroptosis signaling. Furthermore, SLBZS restores the expression of colonic tight junction proteins, thereby preserving the integrity of the colonic mucosal barrier (Chao et al., 2020). Huangqin decoction has demonstrated the ability to inhibit pyroptosis in UC cells, likely through the NLRP3/caspase-1 signaling pathway (Wu et al., 2021). The Shenlingbaizhu formula (SLBZ) also effectively alleviates DSS-induced UC by improving pathological symptoms, modulating gut microbiota and metabolic profiles, and inhibiting pro-inflammatory macrophage activation (Yu et al., 2022b). Tou Nong powder (TNP) has been shown to ameliorate UC by improving the DAI, reversing colonic weight loss, and alleviating histopathological injury. TNP treatment decreases levels of inflammatory markers, including TNF-α, diamine oxidase, ICAM-1, and endotoxin, while inhibiting the NF-κB/NLRP3/Caspase-1/GSDMD signaling pathway (Ye et al., 2023).
Huazhuojiedu decoction (HZJDD) has been clinically validated for UC treatment, with research indicating its role in regulating oxidative stress and downregulating key inflammatory markers such as CRP, TNF-α, IL-6, LPS, IL-1β, and IL-18. HZJDD also inhibits the activation of the NLRP3/caspase-1 signaling pathway, mitigating cellular death and enhancing therapeutic effects (Jia et al., 2022). The Xiao-Jian-Zhong formula (XJZ) treatment has shown promise in restoring gut microbial diversity and abundance, inhibiting pathogenic microbes, and enhancing linoleic acid metabolism and cytochrome P450 activity. In vitro experiments suggest that XJZ inhibits caspase-1-dependent pyroptosis, increases PPAR-γ expression, and promotes macrophage activation (Yu et al., 2022a). Yu Shi An Chang Fang (YST) has been reported to reduce levels of NLRP3, ASC, and cleaved caspase-1, while increasing the expression of tight junction proteins in colonic tissues. This suggests that YST ameliorates TNBS-induced UC by diminishing inflammatory responses and enhancing intestinal barrier function (Ye and Lai, 2021). Shao Yao Decoction (SYD), with historical significance dating back to the Jin-Yuan period, has demonstrated efficacy in UC treatment. SYD appears to protect against UC potentially by inhibiting the MKP1/NF-κB/NLRP3 pathway, thus alleviating cellular pyroptosis (Wei et al., 2021).
In addition to traditional compounded preparations, various herbal extracts have garnered considerable attention for their therapeutic potential in treating UC. Among these, Gegen-Qinlian decoction, which prominently features Puerariae Radix, has been utilized to alleviate intestinal pain and inflammation. Recent studies indicate that Puerariae Radix may ameliorate pyroptosis in DSS-induced UC models, exerting anti-inflammatory effects through the modulation of the NLRP3/caspase-1 signaling pathway (Ga et al., 2024). Similarly, a combination of Polygonum Hydropiper L. and Coptis Chinensis (PH-CC) has been demonstrated to inhibit pyroptosis-associated proteins and downregulate pro-inflammatory cytokines, including IL-18 and IL-1β. These findings support the notion that PH-CC may improve UC symptoms by regulating the NLRP3/caspase-1 pathway, underscoring its potential as a therapeutic agent for inflammatory bowel diseases (Zhu et al., 2024). Additionally, extracts of Astragalus mongholicus Bunge exhibit a spectrum of pharmacological effects, including anti-inflammatory, anticancer, and immunomodulatory properties. Studies reveal that these extracts can alleviate UC in DSS-induced mice by enhancing PLCB2 expression and inhibiting colonic epithelial cell pyroptosis, resulting in reduced inflammation and improved clinical and histopathological outcomes (Shen et al., 2024). Forsythia suspensa extract has also shown promise, significantly alleviating DSS-induced UC in mice by enhancing antioxidant activity and inhibiting pyroptosis through the Nrf2-NLRP3 signaling pathway, while concurrently improving metabolic function (Chao et al., 2022). Portulaca oleracea L., an edible and medicinal plant, has been recognized for its potential in treating gastrointestinal disorders. Comparative studies indicate that P. oleracea juice (PJ) contains a higher concentration of bioactive compounds and demonstrates a greater number of overlapping targets with UC compared to Portulaca oleracea extract (POE). In mouse models of UC, both POE and PJ were effective in reducing DAI scores and inflammatory cell infiltration; however, PJ exhibited superior efficacy. Furthermore, PJ inhibited cellular pyroptosis by downregulating the expression of NLRP3 inflammasome components and helped restore intestinal barrier function by upregulating tight junction proteins (Fan et al., 2023). In one study, polysaccharides derived from garlic (PSG) significantly alleviated inflammatory bowel disease and secondary liver injury induced by a drug delivery system (DDS) in mice. This effect was achieved by reducing colonic inflammation and enhancing intestinal barrier integrity. Mechanistically, PSG was found to suppress key inflammatory markers, including IL-1β, IL-18, and TLR4, alongside pyroptosis-related proteins such as NLRP3, GSDMD, and caspase-1, while also mitigating oxidative damage in liver tissues. These findings highlight the potential of PSG as a viable treatment for inflammatory bowel disease and secondary liver injury in humans (Zhan et al., 2022b). Overall, these studies collectively highlight the therapeutic promise of TCM, including compounds and extracts, in controlling UC by regulating the pathways associated with pyroptosis, which warrants further research into its mechanism of action and clinical application. The more detailed information of TCM formulas, extracts and other targeted pyroptosis treatments for UC are shown in Table 2.
The therapeutic spectrum for treatment of UC has expanded dramatically over the past few decades, with natural products emerging as potential adjunctive or alternative therapies to conventional therapies (Zheng et al., 2022; Xue et al., 2023). A large number of clinical trials have confirmed the efficacy of natural products in improving the symptoms of UC, which are mainly manifested in enhancing intestinal barrier function, reducing inflammatory response and improving the overall quality of life of patients (Guo et al., 2017; Zeng et al., 2022). For instance, SLBZ and Shaoyao decoction have been approved by the State Medical Products Administration in China for the treatment of UC and have shown promising therapeutic effects (Zhao Z. H. et al., 2024). In addition, other TCM compounds such as Curcumin have also been shown in clinical studies to restore the balance of intestinal microbiota and regulate NF-κB signaling in patients (Wang et al., 2018; Lin et al., 2022). When comparing natural products/TCM with conventional therapies, evidence suggests that while aminosalicylates, corticosteroids, and biologics offer robust short-term efficacy, their long-term use is limited by immunosuppression risks and relapse rates (Liu et al., 2022). The integration of natural products into treatment regimens could potentially mitigate some of these adverse effects while enhancing therapeutic outcomes. For example, a retrospective multicenter cohort study involving 88 patients with active UC (50% previously exposed to biologics/small molecule agents) demonstrated that the Curcumin-QingDai combination effectively induced clinical remission (46.5%) and biomarker response (median fecal calprotectin decreased from 1,000 μg/g to 75 μg/g), with no significant safety concerns observed. In conclusion, the current study suggests that the natural products/TCM preparations including SLBZ, Shaoyao decoction, and curcumin have good therapeutic potential in treating UC. Nevertheless, there is a need for further clarification of standardized protocols for the use of these natural products and TCM, optimization of dosage strategies and elucidation of their long-term therapeutic efficacy in different UC patient populations in the future.
Natural products have shown significant potential in treating UC, but still face many challenges in their clinical application. Key issues include bioavailability, standardisation and potential interactions with conventional drugs. (1) Firstly, bioavailability is one of the most critical issues because many natural compounds (e.g., Quercetin) are characterized by poor absorption and rapid metabolism, which may reduce their therapeutic efficacy considerably. Whereas, current reports show that strategies such as nanoparticle formulations and liposomal drug delivery show great promise for improving the bioavailability of Quercetin (Tomou et al., 2023). Therefore, the potential for clinical development of natural products can be greatly enhanced by strategies such as nanoparticle formulation and liposomal drug delivery. (2) Secondly, the standardization and quality control of natural products are also challenges that hinder their further application in the field of UC. The content or clinical efficacy of these natural products varies greatly depending on the geographical location of the plant from which they are derived and the processing method of the part of the plant where they are used. To address this, establishing stringent quality control measures and standardized extraction protocols is vital for ensuring the safety and efficacy of natural products in UC treatment. (3) Moreover, the potential for adverse effects and drug interactions must be carefully considered. While often perceived as safe, natural products can cause adverse reactions or interact with prescribed medications, particularly through modulation of cytochrome P450 enzymes (Jackson et al., 2018). In short, while natural products offer valuable therapeutic options for UC, challenges related to bioavailability, standardization and drug interactions must be addressed for safe and effective clinical application.
Natural products present a promising approach for treating UC, offering diverse bioactive compounds with anti-inflammatory and cytoprotective properties. For instance, flavonoids, polyphenols, and terpenoids have demonstrated efficacy in attenuating inflammatory responses through the inhibition of pyroptotic signaling cascades, thereby reducing mucosal damage and fostering intestinal healing (Wang T. et al., 2024). As the understanding of pyroptosis and its implications in UC deepens, the potential for natural products to serve as adjuncts or even primary treatments becomes increasingly evident. In this review, we find that specific phytochemical subclasses demonstrate multi-target interventions against UC-associated pyroptosis (Figure 4B). Notably, selected flavonoids (e.g., Phloretin, Naringin, and Rutin) and alkaloids (e.g., Oxymatrine and Evodiamine) suppress NLRP3 inflammasome activation mediated pyroptosis signaling, while steroids like β-sitosterol inhibit Caspase-1/GSDMD-mediated pyroptotic signaling. Concurrently, certain phenylpropanoids (e.g., Schisandrin B) and terpenoids (e.g., Brusatol) enhance redox homeostasis by scavenging ROS via Nrf2 pathways and activating AMPK-dependent stress adaptation, indirectly attenuating oxidative stress-triggered pyroptosis. Gut barrier restoration is achieved through SCFAs-GPR43/TLR4 axis modulation by terpenoids (e.g., P. chinensis saponins) which rebalance microbiota and fortify epithelial integrity to prevent pyroptosis-inducing pathogen infiltration. Cross-regulatory mechanisms further amplify anti-pyroptotic effects via dual inhibition of NF-κB/MAPKs cascades (e.g., Platycodon grandiflorum root) and PPARγ/FXR-mediated transcriptional control (e.g., Nigakinone, Phloretin), creating synergistic networks that disrupt chronic inflammatory amplification loops. All in all, the structural diversity of phytochemicals enables polypharmacological targeting of these interconnected axes, highlighting their therapeutic superiority over single-pathway agents in addressing UC’s multifactorial pyroptosis pathology.
Figure 4. Categorization and summary of natural products types, functions, core targets and pathways by targeting pyroptosis for UC treatment. (A) Potential regulatory targets and mechanisms of natural products targeting pyroptosis for the treatment of UC. (B) Integrative network of compound-target/pathway interactions across inflammation, oxidative stress, and gut barrier repair.
In the future, it is need to prioritize continued exploration into the mechanisms by which natural products influence pyroptosis and related inflammatory pathways. Research should focus on elucidating the molecular interactions of these natural compounds with the key components in cell pyroptosis. Additionally, it is need to develop standardized formulations or extracts to ensure consistency in therapeutic efficacy and safety in clinical. Alongside basic research, clinical investigations are equally vital for translating these findings into practical applications. Rigorous clinical trials are necessary to evaluate the effectiveness and safety of interventions using natural products in patients with UC, confirming their role in clinical management. Moreover, studying the pharmacokinetics and bioavailability of these compounds will be crucial for understanding their therapeutic windows and potential interactions with existing drugs. Furthermore, interdisciplinary approaches that combine insights from microbiology (Shen et al., 2018; Kang et al., 2023), immunology (Long et al., 2024b), and pharmacology (Wang Z. et al., 2024) will enhance our understanding of the complex interactions between pyroptosis, inflammation, and the gut microbiome in UC. The gut microbiome plays a pivotal role in modulating immune responses, and its dysbiosis has been implicated in the pathogenesis of UC. Understanding how pyroptosis influences microbial composition and function, as well as how natural products can restore microbial balance, could lead to innovative strategies for UC management.
Emerging technologies, such as metagenomics and metabolomics, offer powerful tools to explore these interactions in greater detail (Li M. et al., 2022; Schirmer et al., 2024). By characterizing the microbial communities in UC patients and assessing the impact of natural products on these communities, it is possible to inform personalized medicine for UC patients. Moreover, the role of lifestyle factors (Lo et al., 2021; Lopes et al., 2022), such as diet and physical activity, in modulating pyroptosis and inflammation in UC deserves further investigation. Dietary interventions that incorporate natural products rich in anti-inflammatory constituents may synergize with pharmacological therapies to enhance patient outcomes. A holistic approach that combines dietary modifications with natural product supplementation could provide a comprehensive strategy for managing UC and improving quality of life for patients.
In conclusion, the significance of pyroptosis in UC cannot be overstated. Its role in driving inflammation and tissue damage presents both challenges and opportunities for therapeutic intervention. Natural products represent a promising frontier in the exploration of new treatments for UC, with the potential to modulate pyroptosis and restore intestinal homeostasis. Continued research into the mechanisms underlying these interactions and the clinical applicability of natural products will be vital in shaping the future landscape of UC management. By embracing a multifaceted approach that integrates basic and clinical research, we can unlock the full potential of natural products as therapeutic agents in the fight against UC.
XL: Investigation, Methodology, Visualization, Writing – original draft. YS: Data curation, Visualization, Writing – original draft. ZZ: Data curation, Methodology, Writing – original draft. ZS: Data curation, Methodology, Writing – original draft. SW: Data curation, Methodology, Visualization, Writing – review and editing. EX: Funding acquisition, Resources, Supervision, Writing – review and editing.
The author(s) declare that financial support was received for the research and/or publication of this article. This study was supported by the “Zhang Zhongjing Inheritance and Innovation Special Project” (GZY-KJS-2022-044-1) from the Science and Technology Department of the National Administration of Traditional Chinese Medicine, the Key Project of Henan Province Science and Technology Research and Development Program Joint Fund (222301420021), and the Natural Science Foundation of Sichuan Province (2024NSFSC2115).
We would like to thank BioRender (http://biorender.com/) for providing the tools used to create our Figures 1, 2.
The authors declare that the research was conducted in the absence of any commercial or financial relationships that could be construed as a potential conflict of interest.
The authors declare that no Generative AI was used in the creation of this manuscript.
All claims expressed in this article are solely those of the authors and do not necessarily represent those of their affiliated organizations, or those of the publisher, the editors and the reviewers. Any product that may be evaluated in this article, or claim that may be made by its manufacturer, is not guaranteed or endorsed by the publisher.
Abdulhakeem Mansour Alhasbary, A., Hashimah Ahamed Hassain Malim, N., and Zuraidah Mohamad Zobir, S. (2025). Exploring natural products potential: a similarity-based target prediction tool for natural products. Comput. Biol. Med. 184, 109351. doi:10.1016/j.compbiomed.2024.109351
Alqudah, A., Qnais, E., Gammoh, O., Bseiso, Y., Wedyan, M., Alqudah, M., et al. (2024). Exploring the therapeutic potential of Anastatica hierochuntica essential oil in DSS-induced colitis. Inflammopharmacology 32, 2035–2048. doi:10.1007/s10787-024-01449-4
Atabaki, R., Khaleghzadeh-Ahangar, H., Esmaeili, N., and Mohseni-Moghaddam, P. (2023). Role of pyroptosis, a pro-inflammatory programmed cell death, in epilepsy. Cell Mol. Neurobiol. 43, 1049–1059. doi:10.1007/s10571-022-01250-3
Bian, W., Wei, L., and Wang, K. (2024a). Carthamin yellow alleviates dextran sodium sulfate-induced ulcerative colitis by repairing the intestinal barrier and activating the Nrf2/GPX4 axis. Int. Immunopharmacol. 141, 113020. doi:10.1016/j.intimp.2024.113020
Bian, W., Wei, L., and Wang, K. (2024b). Carthamin yellow alleviates dextran sodium sulfate-induced ulcerative colitis by repairing the intestinal barrier and activating the Nrf2/GPX4 axis. Int. Immunopharmacol. 141, 113020. doi:10.1016/j.intimp.2024.113020
Bian, Z., Qin, Y., Li, L., Su, L., Fei, C., Li, Y., et al. (2022). Schisandra chinensis (Turcz.) Baill. Protects against DSS-induced colitis in mice: involvement of TLR4/NF-κB/NLRP3 inflammasome pathway and gut microbiota. J. Ethnopharmacol. 298, 115570. doi:10.1016/j.jep.2022.115570
Broz, P., Pelegrín, P., and Shao, F. (2020). The gasdermins, a protein family executing cell death and inflammation. Nat. Rev. Immunol. 20, 143–157. doi:10.1038/s41577-019-0228-2
Cai, J., Liu, J., Fan, P., Dong, X., Zhu, K., Liu, X., et al. (2021). Dioscin prevents DSS-induced colitis in mice with enhancing intestinal barrier function and reducing colon inflammation. Int. Immunopharmacol. 99, 108015. doi:10.1016/j.intimp.2021.108015
Cao, D., Xi, R., Li, H., Zhang, Z., Shi, X., Li, S., et al. (2024). Discovery of a covalent inhibitor of pro-caspase-1 zymogen blocking NLRP3 inflammasome activation and pyroptosis. J. Med. Chem. 67, 15873–15891. doi:10.1021/acs.jmedchem.4c01558
Cao, H., Liu, J., Shen, P., Cai, J., Han, Y., Zhu, K., et al. (2018). Protective effect of Naringin on DSS-induced ulcerative colitis in mice. J. Agric. Food Chem. 66, 13133–13140. doi:10.1021/acs.jafc.8b03942
Chao, L., Li, Z., Zhou, J., Chen, W., Li, Y., Lv, W., et al. (2020). Shen-Ling-Bai-Zhu-San improves dextran sodium sulfate-induced colitis by inhibiting caspase-1/caspase-11-mediated pyroptosis. Front. Pharmacol. 11, 814. doi:10.3389/fphar.2020.00814
Chao, L., Lin, J., Zhou, J., Du, H., Chen, X., Liu, M., et al. (2022). Polyphenol rich forsythia suspensa extract alleviates DSS-induced ulcerative colitis in mice through the nrf2-NLRP3 pathway. Antioxidants (Basel) 11, 475. doi:10.3390/antiox11030475
Chen, B., Dong, X., Zhang, J. L., Sun, X., Zhou, L., Zhao, K., et al. (2024a). Natural compounds target programmed cell death (PCD) signaling mechanism to treat ulcerative colitis: a review. Front. Pharmacol. 15, 1333657. doi:10.3389/fphar.2024.1333657
Chen, J., Jiang, F., Xu, N., Dong, G., Jiang, J., Wang, M., et al. (2024b). Anthocyanin extracted from purple sweet potato alleviates dextran sulfate sodium-induced colitis in mice by suppressing pyroptosis and altering intestinal flora structure. J. Med. Food 27, 110–122. doi:10.1089/jmf.2023.K.0247
Chen, J., Lu, P., Liu, J., Yang, L., Li, Y., Chen, Y., et al. (2023a). 20(S)- Protopanaxadiol saponins isolated from Panax notoginseng target the binding of HMGB1 to TLR4 against inflammation in experimental ulcerative colitis. Phytother. Res. 37, 4690–4705. doi:10.1002/ptr.7938
Chen, J., Pan, M., Wang, J., Zhang, M., Feng, M., Chai, X., et al. (2023b). Hydroxysafflor yellow A protects against colitis in mice by suppressing pyroptosis via inhibiting HK1/NLRP3/GSDMD and modulating gut microbiota. Toxicol. Appl. Pharmacol. 467, 116494. doi:10.1016/j.taap.2023.116494
Chen, L., Liu, D., Mao, M., Liu, W., Wang, Y., Liang, Y., et al. (2022). Betaine ameliorates acute sever ulcerative colitis by inhibiting oxidative stress induced inflammatory pyroptosis. Mol. Nutr. Food Res. 66, e2200341. doi:10.1002/mnfr.202200341
Chen, M., Feng, Y., Luo, D., Zhang, C., Zhou, J., Dai, H., et al. (2024c). Wuwei Kushen Changrong capsule alleviates DSS-induced colitis in mice via inhibition of NLRP3 inflammasome and STAT3 pathway. Front. Pharmacol. 15, 1423012. doi:10.3389/fphar.2024.1423012
Chen, Q., Duan, X., Fan, H., Xu, M., Tang, Q., Zhang, L., et al. (2017). Oxymatrine protects against DSS-induced colitis via inhibiting the PI3K/AKT signaling pathway. Int. Immunopharmacol. 53, 149–157. doi:10.1016/j.intimp.2017.10.025
Chen, Q. L., Yin, H. R., He, Q. Y., and Wang, Y. (2021). Targeting the NLRP3 inflammasome as new therapeutic avenue for inflammatory bowel disease. Biomed. Pharmacother. 138, 111442. doi:10.1016/j.biopha.2021.111442
Cheng, J., Ma, X., Zhang, H., Wu, X., Li, M., Ai, G., et al. (2022). 8-Oxypalmatine, a novel oxidative metabolite of palmatine, exhibits superior anti-colitis effect via regulating Nrf2 and NLRP3 inflammasome. Biomed. Pharmacother. 153, 113335. doi:10.1016/j.biopha.2022.113335
Coll, R. C., Schroder, K., and Pelegrín, P. (2022). NLRP3 and pyroptosis blockers for treating inflammatory diseases. Trends Pharmacol. Sci. 43, 653–668. doi:10.1016/j.tips.2022.04.003
Cui, L. J., Yuan, W., Chen, F. Y., Wang, Y. X., Li, Q. M., Lin, C., et al. (2022). Pectic polysaccharides ameliorate the pathology of ulcerative colitis in mice by reducing pyroptosis. Ann. Transl. Med. 10, 347. doi:10.21037/atm-22-877
Dai, Y., Lu, Q., Li, P., Zhu, J., Jiang, J., Zhao, T., et al. (2023a). Xianglian Pill attenuates ulcerative colitis through TLR4/MyD88/NF-κB signaling pathway. J. Ethnopharmacol. 300, 115690. doi:10.1016/j.jep.2022.115690
Dai, Z., Liu, W. C., Chen, X. Y., Wang, X., Li, J. L., and Zhang, X. (2023b). Gasdermin D-mediated pyroptosis: mechanisms, diseases, and inhibitors. Front. Immunol. 14, 1178662. doi:10.3389/fimmu.2023.1178662
Darb Emamie, A., Rajabpour, M., Ghanavati, R., Asadolahi, P., Farzi, S., Sobouti, B., et al. (2021). The effects of probiotics, prebiotics and synbiotics on the reduction of IBD complications, a periodic review during 2009-2020. J. Appl. Microbiol. 130, 1823–1838. doi:10.1111/jam.14907
Digby-Bell, J. L., Atreya, R., Monteleone, G., and Powell, N. (2020). Interrogating host immunity to predict treatment response in inflammatory bowel disease. Nat. Rev. Gastroenterol. Hepatol. 17, 9–20. doi:10.1038/s41575-019-0228-5
Ekiert, H. M., and Szopa, A. (2020). Biological activities of natural products. Molecules 25, 5769. doi:10.3390/molecules25235769
Fan, X., Fan, Z., Yang, Z., Huang, T., Tong, Y., Yang, D., et al. (2022). Flavonoids-natural gifts to promote health and longevity. Int. J. Mol. Sci. 23, 2176. doi:10.3390/ijms23042176
Fan, Y. M., Wei, Y. Y., Wang, H. R., Yu, G., Zhang, Y. N., and Hao, Z. (2023). Inhibitory effect of Portulaca oleracea L. aqueous extract and juice on NLRP3 inflammasome activation in an ulcerative colitis mouse model. Environ. Sci. Pollut. Res. Int. 30, 86380–86394. doi:10.1007/s11356-023-28365-4
Ga, Y., Wei, Y., Zhao, Q., Fan, Y., Zhang, Y., Zhang, Z., et al. (2024). Puerariae radix protects against ulcerative colitis in mice by inhibiting NLRP3 inflammasome activation. Food Sci. Hum. Wellness 13, 2266–2276. doi:10.26599/FSHW.2022.9250189
Gajendran, M., Loganathan, P., Jimenez, G., Catinella, A. P., Ng, N., Umapathy, C., et al. (2019). A comprehensive review and update on ulcerative colitis. Dis. Mon. 65, 100851. doi:10.1016/j.disamonth.2019.02.004
Gao, B. B., Wang, L., Li, L. Z., Fei, Z. Q., Wang, Y. Y., Zou, X. M., et al. (2024). Beneficial effects of oxymatrine from Sophora flavescens on alleviating Ulcerative colitis by improving inflammation and ferroptosis. J. Ethnopharmacol. 332, 118385. doi:10.1016/j.jep.2024.118385
Gao, X. J., Tang, B., Liang, H. H., Yi, L., and Wei, Z. G. (2019). The protective effect of nigeglanine on dextran sulfate sodium-induced experimental colitis in mice and Caco-2 cells. J. Cell Physiol. 234, 23398–23408. doi:10.1002/jcp.28909
Gong, Z., Zhao, S., Zhou, J., Yan, J., Wang, L., Du, X., et al. (2018). Curcumin alleviates DSS-induced colitis via inhibiting NLRP3 inflammsome activation and IL-1β production. Mol. Immunol. 104, 11–19. doi:10.1016/j.molimm.2018.09.004
Gros, B., and Kaplan, G. G. (2023). Ulcerative colitis in adults: a review. Jama 330, 951–965. doi:10.1001/jama.2023.15389
Gu, D., Wang, H., Yan, M., Li, Y., Yang, S., Shi, D., et al. (2023). Echinacea purpurea (L.) Moench extract suppresses inflammation by inhibition of C3a/C3aR signaling pathway in TNBS-induced ulcerative colitis rats. J. Ethnopharmacol. 307, 116221. doi:10.1016/j.jep.2023.116221
Guo, B. J., Bian, Z. X., Qiu, H. C., Wang, Y. T., and Wang, Y. (2017). Biological and clinical implications of herbal medicine and natural products for the treatment of inflammatory bowel disease. Ann. N. Y. Acad. Sci. 1401, 37–48. doi:10.1111/nyas.13414
He, S., Zhang, T., Wang, Y. Y., Yuan, W., Li, L., Li, J., et al. (2024). Isofraxidin attenuates dextran sulfate sodium-induced ulcerative colitis through inhibiting pyroptosis by upregulating Nrf2 and reducing reactive oxidative species. Int. Immunopharmacol. 128, 111570. doi:10.1016/j.intimp.2024.111570
Hu, J., Huang, H., Che, Y., Ding, C., Zhang, L., Wang, Y., et al. (2021). Qingchang Huashi Formula attenuates DSS-induced colitis in mice by restoring gut microbiota-metabolism homeostasis and goblet cell function. J. Ethnopharmacol. 266, 113394. doi:10.1016/j.jep.2020.113394
Hu, Y., Tang, J., Xie, Y., Xu, W., Zhu, W., Xia, L., et al. (2024). Gegen Qinlian decoction ameliorates TNBS-induced ulcerative colitis by regulating Th2/Th1 and Tregs/Th17 cells balance, inhibiting NLRP3 inflammasome activation and reshaping gut microbiota. J. Ethnopharmacol. 328, 117956. doi:10.1016/j.jep.2024.117956
Huang, S., Tao, R., Zhou, J., Qian, L., and Wu, J. (2022). Trans-10-Hydroxy-2-Decenoic acid alleviates dextran sulfate sodium-induced colitis in mice via regulating the inflammasome-mediated pyroptotic pathway and enhancing colonic barrier function. Mol. Nutr. Food Res. 66, e2100821. doi:10.1002/mnfr.202100821
Huang, X., Li, L., Zheng, C., Li, J., Chen, G., and Chen, Y. (2024a). Xuanbi Yuyang decoction ameliorates DSS-induced colitis by inhibiting pyroptosis via blocking of IL-17 pathway activation. J. Inflamm. Res. 17, 5235–5249. doi:10.2147/jir.s472812
Huang, Y., Chen, X., Liu, X., Lin, C., and Wang, Y. (2024b). The coumarin component isofraxidin targets the G-protein-coupled receptor S1PR1 to modulate IL-17 signaling and alleviate ulcerative colitis. Int. Immunopharmacol. 131, 111814. doi:10.1016/j.intimp.2024.111814
Jackson, K. D., Durandis, R., and Vergne, M. J. (2018). Role of cytochrome P450 enzymes in the metabolic activation of tyrosine kinase inhibitors. Int. J. Mol. Sci. 19, 2367. doi:10.3390/ijms19082367
Jia, X., Li, Z., Guo, Y., Ma, H., Wang, J., Xue, Y., et al. (2022). The potential mechanism of huazhuojiedu decoction in the treatment of ulcerative colitis based on network pharmacology and experimental validation. Front. Pharmacol. 13, 1033874. doi:10.3389/fphar.2022.1033874
Jiang, M., Qi, L., Li, L., and Li, Y. (2020). The caspase-3/GSDME signal pathway as a switch between apoptosis and pyroptosis in cancer. Cell Death Discov. 6, 112. doi:10.1038/s41420-020-00349-0
Jie, F., Xiao, S., Qiao, Y., You, Y., Feng, Y., Long, Y., et al. (2021). Kuijieling decoction suppresses NLRP3-Mediated pyroptosis to alleviate inflammation and experimental colitis in vivo and in vitro. J. Ethnopharmacol. 264, 113243. doi:10.1016/j.jep.2020.113243
Kang, D. Y., Park, J. L., Yeo, M. K., Kang, S. B., Kim, J. M., Kim, J. S., et al. (2023). Diagnosis of Crohn's disease and ulcerative colitis using the microbiome. BMC Microbiol. 23, 336. doi:10.1186/s12866-023-03084-5
Kazmi, I., Altamimi, A. S. A., Afzal, M., Majami, A. A., Abbasi, F. A., Almalki, W. H., et al. (2024). Non-coding RNAs: emerging biomarkers and therapeutic targets in ulcerative colitis. Pathol. Res. Pract. 253, 155037. doi:10.1016/j.prp.2023.155037
Ketelut-Carneiro, N., and Fitzgerald, K. A. (2022). Apoptosis, pyroptosis, and necroptosis-oh my! The many ways a cell can die. J. Mol. Biol. 434, 167378. doi:10.1016/j.jmb.2021.167378
Kim, K. Y., Kang, Y. M., Lee, A., Kim, Y. J., Kim, K. H., and Hwang, Y. H. (2024). Hydroethanolic extract of lepidium apetalum willdenow alleviates dextran sulfate sodium-induced colitis by enhancing intestinal barrier integrity and inhibiting oxidative stress and inflammasome activation. Antioxidants (Basel) 13, 795. doi:10.3390/antiox13070795
Le Berre, C., Honap, S., and Peyrin-Biroulet, L. (2023). Ulcerative colitis. Lancet 402, 571–584. doi:10.1016/s0140-6736(23)00966-2
Leblanc, J. F., Segal, J. P., De Campos Braz, L. M., and Hart, A. L. (2021). The microbiome as a therapy in pouchitis and ulcerative colitis. Nutrients 13, 1780. doi:10.3390/nu13061780
Lee, Y. J., Lee, Y. M., Lee, C. K., Jung, J. K., Han, S. B., and Hong, J. T. (2011). Therapeutic applications of compounds in the Magnolia family. Pharmacol. Ther. 130, 157–176. doi:10.1016/j.pharmthera.2011.01.010
Li, C., Deng, L., Pu, M., Ye, X., and Lu, Q. (2024a). Coptisine alleviates colitis through modulating gut microbiota and inhibiting TXNIP/NLRP3 inflammasome. J. Ethnopharmacol. 335, 118680. doi:10.1016/j.jep.2024.118680
Li, C., Wang, M., Sui, J., Zhou, Y., and Chen, W. (2021). Protective mechanisms of Agrimonia pilosa Ledeb in dextran sodium sulfate-induced colitis as determined by a network pharmacology approach. Acta Biochim. Biophys. Sin. (Shanghai) 53, 1342–1353. doi:10.1093/abbs/gmab116
Li, D., Li, Y., Jiang, X., Liu, W., and Zhao, Q. (2022a). Evodiamine: a privileged structure with broad-ranging biological activities. Mini Rev. Med. Chem. 22, 2680–2701. doi:10.2174/1389557522666220404090835
Li, H., Feng, J., Liu, C., Hou, S., Meng, J., Liu, J. Y., et al. (2024b). Polysaccharides from an edible mushroom, Hericium erinaceus, alleviate ulcerative colitis in mice by inhibiting the NLRP3 inflammasomes and reestablish intestinal homeostasis. Int. J. Biol. Macromol. 267, 131251. doi:10.1016/j.ijbiomac.2024.131251
Li, J., Wu, H., Zhou, J., Jiang, R., Zhuo, Z., Yang, Q., et al. (2024c). Ruscogenin attenuates ulcerative colitis in mice by inhibiting caspase-1-dependent pyroptosis via the TLR4/NF-κB signaling pathway. Biomedicines 12, 989. doi:10.3390/biomedicines12050989
Li, M., Yang, L., Mu, C., Sun, Y., Gu, Y., Chen, D., et al. (2022b). Gut microbial metabolome in inflammatory bowel disease: from association to therapeutic perspectives. Comput. Struct. Biotechnol. J. 20, 2402–2414. doi:10.1016/j.csbj.2022.03.038
Li, R., Chen, C., Liu, B., Shi, W., Shimizu, K., and Zhang, C. (2022c). Bryodulcosigenin a natural cucurbitane-type triterpenoid attenuates dextran sulfate sodium (DSS)-induced colitis in mice. Phytomedicine 94, 153814. doi:10.1016/j.phymed.2021.153814
Li, W. Y., Liu, J. Y., Wang, Z. X., Wang, K. Y., Huang, C. X., He, W., et al. (2024d). Sinapic acid attenuates chronic DSS-induced intestinal fibrosis in C57bl/6J mice by modulating NLRP3 inflammasome activation and the autophagy pathway. ACS Omega 9, 1230–1241. doi:10.1021/acsomega.3c07474
Li, X., Wu, X., Wang, Q., Xu, W., Zhao, Q., Xu, N., et al. (2022d). Sanguinarine ameliorates DSS induced ulcerative colitis by inhibiting NLRP3 inflammasome activation and modulating intestinal microbiota in C57BL/6 mice. Phytomedicine 104, 154321. doi:10.1016/j.phymed.2022.154321
Li, Y. X., Lv, L., Li, S. L., and Qian, H. H. (2024e). Gentianine alleviates dextran sulfate sodium-induced ulcerative colitis via inhibition of TLR4/NLRP3-mediated pyroptosis. Int. Immunopharmacol. 126, 111214. doi:10.1016/j.intimp.2023.111214
Li, Z., Song, Y., Xu, W., Chen, J., Zhou, R., Yang, M., et al. (2023). Pulsatilla chinensis saponins improve SCFAs regulating GPR43-NLRP3 signaling pathway in the treatment of ulcerative colitis. J. Ethnopharmacol. 308, 116215. doi:10.1016/j.jep.2023.116215
Liang, H., Cheng, R., Wang, J., Xie, H., Li, R., Shimizu, K., et al. (2021). Mogrol, an aglycone of mogrosides, attenuates ulcerative colitis by promoting AMPK activation. Phytomedicine 81, 153427. doi:10.1016/j.phymed.2020.153427
Liang, J., Chen, S., Chen, J., Lin, J., Xiong, Q., Yang, Y., et al. (2018). Therapeutic roles of polysaccharides from Dendrobium Officinaleon colitis and its underlying mechanisms. Carbohydr. Polym. 185, 159–168. doi:10.1016/j.carbpol.2018.01.013
Lin, Y., Liu, H., Bu, L., Chen, C., and Ye, X. (2022). Review of the effects and mechanism of curcumin in the treatment of inflammatory bowel disease. Front. Pharmacol. 13, 908077. doi:10.3389/fphar.2022.908077
Lin, Z., Chen, Q., and Ruan, H.-B. (2024a). To die or not to die: Gasdermins in intestinal health and disease. Seminars Immunol. 71, 101865. doi:10.1016/j.smim.2024.101865
Lin, Z., Li, L. Y., Chen, L., Jin, C., Li, Y., Yang, L., et al. (2024b). Lonicerin promotes wound healing in diabetic rats by enhancing blood vessel regeneration through Sirt1-mediated autophagy. Acta Pharmacol. Sin. 45, 815–830. doi:10.1038/s41401-023-01193-5
Liso, M., Verna, G., Cavalcanti, E., De Santis, S., Armentano, R., Tafaro, A., et al. (2022). Interleukin 1β blockade reduces intestinal inflammation in a murine model of tumor necrosis factor-independent ulcerative colitis. Cell Mol. Gastroenterol. Hepatol. 14, 151–171. doi:10.1016/j.jcmgh.2022.03.003
Liu, D., Tian, Q., Liu, K., Ren, F., Liu, G., Zhou, J., et al. (2023a). Ginsenoside Rg3 ameliorates DSS-induced colitis by inhibiting NLRP3 inflammasome activation and regulating microbial homeostasis. J. Agric. Food Chem. 71, 3472–3483. doi:10.1021/acs.jafc.2c07766
Liu, F., Wang, X., Cui, Y., Yin, Y., Qiu, D., Li, S., et al. (2021). Apple polyphenols extract (APE) alleviated dextran sulfate sodium induced acute ulcerative colitis and accompanying neuroinflammation via inhibition of apoptosis and pyroptosis. Foods 10, 2711. doi:10.3390/foods10112711
Liu, F., Yao, Y., Wang, Q., Zhang, F., Wang, M., Zhu, C., et al. (2023b). Nigakinone alleviates DSS-induced experimental colitis via regulating bile acid profile and FXR/NLRP3 signaling pathways. Phytother. Res. 37, 15–34. doi:10.1002/ptr.7588
Liu, F., Zhang, Q., Lin, C., Yao, Y., Wang, M., Liu, C., et al. (2020a). A comparative study on pharmacokinetics and tissue distribution of 5-hydroxy-4-methoxycanthin-6-one and its metabolite in normal and dextran sodium sulfate-induced colitis rats by HPLC-MS/MS. J. Pharm. Pharmacol. 72, 1761–1770. doi:10.1111/jphp.13285
Liu, H., Chen, R., Wen, S., Li, Q., Lai, X., Zhang, Z., et al. (2023c). Tea (Camellia sinensis) ameliorates DSS-induced colitis and liver injury by inhibiting TLR4/NF-κB/NLRP3 inflammasome in mice. Biomed. Pharmacother. 158, 114136. doi:10.1016/j.biopha.2022.114136
Liu, H., Wang, Z., Zheng, C., Zhou, L., Peng, S., Mcclements, D. J., et al. (2024). Alleviation of ulcerative colitis via curcumin-fortified orange juice: utilization of orange-derived extracellular vesicles as active nanocarrier. Food Biosci. 62, 105490. doi:10.1016/j.fbio.2024.105490
Liu, J., Cai, J., Fan, P., Zhang, N., and Cao, Y. (2019). The abilities of salidroside on ameliorating inflammation, skewing the imbalanced nucleotide oligomerization domain-like receptor family pyrin domain containing 3/autophagy, and maintaining intestinal barrier are profitable in colitis. Front. Pharmacol. 10, 1385. doi:10.3389/fphar.2019.01385
Liu, M., Wang, Z., Liu, X., Xiao, H., Liu, Y., Wang, J., et al. (2023d). Therapeutic effect of Yiyi Fuzi Baijiang formula on TNBS-induced ulcerative colitis via metabolism and Th17/Treg cell balance. J. Ethnopharmacol. 309, 116301. doi:10.1016/j.jep.2023.116301
Liu, Q., Zuo, R., Wang, K., Nong, F. F., Fu, Y. J., Huang, S. W., et al. (2020b). Oroxindin inhibits macrophage NLRP3 inflammasome activation in DSS-induced ulcerative colitis in mice via suppressing TXNIP-dependent NF-κB pathway. Acta Pharmacol. Sin. 41, 771–781. doi:10.1038/s41401-019-0335-4
Liu, X., Zhou, M., Dai, Z., Luo, S., Shi, Y., He, Z., et al. (2023e). Salidroside alleviates ulcerative colitis via inhibiting macrophage pyroptosis and repairing the dysbacteriosis-associated Th17/Treg imbalance. Phytother. Res. 37, 367–382. doi:10.1002/ptr.7636
Liu, Y., Li, B. G., Su, Y. H., Zhao, R. X., Song, P., Li, H., et al. (2022). Potential activity of traditional Chinese medicine against ulcerative colitis: a review. J. Ethnopharmacol. 289, 115084. doi:10.1016/j.jep.2022.115084
Lo, C. H., Khalili, H., Song, M., Lochhead, P., Burke, K. E., Richter, J. M., et al. (2021). Healthy lifestyle is associated with reduced mortality in patients with inflammatory bowel diseases. Clin. Gastroenterol. Hepatol. 19, 87–95.e4. doi:10.1016/j.cgh.2020.02.047
Long, D., Mao, C., Huang, Y., Xu, Y., and Zhu, Y. (2024a). Ferroptosis in ulcerative colitis: potential mechanisms and promising therapeutic targets. Biomed. Pharmacother. 175, 116722. doi:10.1016/j.biopha.2024.116722
Long, D., Mao, C., Xu, Y., and Zhu, Y. (2024b). The emerging role of neutrophil extracellular traps in ulcerative colitis. Front. Immunol. 15, 1425251. doi:10.3389/fimmu.2024.1425251
Lopes, E. W., Chan, S. S. M., Song, M., Ludvigsson, J. F., Håkansson, N., Lochhead, P., et al. (2022). Lifestyle factors for the prevention of inflammatory bowel disease. Gut 72, 1093, 1100. doi:10.1136/gutjnl-2022-328174
Luo, M., Huang, J. C., Yang, Z. Q., Wang, Y. S., Guo, B., and Yue, Z. P. (2020). Hydroxysafflor yellow A exerts beneficial effects by restoring hormone secretion and alleviating oxidative stress in polycystic ovary syndrome mice. Exp. Physiol. 105, 282–292. doi:10.1113/ep088147
Luo, X., Bao, X., Weng, X., Bai, X., Feng, Y., Huang, J., et al. (2022). The protective effect of quercetin on macrophage pyroptosis via TLR2/Myd88/NF-κB and ROS/AMPK pathway. Life Sci. 291, 120064. doi:10.1016/j.lfs.2021.120064
Lv, Q., Xing, Y., Liu, J., Dong, D., Liu, Y., Qiao, H., et al. (2021). Lonicerin targets EZH2 to alleviate ulcerative colitis by autophagy-mediated NLRP3 inflammasome inactivation. Acta Pharm. Sin. B 11, 2880–2899. doi:10.1016/j.apsb.2021.03.011
Lv, W., Jin, W., Lin, J., Wang, Z., Ma, Y., Zhang, W., et al. (2024). Forsythia suspensa polyphenols regulate macrophage M1 polarization to alleviate intestinal inflammation in mice. Phytomedicine 125, 155336. doi:10.1016/j.phymed.2024.155336
Lv, Y., Qiu, T., and Niu, L. (2023). Jaceosidin inhibits NLRP3-mediated pyroptosis by activating SIRT1/NRF2 and ameliorating intestinal epithelial cell injury. Trop. J. Pharm. Res. 22, 1773–1778. doi:10.4314/tjpr.v22i9.2
Ma, J., Zhang, J., Wang, Y., Huang, J., Yang, X., Ma, J., et al. (2023a). Modified Gegen Qinlian decoction ameliorates DSS-induced chronic colitis in mice by restoring the intestinal mucus barrier and inhibiting the activation of γδT17 cells. Phytomedicine 111, 154660. doi:10.1016/j.phymed.2023.154660
Ma, S., Yang, B., Du, Y., Lv, Y., Liu, J., Shi, Y., et al. (2023b). 1,8-cineole ameliorates colon injury by downregulating macrophage M1 polarization via inhibiting the HSP90-NLRP3-SGT1 complex. J. Pharm. Anal. 13, 984–998. doi:10.1016/j.jpha.2023.07.001
Mahmoud, T. N., El-Maadawy, W. H., Kandil, Z. A., Khalil, H., El-Fiky, N. M., and El Alfy, T. (2021). Canna x generalis L.H. Bailey rhizome extract ameliorates dextran sulfate sodium-induced colitis via modulating intestinal mucosal dysfunction, oxidative stress, inflammation, and TLR4/NF-ҡB and NLRP3 inflammasome pathways. J. Ethnopharmacol. 269, 113670. doi:10.1016/j.jep.2020.113670
Man, S. M., and Kanneganti, T. D. (2015). Regulation of inflammasome activation. Immunol. Rev. 265, 6–21. doi:10.1111/imr.12296
Miao, F., Shan, C., Ma, T., Geng, S., and Ning, D. (2021). Walnut oil alleviates DSS-induced colitis in mice by inhibiting NLRP3 inflammasome activation and regulating gut microbiota. Microb. Pathog. 154, 104866. doi:10.1016/j.micpath.2021.104866
Ning, L., Ye, N., Ye, B., Miao, Z., Cao, T., Lu, W., et al. (2023). Qingre Xingyu recipe exerts inhibiting effects on ulcerative colitis development by inhibiting TNFα/NLRP3/Caspase-1/IL-1β pathway and macrophage M1 polarization. Cell Death Discov. 9, 84. doi:10.1038/s41420-023-01361-w
Pan, H., Jian, Y., Wang, F., Yu, S., Guo, J., Kan, J., et al. (2022a). NLRP3 and gut microbiota homeostasis: progress in research. Cells 11, 3758. doi:10.3390/cells11233758
Pan, X., Wang, H., Zheng, Z., Huang, X., Yang, L., Liu, J., et al. (2022b). Pectic polysaccharide from Smilax China L. ameliorated ulcerative colitis by inhibiting the galectin-3/NLRP3 inflammasome pathway. Carbohydr. Polym. 277, 118864. doi:10.1016/j.carbpol.2021.118864
Park, S. Y., Hisham, Y., Shin, H. M., Yeom, S. C., and Kim, S. (2022). Interleukin-18 binding protein in immune regulation and autoimmune diseases. Biomedicines 10, 1750. doi:10.3390/biomedicines10071750
Peiró, C., Lorenzo, Ó., Carraro, R., and Sánchez-Ferrer, C. F. (2017). IL-1β inhibition in cardiovascular complications associated to diabetes mellitus. Front. Pharmacol. 8, 363. doi:10.3389/fphar.2017.00363
Peng, B., Shu, J., Hou, Z., Qian, S., Yan, B., Zhang, B., et al. (2024a). Vibrational spectroscopic detection and analysis of salicylic acid and aspirin binary cocrystal. Int. J. Pharm. 651, 123767. doi:10.1016/j.ijpharm.2024.123767
Peng, J., Tang, S., Huang, L., and Fang, Y. (2024b). Protective role of TRPM7 knockdown in ulcerative colitis via blocking NLRP3 inflammasome-mediated pyroptosis. Prostagl. and Other Lipid Mediat. 175, 106904. doi:10.1016/j.prostaglandins.2024.106904
Qin, W., Luo, H., Yang, L., Hu, D., Jiang, S. P., Peng, D. Y., et al. (2022). Rubia cordifolia L. ameliorates DSS-induced ulcerative colitis in mice through dual inhibition of NLRP3 inflammasome and IL-6/JAK2/STAT3 pathways. Heliyon 8, e10314. doi:10.1016/j.heliyon.2022.e10314
Rathinam, V. a.K., Zhao, Y., and Shao, F. (2019). Innate immunity to intracellular LPS. Nat. Immunol. 20, 527–533. doi:10.1038/s41590-019-0368-3
Ren, W., Sun, Y., Zhao, L., and Shi, X. (2024). NLRP3 inflammasome and its role in autoimmune diseases: a promising therapeutic target. Biomed. and Pharmacother. 175, 116679. doi:10.1016/j.biopha.2024.116679
Ren, Y., Sun, Q., Gao, R., Sheng, Y., Guan, T., Li, W., et al. (2023). Low weight polysaccharide of hericium erinaceus ameliorates colitis via inhibiting the NLRP3 inflammasome activation in association with gut microbiota modulation. Nutrients 15, 739. doi:10.3390/nu15030739
Ruan, Y., Zhu, X., Shen, J., Chen, H., and Zhou, G. (2024). Mechanism of Nicotiflorin in San-Ye-Qing rhizome for anti-inflammatory effect in ulcerative colitis. Phytomedicine 129, 155564. doi:10.1016/j.phymed.2024.155564
Saber, S., Abd El-Kader, E. M., Sharaf, H., El-Shamy, R., El-Saeed, B., Mostafa, A., et al. (2020). Celastrol augments sensitivity of NLRP3 to CP-456773 by modulating HSP-90 and inducing autophagy in dextran sodium sulphate-induced colitis in rats. Toxicol. Appl. Pharmacol. 400, 115075. doi:10.1016/j.taap.2020.115075
Schirmer, M., Stražar, M., Avila-Pacheco, J., Rojas-Tapias, D. F., Brown, E. M., Temple, E., et al. (2024). Linking microbial genes to plasma and stool metabolites uncovers host-microbial interactions underlying ulcerative colitis disease course. Cell Host Microbe 32, 209–226.e7. doi:10.1016/j.chom.2023.12.013
Shah, B., and Solanki, N. (2024a). Aegeline attenuates TNBS-induced colitis by suppressing the NFƙB-mediated NLRP3 inflammasome pathway in mice. Inflammopharmacology 32, 2589–2599. doi:10.1007/s10787-024-01493-0
Shah, B., and Solanki, N. (2024b). Ameliorative effect of nodakenin in combating TNBS-induced ulcerative colitis by suppressing NFƙB-mediated NLRP3 inflammasome pathway. Naunyn Schmiedeb. Arch. Pharmacol. 398, 673–686. doi:10.1007/s00210-024-03304-3
Shao, M., Yan, Y., Zhu, F., Yang, X., Qi, Q., Yang, F., et al. (2022). Artemisinin analog SM934 alleviates epithelial barrier dysfunction via inhibiting apoptosis and caspase-1-mediated pyroptosis in experimental colitis. Front. Pharmacol. 13, 849014. doi:10.3389/fphar.2022.849014
Shen, J., Zhao, Y., and Cui, W. (2024). Astragalus mongholicus Bunge extract improves ulcerative colitis by promoting PLCB2 to inhibit colonic epithelial cell pyroptosis. J. Ethnopharmacol. 334, 118554. doi:10.1016/j.jep.2024.118554
Shen, P., Wang, H. G., Li, M. M., Ma, Q. Y., Zhou, C. W., Pan, F., et al. (2017). Isofraxidin inhibited proliferation and induced apoptosis via blockage of Akt pathway in human colorectal cancer cells. Biomed. Pharmacother. 92, 78–85. doi:10.1016/j.biopha.2017.05.065
Shen, P., Zhang, Z., Zhu, K., Cao, H., Liu, J., Lu, X., et al. (2019). Evodiamine prevents dextran sulfate sodium-induced murine experimental colitis via the regulation of NF-κB and NLRP3 inflammasome. Biomed. Pharmacother. 110, 786–795. doi:10.1016/j.biopha.2018.12.033
Shen, Z. H., Zhu, C. X., Quan, Y. S., Yang, Z. Y., Wu, S., Luo, W. W., et al. (2018). Relationship between intestinal microbiota and ulcerative colitis: mechanisms and clinical application of probiotics and fecal microbiota transplantation. World J. Gastroenterol. 24, 5–14. doi:10.3748/wjg.v24.i1.5
Shi, J., Gao, W., and Shao, F. (2017). Pyroptosis: gasdermin-mediated programmed necrotic cell death. Trends Biochem. Sci. 42, 245–254. doi:10.1016/j.tibs.2016.10.004
Stakenborg, M., Verstockt, B., Meroni, E., Goverse, G., De Simone, V., Verstockt, S., et al. (2020). Neutrophilic HGF-MET signalling exacerbates intestinal inflammation. J. Crohns Colitis 14, 1748–1758. doi:10.1093/ecco-jcc/jjaa121
Sun, J., Wang, S., Zhao, Z., Lu, J., Zhang, Y., An, W., et al. (2024a). Oxymatrine attenuates ulcerative colitis through inhibiting pyroptosis mediated by the NLRP3 inflammasome. Molecules 29, 2897. doi:10.3390/molecules29122897
Sun, X., Jin, X., Wang, L., Lin, Z., Feng, H., Zhan, C., et al. (2024b). Fraxetin ameliorates symptoms of dextran sulphate sodium-induced colitis in mice. Heliyon 10, e23295. doi:10.1016/j.heliyon.2023.e23295
Tan, X., Wen, Y., Han, Z., Su, X., Peng, J., Chen, F., et al. (2023). Cinnamaldehyde ameliorates dextran sulfate sodium-induced colitis in mice by modulating TLR4/NF-κB signaling pathway and NLRP3 inflammasome activation. Chem. Biodivers. 20, e202200089. doi:10.1002/cbdv.202200089
Tan, Y., Chen, Q., Li, X., Zeng, Z., Xiong, W., Li, G., et al. (2021). Pyroptosis: a new paradigm of cell death for fighting against cancer. J. Exp. Clin. Cancer Res. 40, 153. doi:10.1186/s13046-021-01959-x
Tomou, E. M., Papakyriakopoulou, P., Saitani, E. M., Valsami, G., Pippa, N., and Skaltsa, H. (2023). Recent advances in nanoformulations for quercetin delivery. Pharmaceutics 15, 1656. doi:10.3390/pharmaceutics15061656
Tong, X., Chen, L., He, S., Liu, S., Yao, J., Shao, Z., et al. (2023). Forsythia suspensa (Thunb.) Vahl extract ameliorates ulcerative colitis via inhibiting NLRP3 inflammasome activation through the TLR4/MyD88/NF-κB pathway. Immun. Inflamm. Dis. 11, e1069. doi:10.1002/iid3.1069
Ullah, H. M. A., Saba, E., Lee, Y. Y., Hong, S. B., Hyun, S. H., Kwak, Y. S., et al. (2022). Restorative effects of Rg3-enriched Korean Red Ginseng and Persicaria tinctoria extract on oxazolone-induced ulcerative colitis in mice. J. Ginseng Res. 46, 628–635. doi:10.1016/j.jgr.2021.07.001
Vasudevan, S. O., Behl, B., and Rathinam, V. A. (2023). Pyroptosis-induced inflammation and tissue damage. Semin. Immunol. 69, 101781. doi:10.1016/j.smim.2023.101781
Voet, S., Srinivasan, S., Lamkanfi, M., and Van Loo, G. (2019). Inflammasomes in neuroinflammatory and neurodegenerative diseases. EMBO Mol. Med. 11, e10248. doi:10.15252/emmm.201810248
Wang, H. Y., Lin, X., Huang, G. G., Zhou, R., Lei, S. Y., Ren, J., et al. (2023a). Atranorin inhibits NLRP3 inflammasome activation by targeting ASC and protects NLRP3 inflammasome-driven diseases. Acta Pharmacol. Sin. 44, 1687–1700. doi:10.1038/s41401-023-01054-1
Wang, J., Wang, X., Ma, X., Xu, B., Chen, L., Chen, C., et al. (2022a). Therapeutic effect of Patrinia villosa on TNBS-induced ulcerative colitis via metabolism, vitamin D receptor and NF-κB signaling pathways. J. Ethnopharmacol. 288, 114989. doi:10.1016/j.jep.2022.114989
Wang, J., Yao, J., Liu, Y., and Huang, L. (2021). Targeting the gasdermin D as a strategy for ischemic stroke therapy. Biochem. Pharmacol. 188, 114585. doi:10.1016/j.bcp.2021.114585
Wang, K., Sun, Q., Zhong, X., Zeng, M., Zeng, H., Shi, X., et al. (2020). Structural mechanism for GSDMD targeting by autoprocessed caspases in pyroptosis. Cell 180, 941–955. doi:10.1016/j.cell.2020.02.002
Wang, N., Kong, R., Han, W., Bao, W., Shi, Y., Ye, L., et al. (2022b). Honokiol alleviates ulcerative colitis by targeting PPAR-γ-TLR4-NF-κB signaling and suppressing gasdermin-D-mediated pyroptosis in vivo and in vitro. Int. Immunopharmacol. 111, 109058. doi:10.1016/j.intimp.2022.109058
Wang, S., Du, Q., Meng, X., and Zhang, Y. (2022c). Natural polyphenols: a potential prevention and treatment strategy for metabolic syndrome. Food Funct. 13, 9734–9753. doi:10.1039/d2fo01552h
Wang, S., Wu, P., Fan, Z., He, X., Liu, J., Li, M., et al. (2023b). Dandelion polysaccharide treatment protects against dextran sodium sulfate-induced colitis by suppressing NF-κB/NLRP3 inflammasome-mediated inflammation and activating Nrf2 in mouse colon. Food Sci. Nutr. 11, 7271–7282. doi:10.1002/fsn3.3653
Wang, T., Liu, X., Zhang, W., Wang, J., Wang, T., Yue, W., et al. (2024a). Traditional Chinese medicine treats ulcerative colitis by regulating gut microbiota, signaling pathway and cytokine: future novel method option for pharmacotherapy. Heliyon 10, e27530. doi:10.1016/j.heliyon.2024.e27530
Wang, X., Shen, C., Wang, X., Tang, J., Wu, Z., Huang, Y., et al. (2023c). Schisandrin protects against ulcerative colitis by inhibiting the SGK1/NLRP3 signaling pathway and reshaping gut microbiota in mice. Chin. Med. 18, 112. doi:10.1186/s13020-023-00815-8
Wang, Y., Tang, Q., Duan, P., and Yang, L. (2018). Curcumin as a therapeutic agent for blocking NF-κB activation in ulcerative colitis. Immunopharmacol. Immunotoxicol. 40, 476–482. doi:10.1080/08923973.2018.1469145
Wang, Z., Li, C., He, X., Xu, K., Xue, Z., Wang, T., et al. (2022d). Platycodon grandiflorum root fermentation broth reduces inflammation in a mouse IBD model through the AMPK/NF-κB/NLRP3 pathway. Food Funct. 13, 3946–3956. doi:10.1039/d1fo03969e
Wang, Z., Lian, W., Chen, C., Dai, Q., Liu, Z., Liu, J., et al. (2024b). Network pharmacology and experimental verification revealing valnemulin alleviates DSS-induced ulcerative colitis by inhibiting intestinal senescence. Int. Immunopharmacol. 141, 112810. doi:10.1016/j.intimp.2024.112810
Wangchuk, P., Yeshi, K., and Loukas, A. (2024). Ulcerative colitis: clinical biomarkers, therapeutic targets, and emerging treatments. Trends Pharmacol. Sci. 45, 892–903. doi:10.1016/j.tips.2024.08.003
Wei, X., Ma, N., Yang, W., Tian, J., Liu, H., and Fang, H. (2024). Polyphenol extracts from Ziziphus jujuba Mill. “Junzao” attenuates ulcerative colitis by inhibiting the NLRP3 and MAPKs signaling pathways and regulating gut microbiota homeostasis in mice. Mol. Nutr. Food Res. 68, e2300643. doi:10.1002/mnfr.202300643
Wei, Y.-Y., Fan, Y.-M., Ga, Y., Zhang, Y.-N., Han, J.-C., and Hao, Z.-H. (2021). Shaoyao decoction attenuates DSS-induced ulcerative colitis, macrophage and NLRP3 inflammasome activation through the MKP1/NF-κB pathway. Phytomedicine 92, 153743. doi:10.1016/j.phymed.2021.153743
Wu, M., Li, P., An, Y., Ren, J., Yan, D., Cui, J., et al. (2019). Phloretin ameliorates dextran sulfate sodium-induced ulcerative colitis in mice by regulating the gut microbiota. Pharmacol. Res. 150, 104489. doi:10.1016/j.phrs.2019.104489
Wu, N., Wan, Z. P., Han, L., Liu, H. Y., and Li, H. S. (2021). Effect of Huangqin Decoction on pyroptosis pathway of NLRP3/caspase-1 in mice with ulcerative colitis. Zhongguo Zhong Yao Za Zhi 46, 1191–1196. doi:10.19540/j.cnki.cjcmm.20201117.402
Xiao, S., Yan, Y., Shao, M., Zhou, X., Niu, Z., Wu, Y., et al. (2024). Kuijieling decoction regulates the Treg/Th17 cell balance in ulcerative colitis through the RA/RARα signaling pathway. J. Ethnopharmacol. 318, 116909. doi:10.1016/j.jep.2023.116909
Xing, D., Zheng, T., Chen, X., and Xie, Z. (2024). Yellow teas protect against DSS-induced ulcerative colitis by inhibiting TLR4/NF-κB/NLRP3 inflammasome in mice. Foods 13, 2843. doi:10.3390/foods13172843
Xue, H., Li, P., Luo, Y., Wu, C., Liu, Y., Qin, X., et al. (2019). Salidroside stimulates the Sirt1/PGC-1α axis and ameliorates diabetic nephropathy in mice. Phytomedicine 54, 240–247. doi:10.1016/j.phymed.2018.10.031
Xue, J. C., Yuan, S., Hou, X. T., Meng, H., Liu, B. H., Cheng, W. W., et al. (2023). Natural products modulate NLRP3 in ulcerative colitis. Front. Pharmacol. 14, 1265825. doi:10.3389/fphar.2023.1265825
Xue, S., Xue, Y., Dou, D., Wu, H., Zhang, P., Gao, Y., et al. (2022). Kui Jie Tong ameliorates ulcerative colitis by regulating gut microbiota and NLRP3/caspase-1 classical pyroptosis signaling pathway. Dis. Markers 2022, 2782112. doi:10.1155/2022/2782112
Yan, J., Tang, Y., Yu, W., Jiang, L., Liu, C., Li, Q., et al. (2022a). Validation of the anticolitis efficacy of the jian-wei-yu-yang formula. Evid. Based Complement. Altern. Med. 2022, 9110704. doi:10.1155/2022/9110704
Yan, S., Wang, P., Wei, H., Jia, R., Zhen, M., Li, Q., et al. (2022b). Treatment of ulcerative colitis with Wu-Mei-Wan by inhibiting intestinal inflammatory response and repairing damaged intestinal mucosa. Phytomedicine 105, 154362. doi:10.1016/j.phymed.2022.154362
Yang, F., Bettadapura, S. N., Smeltzer, M. S., Zhu, H., and Wang, S. (2022). Pyroptosis and pyroptosis-inducing cancer drugs. Acta Pharmacol. Sin. 43, 2462–2473. doi:10.1038/s41401-022-00887-6
Yang, L., Ma, X. Y., Mu, K. X., Dai, Y., Xia, Y. F., and Wei, Z. F. (2023). Galangin targets HSP90β to alleviate ulcerative colitis by controlling fatty acid synthesis and subsequent NLRP3 inflammasome activation. Mol. Nutr. Food Res. 67, e2200755. doi:10.1002/mnfr.202200755
Yang, Y., Li, S., Liu, K., Zhang, Y., Zhu, F., Ben, T., et al. (2024). Lipocalin-2-mediated intestinal epithelial cells pyroptosis via NF-κB/NLRP3/GSDMD signaling axis adversely affects inflammation in colitis. Biochimica Biophysica Acta (BBA) - Mol. Basis Dis. 1870, 167279. doi:10.1016/j.bbadis.2024.167279
Ye, B., and Lai, L. (2021). Yu Shi an Chang Fang ameliorates TNBS-induced colitis in mice by reducing inflammatory response and protecting the intestinal mucosal barrier. Evid. Based Complement. Altern. Med. 2021, 8870901. doi:10.1155/2021/8870901
Ye, Z., Li, L., Li, Y., Hu, Y., Wu, M., Yu, H., et al. (2023). Tou Nong powder obstructs ulcerative colitis through the regulation of NF-κB/NLRP3/Caspase-1/GSDMD inflammasome pyroptotic pathway. J. Ethnopharmacol. 317, 116846. doi:10.1016/j.jep.2023.116846
Yu, P., Xu, F., Wu, H., Wang, X., Ding, Q., Zhang, M., et al. (2023a). Anti-ulcerative colitis effects and active ingredients in ethyl acetate extract from decoction of sargentodoxa cuneata. Molecules 28, 7663. doi:10.3390/molecules28227663
Yu, S., Qian, H., Zhang, D., and Jiang, Z. (2023b). Ferulic acid relieved ulcerative colitis by inhibiting the TXNIP/NLRP3 pathway in rats. Cell Biol. Int. 47, 417–427. doi:10.1002/cbin.11935
Yu, T. Y., Feng, Y. M., Kong, W. S., Li, S. N., Sun, X. J., Zhou, G., et al. (2023c). Gallic acid ameliorates dextran sulfate sodium-induced ulcerative colitis in mice via inhibiting NLRP3 inflammasome. Front. Pharmacol. 14, 1095721. doi:10.3389/fphar.2023.1095721
Yu, W., Liang, Z., Li, Q., Liu, Y., Liu, X., Jiang, L., et al. (2022a). The pharmacological validation of the Xiao-Jian-Zhong formula against ulcerative colitis by network pharmacology integrated with metabolomics. J. Ethnopharmacol. 298, 115647. doi:10.1016/j.jep.2022.115647
Yu, W., Wang, G., Lu, C., Liu, C., Jiang, L., Jiang, Z., et al. (2022b). Pharmacological mechanism of Shenlingbaizhu formula against experimental colitis. Phytomedicine 98, 153961. doi:10.1016/j.phymed.2022.153961
Yuan, W., Tian, Y., Lin, C., Wang, Y., Liu, Z., Zhao, Y., et al. (2023). Pectic polysaccharides derived from Hainan Rauwolfia ameliorate NLR family pyrin domain-containing 3-mediated colonic epithelial cell pyroptosis in ulcerative colitis. Physiol. Genomics 55, 27–40. doi:10.1152/physiolgenomics.00081.2022
Zang, Z., Li, L., Yang, M., Zhang, H., Naeem, A., Wu, Z., et al. (2024). Study on the ameliorative effect of honeysuckle on DSS-induced ulcerative colitis in mice. J. Ethnopharmacol. 325, 117776. doi:10.1016/j.jep.2024.117776
Zeng, J., Zhang, D., Wan, X., Bai, Y., Yuan, C., Wang, T., et al. (2020). Chlorogenic acid suppresses miR-155 and ameliorates ulcerative colitis through the NF-κB/NLRP3 inflammasome pathway. Mol. Nutr. Food Res. 64, e2000452. doi:10.1002/mnfr.202000452
Zeng, L., Yang, T., Yang, K., Yu, G., Li, J., Xiang, W., et al. (2022). Curcumin and curcuma longa extract in the treatment of 10 types of autoimmune diseases: a systematic review and meta-analysis of 31 randomized controlled trials. Front. Immunol. 13, 896476. doi:10.3389/fimmu.2022.896476
Zhan, X., Li, Q., Xu, G., Xiao, X., and Bai, Z. (2022a). The mechanism of NLRP3 inflammasome activation and its pharmacological inhibitors. Front. Immunol. 13, 1109938. doi:10.3389/fimmu.2022.1109938
Zhan, X., Peng, W., Wang, Z., Liu, X., Dai, W., Mei, Q., et al. (2022b). Polysaccharides from garlic protect against liver injury in DSS-induced inflammatory bowel disease of mice via suppressing pyroptosis and oxidative damage. Oxid. Med. Cell Longev. 2022, 2042163. doi:10.1155/2022/2042163
Zhang, D., Ge, F., Ji, J., Li, Y. J., Zhang, F. R., Wang, S. Y., et al. (2023). β-sitosterol alleviates dextran sulfate sodium-induced experimental colitis via inhibition of NLRP3/Caspase-1/GSDMD-mediated pyroptosis. Front. Pharmacol. 14, 1218477. doi:10.3389/fphar.2023.1218477
Zhang, H. Y., Zeng, H. R., Wei, H. Z., Chu, X. Y., Zhu, H. T., Zhao, B., et al. (2022a). Tongxie-Yaofang formula regulated macrophage polarization to ameliorate DSS-induced colitis via NF-κB/NLRP3 signaling pathway. Phytomedicine 107, 154455. doi:10.1016/j.phymed.2022.154455
Zhang, J. X., Hu, Y. X., Liu, Y., Chen, Z. Z., Zheng, J. T., Qu, X. T., et al. (2024a). Xianglian pill alleviates ulcerative colitis by inhibiting M1 macrophage polarization via modulation of energy metabolite itaconate. Phytomedicine 135, 156179. doi:10.1016/j.phymed.2024.156179
Zhang, L., Song, J., Kong, L., Yuan, T., Li, W., Zhang, W., et al. (2020a). The strategies and techniques of drug discovery from natural products. Pharmacol. Ther. 216, 107686. doi:10.1016/j.pharmthera.2020.107686
Zhang, L. X., Li, C. X., Kakar, M. U., Khan, M. S., Wu, P. F., Amir, R. M., et al. (2021a). Resveratrol (RV): a pharmacological review and call for further research. Biomed. Pharmacother. 143, 112164. doi:10.1016/j.biopha.2021.112164
Zhang, Q., Wang, S., and Ji, S. (2022b). Trifolirhizin regulates the balance of Th17/Treg cells and inflammation in the ulcerative colitis mice through inhibiting the TXNIP-mediated activation of NLRP3 inflammasome. Clin. Exp. Pharmacol. Physiol. 49, 787–796. doi:10.1111/1440-1681.13654
Zhang, Q., Xu, N., Hu, X., and Zheng, Y. (2020b). Anti-colitic effects of Physalin B on dextran sodium sulfate-induced BALB/c mice by suppressing multiple inflammatory signaling pathways. J. Ethnopharmacol. 259, 112956. doi:10.1016/j.jep.2020.112956
Zhang, W., Wang, W., Shen, C., Wang, X., Pu, Z., and Yin, Q. (2021b). Network pharmacology for systematic understanding of Schisandrin B reduces the epithelial cells injury of colitis through regulating pyroptosis by AMPK/Nrf2/NLRP3 inflammasome. Aging (Albany NY) 13, 23193–23209. doi:10.18632/aging.203611
Zhang, W. J., Chen, S. J., Zhou, S. C., Wu, S. Z., and Wang, H. (2021c). Inflammasomes and fibrosis. Front. Immunol. 12, 643149. doi:10.3389/fimmu.2021.643149
Zhang, Y., Wang, H., Sang, Y., Liu, M., Wang, Q., Yang, H., et al. (2024b). Gut microbiota in health and disease: advances and future prospects. MedComm 5, e70012. doi:10.1002/mco2.70012
Zhang, Z., Li, S., Cao, H., Shen, P., Liu, J., Fu, Y., et al. (2019). The protective role of phloretin against dextran sulfate sodium-induced ulcerative colitis in mice. Food Funct. 10, 422–431. doi:10.1039/c8fo01699b
Zhao, L., Weng, W., Ni, M., Shen, H., Zhang, S., Chen, Y., et al. (2024a). Rubidium salt can effectively relieve the symptoms of DSS-induced ulcerative colitis. Biomed. and Pharmacother. 181, 117574. doi:10.1016/j.biopha.2024.117574
Zhao, P., Ning, J., Huang, J., and Huang, X. (2024b). Mechanism of Resveratrol on LPS/ATP-induced pyroptosis and inflammatory response in HT29 cells. Autoimmunity 57, 2427094. doi:10.1080/08916934.2024.2427094
Zhao, X., Chen, X., and Yue, C. (2024c). Rutin ameliorates inflammation and oxidative stress in ulcerative colitis by inhibiting NLRP3 inflammasome signaling pathway. Cell Biochem. Biophys. 82, 3715–3726. doi:10.1007/s12013-024-01459-7
Zhao, X., Cui, D., Yuan, W., Chen, C., and Liu, Q. (2022a). Berberine represses Wnt/β-catenin pathway activation via modulating the microRNA-103a-3p/Bromodomain-containing protein 4 axis, thereby refraining pyroptosis and reducing the intestinal mucosal barrier defect induced via colitis. Bioengineered 13, 7392–7409. doi:10.1080/21655979.2022.2047405
Zhao, Y., Liu, P., Zhang, Y., Jiang, H., Luan, H., Xu, Y., et al. (2022b). Demethyleneberberine blocked the maturation of IL-1β in inflammation by inhibiting TLR4-mitochondria signaling. Int. Immunopharmacol. 113, 109319. doi:10.1016/j.intimp.2022.109319
Zhao, Y., Ma, Y., Pei, J., Zhao, X., Jiang, Y., and Liu, Q. (2024d). Exploring pyroptosis-related signature genes and potential drugs in ulcerative colitis by transcriptome data and animal experimental validation. Inflammation 47, 2057–2076. doi:10.1007/s10753-024-02025-2
Zhao, Z. H., Dong, Y. H., Jiang, X. Q., Wang, J., Qin, W. L., Liu, Z. Y., et al. (2024e). Five commonly used traditional Chinese medicine formulas in the treatment of ulcerative colitis: a network meta-analysis. World J. Clin. Cases 12, 5067–5082. doi:10.12998/wjcc.v12.i22.5067
Zhen, Y., and Zhang, H. (2019). NLRP3 inflammasome and inflammatory bowel disease. Front. Immunol. 10, 276. doi:10.3389/fimmu.2019.00276
Zheng, S., Xue, T., Wang, B., Guo, H., and Liu, Q. (2022). Chinese medicine in the treatment of ulcerative colitis: the mechanisms of signaling pathway regulations. Am. J. Chin. Med. 50, 1781–1798. doi:10.1142/s0192415x22500756
Zheng, X., Wan, J., and Tan, G. (2023). The mechanisms of NLRP3 inflammasome/pyroptosis activation and their role in diabetic retinopathy. Front. Immunol. 14, 1151185. doi:10.3389/fimmu.2023.1151185
Zhou, C., Peng, B., Zhang, M., Yang, Y., Yi, Z., and Wu, Y. (2024a). Ganjiang Huangqin Huanglian Renshen Decoction protects against ulcerative colitis by modulating inflammation, oxidative stress, and gut microbiota. Phytomedicine 135, 156172. doi:10.1016/j.phymed.2024.156172
Zhou, J., Wang, J., Wang, J., Li, D., Hou, J., Li, J., et al. (2024b). An inulin-type fructan CP-A from Codonopsis pilosula attenuates experimental colitis in mice by promoting autophagy-mediated inactivation of NLRP3 inflammasome. Chin. J. Nat. Med. 22, 249–264. doi:10.1016/s1875-5364(24)60556-x
Zhou, J., Wang, T., Dou, Y., Huang, Y., Qu, C., Gao, J., et al. (2018). Brusatol ameliorates 2, 4, 6-trinitrobenzenesulfonic acid-induced experimental colitis in rats: involvement of NF-κB pathway and NLRP3 inflammasome. Int. Immunopharmacol. 64, 264–274. doi:10.1016/j.intimp.2018.09.008
Zhou, J., Yang, Q., Wei, W., Huo, J., and Wang, W. (2025). Codonopsis pilosula polysaccharide alleviates ulcerative colitis by modulating gut microbiota and SCFA/GPR/NLRP3 pathway. J. Ethnopharmacol. 337, 118928. doi:10.1016/j.jep.2024.118928
Zhou, M., Ma, X., Zhang, J., and Lu, X. (2024c). 3,5-Dimethyl-8-methoxy-3,4-dihydro-1H-isochromen-6-ol attenuates ulcerative colitis via targeting NLRP3 in mice. J. Agric. Food Chem. 72, 16700–16707. doi:10.1021/acs.jafc.4c00971
Zhou, Z., He, W., Tian, H., Zhan, P., and Liu, J. (2023a). Thyme (Thymus vulgaris L.) polyphenols ameliorate DSS-induced ulcerative colitis of mice by mitigating intestinal barrier damage, regulating gut microbiota, and suppressing TLR4/NF-κB-NLRP3 inflammasome pathways. Food Funct. 14, 1113–1132. doi:10.1039/d2fo02523j
Zhou, Z., Wang, Y., Sun, S., Zhang, K., Wang, L., Zhao, H., et al. (2023b). Paeonia lactiflora Pall. Polysaccharide alleviates depression in CUMS mice by inhibiting the NLRP3/ASC/Caspase-1 signaling pathway and affecting the composition of their intestinal flora. J. Ethnopharmacol. 316, 116716. doi:10.1016/j.jep.2023.116716
Zhu, F., Zhi, Y., Li, Y., Niu, H., and Ren, S. (2024). The mechanism of Polygonum hydropiper L-coptis chinensis in the treatment of ulcerative colitis based on network pharmacology and experimental validation. Front. Biosci. Landmark Ed. 29, 93. doi:10.31083/j.fbl2903093
Zohny, M. H., Alrouji, M., Alhajlah, S., Alomeir, O., Ewees, M. G. E., Ghaffar, D. M. A., et al. (2022). Diacetylrhein, an anthraquinone antiarthritic agent, suppresses dextran sodium sulfate-induced inflammation in rats: a possible mechanism for a protective effect against ulcerative colitis. Biomed. Pharmacother. 154, 113651. doi:10.1016/j.biopha.2022.113651
AIM2 absent in melanoma 2
APE Apple polyphenols extract
BBR Berberine
CSR celastrol
COX2 cyclooxygenase-2
CAT catalase
DSS dextran sulfate sodium
DAR Diacetylrhein
DAI Disease Activity Index
DAMPs damage-associated molecular patterns
EVO Evodiamine
GA Gallic acid
GSDMD gasdermin D
GSDME gasdermin E
GTN Gentianine
GSH glutathione
HSP-90 heat shock protein 90
HZJDD Huazhuojiedu decoction
KJT Kui Jie Tong
MPO myeloperoxidase
MDA malondialdehyde
MPO myeloperoxidase
NOS nitric oxide synthase
NRF2 nuclear factor erythroid 2-related factor 2
NLRs NOD-like receptors
PAMPs pathogen-associated molecular patterns
PRRs pattern recognition receptors
Pg3gal Pelargonidin-3-galactoside
PPs Pectic polysaccharides
PH-CC Polygonum Hydropiper L. and Coptis Chinensis
PJ Portulaca oleracea juice
POE Portulaca oleracea extract
PSG polysaccharides derived from garlic
SOD1 superoxide dismutase 1
SCFAs short-chain fatty acids
STAT3 signal transducer and activator of transcription 3
SM934 β-aminoarteether maleate
SLBZS Shen-ling-bai-zhu-san
SLBZ Shenlingbaizhu formula
SYD Shao Yao Decoction
TLR4 Toll-like receptor 4
TCM Traditional Chinese medicine
TNP Tou Nong powder
UC Ulcerative colitis
XLP Xianglian pill
XJZ Xiao-Jian-Zhong formula
YST Yu Shi An Chang Fang
ZO-1 zonula occludens-1
10-HAD Trans-10-Hydroxy-2-Decenoic Acid
Keywords: ulcerative colitis, pyroptosis, natural products, NLRP3, flavonoids, polyphenols
Citation: Lu X, Sun Y, Zhang Z, Sun Z, Wang S and Xu E (2025) Regulation of pyroptosis by natural products in ulcerative colitis: mechanisms and therapeutic potential. Front. Pharmacol. 16:1573684. doi: 10.3389/fphar.2025.1573684
Received: 09 February 2025; Accepted: 31 March 2025;
Published: 09 April 2025.
Edited by:
Ramzi Mothana, King Saud University, Saudi ArabiaReviewed by:
Ulrike Lindequist, University of Greifswald, GermanyCopyright © 2025 Lu, Sun, Zhang, Sun, Wang and Xu. This is an open-access article distributed under the terms of the Creative Commons Attribution License (CC BY). The use, distribution or reproduction in other forums is permitted, provided the original author(s) and the copyright owner(s) are credited and that the original publication in this journal is cited, in accordance with accepted academic practice. No use, distribution or reproduction is permitted which does not comply with these terms.
*Correspondence: Erping Xu, eHVlcnBpbmdAaGFjdGNtLmVkdS5jbg==; Shaohui Wang, d2ludGVyOTA5MUAxNjMuY29t
Disclaimer: All claims expressed in this article are solely those of the authors and do not necessarily represent those of their affiliated organizations, or those of the publisher, the editors and the reviewers. Any product that may be evaluated in this article or claim that may be made by its manufacturer is not guaranteed or endorsed by the publisher.
Research integrity at Frontiers
Learn more about the work of our research integrity team to safeguard the quality of each article we publish.