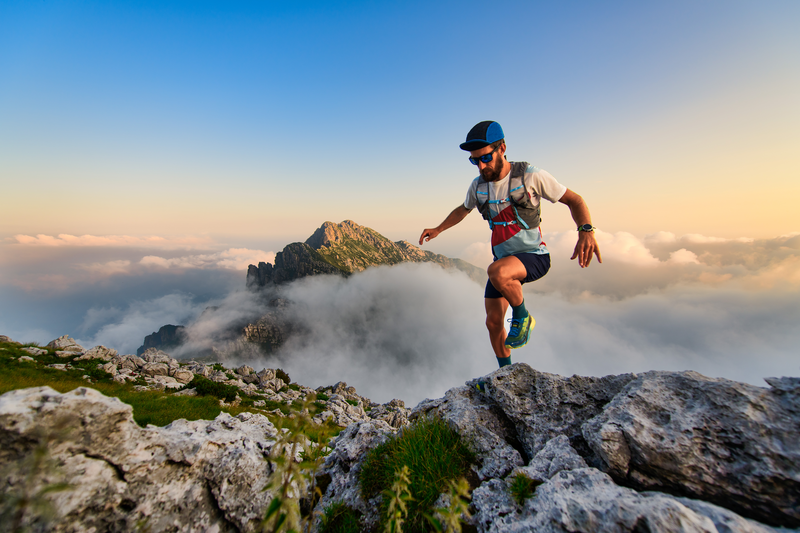
95% of researchers rate our articles as excellent or good
Learn more about the work of our research integrity team to safeguard the quality of each article we publish.
Find out more
REVIEW article
Front. Pharmacol. , 26 March 2025
Sec. Ethnopharmacology
Volume 16 - 2025 | https://doi.org/10.3389/fphar.2025.1569141
This article is part of the Research Topic Food Derived Bioactive Metabolites: Unlocking their Potential Health Benefits and Medical Potential View all 10 articles
Pomegranate peel is a by-product generated during the processing of pomegranate (Punica granatum L.) fruit, accounting for approximately 50% of the total mass of the fruit. Although pomegranate peel is usually regarded as waste, it is rich in various bioactive metabolites such as polyphenols, tannins, and flavonoids, demonstrating significant medicinal and nutritional value. In recent years, Pomegranate peel extract (PPE) has shown broad application prospects in the biomedical field due to its multiple effects, including antioxidant, anti-inflammatory, antibacterial, anti-apoptotic properties, and promotion of cell regeneration. This review consolidates the major bioactive metabolites of PPE and explores its applications in biomedical materials, including nanodrug carriers, hydrogels, and tissue engineering scaffolds. By synthesizing the existing literature, we delve into the potential value of PPE in biomedicine, the challenges currently encountered, and the future directions for research. The aim of this review is to provide a scientific basis for optimizing the utilization of PPE and to facilitate its broader application in the biomedical field.
Pomegranate (Punica granatum L.), belonging to the family Lythraceae and the genus Punica, is one of the oldest edible fruits, originating in Persia (modern-day Iran) and surrounding areas. It was later spread to Asia, North Africa, and the Mediterranean regions through activities such as seafaring and wars, and is now widely cultivated around the world (Benedetti et al., 2023; Langgut, 2024). It is estimated that the total global cultivation area of pomegranate exceeds 300,000 ha, with an annual production of approximately 3 million tons (Melgarejo-Sánchez et al., 2021). Pomegranate peel is one of the main components of the fruit, accounting for about 50% (Arun et al., 2022; Derakhshan et al., 2018). In current pomegranate product processing, pomegranate peel is usually discarded as waste, resulting in around 1.6 million tons of waste annually (Bertolo et al., 2021; Giri et al., 2023). In addition to resource wastage, pomegranate peel waste negatively impacts natural resources such as land and water, further exacerbating environmental issues (Sagar et al., 2018).
Pomegranate peel is not without value but possesses unique medicinal properties. Thousands of years ago, pomegranate peel was widely used in traditional medicine across many cultures (Li et al., 2006; Liu et al., 2012). Historically, pomegranate peel has been utilized in various cultures for medicinal purposes. The Romans employed it as an anthelmintic, while some ethnic groups in the Middle East and South America have used boiled pomegranate peel for treating dysentery (Ge et al., 2021; Longtin, 2003). In traditional Chinese medicine, pomegranate peel is regarded as a potent astringent and anti-inflammatory agent, applied to treat traumatic bleeding, infections, and gastrointestinal disorders such as diarrhea (Mo et al., 2013). In Ayurvedic medicine, it is similarly valued as an effective remedy for oral infections (Rafey et al., 2021). In modern times, the medicinal efficacy of pomegranate peel has been scientifically validated. Studies reveal that pomegranate peel is rich in bioactive metabolites such as tannins, phenolic acids, and flavonoids, which impart a range of pharmacological effects, including antioxidant, anti-inflammatory, antibacterial, and anticancer activities (Ain et al., 2023; Ozkan, 2023; Siddiqui et al., 2024). Research has demonstrated that the antioxidant capacity of pomegranate peel extract (PPE) is nearly ten times greater than that of other parts of the pomegranate fruit, a property closely linked to its polyphenolic structure (Hanafy et al., 2021). Furthermore, PPE is capable of modulating several critical molecular pathways, including mitogen-activated protein kinases, nuclear factor-kappa B (NF-κB), cysteine-aspartic acid-specific proteases, and peroxisome proliferator-activated receptors, thus providing theoretical support for its use in the treatment of a variety of diseases (Andishmand et al., 2025). In addition to its notable pharmacological properties, PPE can be processed into nanodrug carriers, hydrogels, and other biomedical materials through chemical reduction, complexation, emulsification, and hydration methods, offering potential therapeutic applications for diseases such as diabetes and breast cancer (Andishmand et al., 2023; Askari and Nikoonahad Lotfabadi, 2020; Baccarin and Lemos-Senna, 2017; Ganeshkumar et al., 2013; Savekar et al., 2024; Tiyaboonchai et al., 2015). Thus, utilizing pomegranate peel, a by-product, can achieve resource recycling and create new economic value.
This paper aims to explore the main bioactive metabolites of PPE and its applications in biomedicine (Figure 1), while providing an in-depth analysis of its limitations in practical applications and its development potential. The goal is to offer a theoretical foundation and scientific support for the future development and utilization of pomegranate peel.
The literature review was conducted using several databases, including Web of Science (https://webofscience.clarivate.cn), ScienceDirect (www.sciencedirect.com), and PubMed (https://www.ncbi.nlm.nih.gov/). The search keywords included “pomegranate peel extract,” “Punica granatum L.,” “bioactivity,” “hydrogels,” “nanodrugs,” “tissue engineering,” “biomedical materials,” and their combinations. All duplicate articles identified during the search were excluded. The species names were verified using the taxonomic database (http://mpns.kew.org/mpns-portal/).
The content of polyphenolic metabolites in pomegranate peel is significantly higher than in the flesh and seeds, containing ellagic acid, punicalagin, punicalin, and other ellagitannins and their derivatives, as well as gallic acid, coumaric acid, and other phenolic acids, along with flavonoids such as catechin and epicatechin (Table 1). These polyphenolic metabolites exhibit a wide range of bioactivities and can exert certain physiological or pharmacological effects on organisms, including antioxidant, anti-inflammatory, and antibacterial activities (Table 2).
Ellagitannins are derived from esters of hexahydroxydiphenic acid, which undergo hydrolysis to form ellagic acid, and bind with polyols. Through oxidative coupling reactions of ellagic acid moieties, ellagitannins form hexahydroxydiphenyl groups, which are dehydrodimeric ellagic acid esters with aromaticity (Molnar et al., 2024). This property gives ellagitannins a more complex structural characteristic compared to ordinary tannic acids. Ellagitannins in PPE include ellagic acid, punicalagin, punicalin, corilagin, and pedunculagin, among others. Punicalagin, characterized by a high total phenolic content, is the primary contributor to the antioxidant properties of pomegranate products (Nuncio-Jáuregui et al., 2015; Valero-Mendoza et al., 2023). Its antioxidant capacity is remarkably high, approximately 50%, whereas ellagic acid demonstrates a weaker antioxidant capacity, with only 3% per molecule (Shabir et al., 2024). Punicalagin contains galloyl groups esterified with glucose, which undergo hydrolysis to form ellagic acid and punicalin (Yamada et al., 2018). Punicalin retains significant antioxidant activity, effectively scavenging free radicals and reducing oxidative damage to cells, thereby contributing to the prevention of aging and related diseases (Magangana et al., 2020; Ranjha et al., 2023; Sharma et al., 2022).
The role of ellagitannins and their derivatives in disease prevention is closely tied to their antioxidant potential, which increases with the number of hydroxyl groups in their molecular structure (Gupta et al., 2019; Ismail et al., 2016). Pedunculagin, the most abundant and biologically active ellagitannin in pomegranate, has therapeutic effects on inflammatory diseases (Shabir et al., 2024; Xu et al., 2022). Seo et al. used a lupus-prone mouse model with spontaneously occurring severe rheumatoid disease to investigate the effects of different doses of pedunculagin (Seo et al., 2020). As the concentration of pedunculagin increased, the severity of renal damage in the mice significantly decreased. The study demonstrated that pedunculagin could alleviate lupus nephritis in mice by inhibiting the protease-activated receptor 2 pathway. Future research could further explore the role of potential pathways such as NF-κB or MAPK, and evaluate the potential toxic side effects of pedunculagin on the liver and kidneys. Nguyen et al. stimulated human placental, visceral fat, and subcutaneous fat tissue explants with tumor necrosis factor (TNF), and the study showed that punicalagin significantly inhibited the TNF-induced expression of pro-inflammatory factors (IL-1β, IL-6) in human placenta and adipose tissue (Nguyen-Ngo et al., 2020). A strength of this study was the use of human-derived tissues in vitro, providing greater clinical relevance. However, the limitation of this research lies in the lack of in vivo studies with animal models, leading to insufficient validation of its in vivo efficacy and mechanisms. Furthermore, other ellagitannins such as corilagin, casuarinin, and tellimagrandin, which contain multiple hydroxyl groups, exhibit significant antioxidant activity. Corilagin inhibits the reverse transcriptase activity of RNA tumor viruses, thereby demonstrating anticancer effects (Li et al., 2018). Casuarinin exhibits antiviral activity by inhibiting the adhesion and penetration of herpes simplex virus type 2 (Cheng et al., 2002). Chang et al.‘s study showed that tellimagrandin inhibits the activity of methicillin-resistant Staphylococcus aureus with a minimum inhibitory concentration of 128 μg/mL (Chang et al., 2019). Overall, ellagitannins and their derivatives possess multiple bioactivities, including antioxidant, anti-inflammatory, antibacterial, and antitumor effects, demonstrating broad application potential in disease prevention and treatment.
The metabolic pathways of ellagitannins in vivo show significant differences from those of tannic acids. Ellagitannins are not directly absorbed into the bloodstream but are metabolized through hydrolysis processes (Shabir et al., 2024). For example, metabolites such as punicalagin and corilagin are hydrolyzed under acidic or alkaline conditions to generate hexahydroxydiphenoyl (HHDP) groups, which spontaneously lactonize into ellagic acid (Evtyugin et al., 2020). Ellagic acid, a major metabolite of pomegranate peel (Kumar et al., 2022), is a polyphenolic dilactone that has been proven to exhibit various bioactivities, including antioxidant, anti-inflammatory, and antibacterial effects (Cuevas-Magana et al., 2022; Yu et al., 2023). After ingesting ellagitannins or ellagic acid, the human body metabolizes these metabolites through intestinal microbiota, producing 6H-dibenzo [b,d]pyran-6-one derivatives, also known as urolithins (Uros) (Figure 2), which are beneficial to health (Cerdá et al., 2003a; Cerdá et al., 2003b). Depending on the number and position of hydroxyl groups, Uros can be further classified into different types, such as urolithin A, urolithin B, urolithin C, etc. Long-term intake of ellagitannin-rich foods can increase the concentration of Uros in bodily fluids such as plasma and urine (Singh et al., 2022; Zhang et al., 2021; Zhang et al., 2020), as well as in tissues like the breast and bones (Andreux et al., 2019; Angeles Avila-Galvez et al., 2019). Research has shown that urolithins play a significant role in the biological activities within the body. For instance, in an inflammatory bowel disease model (Larrosa et al., 2010), following the administration of pomegranate extract, inflammation markers such as inducible nitric oxide synthase (iNOS), cyclooxygenase-2 (COX-2), and prostaglandin E synthase (PTGES) were significantly reduced in the inflammatory rats. Additionally, oxidative stress levels in the plasma and colonic mucosa decreased, and the concentration of urolithins in fecal extracts was significantly lower than in the healthy rat group. In a Parkinson’s disease model, after the administration of pomegranate extract, urolithins were detected in the brains of the affected rats, and improvements were observed in their olfactory and motor impairments (Kujawska et al., 2021). Furthermore, the levels of α-synuclein oligomers were also reduced (Kujawska et al., 2020). Additionally, urolithins can regulate cholesterol metabolism by reducing the abundance of Coriobacteriaceae bacteria and altering the bile acid pool, thereby promoting lipid absorption in the intestines and providing cardiovascular protection (Cortes-Martin et al., 2024). These findings highlight that foods rich in ellagic acid can positively impact health both in the gastrointestinal tract and systemically through urolithins.
Figure 2. Degradation Pathway of ellagic Acid Metabolism into Uros. In the figure, Uros are classified based on the number of hydroxyl groups (5-OH, 4-OH, 3-OH, 2-OH, 1-OH), and the final products include urolithin A, urolithin B, and Isourolithin A (Xiang et al., 2022).
Upon entering the human body, ellagitannins undergo bidirectional modulation with the gut microbiota. The gut microbiota can metabolize ellagitannins into urolithin metabolites through hydrolysis, reduction, and other reactions, while ellagitannins and their metabolites can also selectively regulate the composition of the microbiota (Caballero et al., 2022; Henning et al., 2022; Sanchez-Terron et al., 2024). For example, punicalagin intervention significantly enriched short-chain fatty acid-producing bacteria such as Akkermansia muciniphila and Ruminiclostridium 9 in the intestines of high-fat diet mice (Gao et al., 2024), while also increasing the abundance of Lachnospiraceae NK4A136 and Bifidobacterium, which have bile acid metabolism functions (Liu et al., 2024). The gut microbiota, as a “metabolic regulatory organ,” plays a crucial role in the bile acid metabolic cycle. Approximately 95% of primary bile acids are reabsorbed through the terminal ileum sodium-dependent bile acid transporter and enter the enterohepatic circulation, while the remaining 5% are metabolized by bile salt hydrolases and 7α-dehydroxylases secreted by Bacteroides, Clostridium, Bifidobacterium, and Lactobacillus into secondary bile acids such as deoxycholic acid and lithocholic acid (Begley et al., 2005; Ridlon et al., 2016). Secondary bile acids play a pivotal role in inflammation regulation. Studies have shown that the levels of secondary bile acids in the intestines of patients with inflammatory bowel disease are lower compared to healthy controls (Lloyd-Price et al., 2019). Supplementation with secondary bile acids can alleviate inflammation in acute and chronic colitis models in mice (Sinha et al., 2020), and remodeling the gut microbiota to improve bile acid metabolism can reduce colitis (Zhou et al., 2023). These findings suggest that ellagitannins may modulate disease development through the gut microbiota-bile acid axis. The bidirectional regulation of ellagitannins and the gut microbiota offers a new perspective on their potential health benefits. However, current research still faces certain limitations, particularly the lack of systematic studies on the combined administration of ellagitannins with specific probiotics. Future in-depth exploration in this area may not only help elucidate their synergistic mechanisms but also open new avenues for the application of PPE in functional nutritional supplements.
Pomegranate peel also contains flavonoids such as quercetin, catechin, and kaempferol. Among them, quercetin is one of the most common flavonoids, though its content in pomegranate peel is relatively low (Kumar et al., 2022). After oral administration, quercetin is metabolized into derivatives such as O-glycosides and C-glycosides. These metabolites are absorbed in the small intestine, bind to serum albumin, and are transported to the liver, where they undergo sulfation, glucuronidation, and methylation to form stable metabolites, which are then distributed throughout the body (Figure 3) (Manach et al., 1995; Nijveldt et al., 2001; Zhang et al., 2023). Quercetin exerts antioxidant effects by directly scavenging reactive oxygen species (ROS) and chelating metal ions (Min et al., 2019; Tang et al., 2014). It also improves cardiovascular health by modulating the hsp70/ERK/PPAR signaling pathway (Oyagbemi et al., 2018; Pereira et al., 2018).
Catechin is one of the most studied flavonoids in pharmacology and has been reported to possess diverse pharmacological activities. Catechin exhibits anticancer properties through mechanisms such as inducing apoptosis, inhibiting cancer cell proliferation, arresting the cell cycle, and regulating tumor-related signaling pathways (e.g., inhibiting AKT phosphorylation) (Shikha et al., 2024; Shukla et al., 2018; Sun et al., 2020). Manikandan et al. utilized HCT 15, HCT 116 (human colon adenocarcinoma cells), and Hep G-2 (human laryngeal carcinoma cells) as experimental models to investigate the synergistic anticancer activity of curcumin and catechin (Manikandan et al., 2012). The results indicated that both curcumin and catechin alone, as well as their combination, effectively inhibited the proliferation of HCT 15, HCT 116, and Hep G-2 cells. However, when combined, the cytotoxicity was more pronounced, significantly disrupting the monolayer of the cells. This suggests a synergistic effect between catechin and other anticancer agents like curcumin, enhancing the suppression of cancer cells. Notably, HCT 15, HCT 116, and Hep G-2 cells exhibited significant pathological differences, making it difficult to universally interpret the applicability of the synergistic effect. Furthermore, the study did not validate the shared targets of curcumin and catechin through molecular docking or protein interaction experiments. Therefore, future research should strengthen these aspects to clarify their molecular mechanisms. Additionally, catechin is an effective molecule for treating inflammation. It exerts anti-inflammatory effects by inhibiting the gene expression of pro-inflammatory cytokines such as IL-1α, IL-1β, IL-6, and IL-12p35, downregulating the activity of inflammatory enzymes like iNOS and COX-2, and suppressing key factors in inflammation signaling pathways such as NF-κB, FOXO3a, and SIRT1(Cheng et al., 2019). Moreover, catechin has been found to exhibit antibacterial activity against various bacterial strains by disrupting bacterial membranes, inhibiting spore and vegetative cell growth, and inducing oxidative stress (Hara-Kudo et al., 2005; Parvez et al., 2019). However, despite these studies showcasing the bioactivity of catechins, their clinical efficacy may be limited due to their low bioavailability in vivo.
Kaempferol is another important flavonoid metabolite found in pomegranate peel, exhibiting multiple bioactivities such as antiviral, anti-inflammatory, anti-metabolic disorder, and cardiovascular protective effects (Al-Numair et al., 2015; Hussain et al., 2024; Huwait et al., 2022; Zhuang et al., 2017). In terms of antiviral effects, kaempferol effectively inhibits hepatitis B virus activity by suppressing the synthesis of hepatitis B surface antigen and hepatitis B e antigen (Parvez et al., 2022). Studies have revealed that increasing kaempferol concentration significantly reduces intracellular viral DNA levels (Li et al., 2008). Additionally, kaempferol demonstrates neuroprotective properties in neurodegenerative diseases. In Alzheimer’s disease models of Drosophila expressing wild-type human amyloid-beta 42, kaempferol alleviates symptoms by reducing oxidative stress in the brain (Han et al., 2019). Li et al. induced Parkinson’s disease in C57BL/6 mice by injecting MPTP (neurotoxin) and treated the mice with different doses (25, 50, and 100 mg/kg) of kaempferol for 14 consecutive days prior to MPTP injection (Li and Pu, 2011). The results showed that kaempferol could effectively protect dopaminergic neurons by increasing the activity of endogenous antioxidants (such as superoxide dismutase and glutathione peroxidase) and decreasing malondialdehyde levels. These studies confirmed the neuroprotective effects of kaempferol in vitro models. However, the MPTP model primarily simulates the motor symptoms of Parkinson’s disease but does not fully replicate pathological features such as α-synuclein aggregation. Additionally, current research has not compared the efficacy of kaempferol with commonly used neuroprotective drugs. Future studies could consider using α-synuclein overexpressing transgenic mice to better simulate the pathological characteristics of Parkinson’s disease, combined with comparative experiments, to further assess the relative efficacy and application potential of kaempferol in neuroprotection.
Granatin A and Granatin B are two flavonoids predominantly found in pomegranate peel, differing mainly in the type and number of glycosides they contain. Their content in pomegranate peel is relatively low, with yields of 0.003% and 0.013%, respectively, obtained via fractionation techniques such as column chromatography (Lee et al., 2010). Despite structural differences, Granatin A and Granatin B generally exhibit similar bioactivities, primarily in anti-inflammatory and anticancer applications. Research has shown that they selectively inhibit microsomal prostaglandin E synthase-1 expression in non-small cell lung cancer A549 cells, downregulate tumor necrosis factor-α, inducible nitric oxide synthase, and anti-apoptotic factor B-cell lymphoma 2, and induce apoptosis in A549 cells (Toda et al., 2020). Additionally, these metabolites effectively suppress NO production and nitric oxide synthase expression in RAW 264.7 macrophages, thereby exerting anti-inflammatory effects (Lee et al., 2010). These findings highlight the potential applications of Granatin A and Granatin B in anti-inflammatory and anticancer research. However, due to their low yields, this may limit their future application in the biomedical field.
Phenolic acids are an important subclass of polyphenolic metabolites, characterized by the presence of one or more hydroxyl (-OH) and carboxyl (-COOH) groups attached to a benzene ring (Sun and Shahrajabian, 2023; Wani et al., 2023). Protocatechuic acid (PCA), or 3,4-dihydroxybenzoic acid, is one of the main phenolic acids found in pomegranate peel and is also a metabolite of flavonoid metabolites such as quercetin (Azzini et al., 2010; Crozier et al., 2010; Singh et al., 2016). PCA exhibits various bioactivities, including antioxidant, anti-inflammatory, and antibacterial properties, playing an important role in the prevention and treatment of diseases such as cancer, diabetes, and Alzheimer’s disease.
ROS are byproducts of aerobic metabolism and primarily include superoxide anion (O2−), hydrogen peroxide (H2O2), and hydroxyl radical (⋅OH). When ROS become free radicals due to unpaired electrons, if not neutralized by antioxidants in a timely manner, they can cause oxidative damage to biological macromolecules such as lipids, proteins, and DNA. This oxidative damage can lead to cellular dysfunction, aging, and the onset of various diseases (Song et al., 2020). As a natural polyphenolic metabolite, PCA exerts its antioxidant effects through multiple mechanisms. PCA enhances the activity of intracellular antioxidant enzymes, such as superoxide dismutase (SOD) and glutathione peroxidase (GSH-Px), promoting the clearance of ROS and alleviating oxidative stress in cells (Figure 4) (Cadena-Iniguez et al., 2024; Laskar and Mazumder, 2020). PCA also inhibits the Fenton reaction catalyzed by metal ions (e.g., Fe2+, Cu+), reducing the production of hydroxyl radicals and mitigating oxidative stress from the source (Shahidi and Ambigaipalan, 2015). Additionally, compared to the standard antioxidant Trolox, PCA has demonstrated stronger in vitro antioxidant activity in both lipid and aqueous environments (Kakkar and Bais, 2014). Therefore, with its multiple antioxidant mechanisms, PCA serves as an effective natural antioxidant, aiding in the prevention of aging and various diseases related to oxidative stress.
Figure 4. PCA can enhance the activity of SOD and GSH-PX enzymes, thereby playing a regulatory role in maintaining redox balance.
Inflammatory processes are regulated by various cytokines, chemokines, and inflammatory mediators. Pro-inflammatory cytokines such as TNF-α can bind to corresponding receptors and activate signaling pathways like NF-κB and MAPK, which in turn induce the expression of pro-inflammatory factors like IL-1 and IL-6, thereby exacerbating the inflammatory response (Al-Khayri et al., 2022; Hirano, 2021; Ren et al., 2020). In vitro experiments with RAW 264.7 cells, PCA was shown to inhibit the expression of TNF-α by modulating the activation of the NF-κB and MAPK pathways (Min et al., 2010). Wang et al. used AIN-93G diet containing PCA or standard AIN-93G diet to feed apoE knockout mice for 20 weeks (Wang et al., 2010). The animal experiment results showed that the PCA supplement reduced the area of aortic sinus plaques by 42%. PCA exerted anti-inflammatory effects by lowering the expression of Vascular Cell Adhesion Molecule 1 (VCAM-1) and Intercellular Adhesion Molecule 1 (ICAM-1) in the aorta, inhibiting NF-κB activity, and reducing the levels of soluble VCAM-1 and ICAM-1 in plasma, thereby inhibiting the development of atherosclerosis. These findings suggest that PCA, as a natural metabolite, can regulate inflammation-related signaling pathways, showing potential for anti-inflammatory applications and the treatment of inflammation-related diseases.
Among plant-derived active metabolites, antimicrobial potency generally decreases in the order of phenols, aldehydes, ketones, alcohols, ethers, and hydrocarbons, making polyphenolic extracts ideal candidates to replace traditional antimicrobial agents (Nguyen et al., 2018). As a polyphenolic metabolite derived from plants, PCA exhibits significant antibacterial activity, and has been shown to specifically inhibit the growth of Pseudomonas aeruginosa, Listeria monocytogenes, Aeromonas hydrophila, Pasteurella multocida, Escherichia coli, and certain Salmonella strains, while also reducing the pathogenicity of other bacteria such as Proteus mirabilis (Aldulaimi, 2017; Alvarado-Martinez et al., 2020; Bernal-Mercado et al., 2020; López-Romero et al., 2018; Torzewska and Rozalski, 2014). Research has indicated that the antimicrobial mechanism of PCA primarily involves disrupting bacterial membrane integrity, interfering with bacterial metabolism, and inhibiting biofilm formation (Tian L. et al., 2022; Wu et al., 2022; Yuan et al., 2024). Additionally, when used in combination with other antibiotics, PCA demonstrates synergistic effects, further enhancing its antibacterial efficacy (Fifere et al., 2022). These multiple mechanisms make PCA a promising candidate for antimicrobial applications.
Furthermore, PCA also possesses anti-apoptotic and anti-tumor activities. Abdelrahman et al. induced chronic liver injury and hepatic encephalopathy in male BALB/c mice (7–9 weeks old) through intraperitoneal injection of thioacetamide (200 mg/kg, 3 times per week) (Abdelrahman and El-Tanbouly, 2022). Starting from the fourth week, the mice were treated with oral PCA (100 mg/kg and 150 mg/kg). The results showed that PCA significantly restored the normal histological structure of the liver and brain tissues of the treated mice by downregulating the expression of Caspase-3 in the liver and the expression of tumor protein p53 in both the liver and brain, showing a significant anti-apoptotic effect. However, the PCA dosage used in the study was much higher than the normal dietary intake, which creates a gap in the clinical translational application. PCA’s anti-apoptotic mechanisms are closely related to its regulation of the abnormal expression of mitochondrial pathway-related proteins such as Bcl-2-associated X protein and cytochrome c, and its ability to modulate the Nrf2/p62 pathway, reducing oxidative stress and apoptotic signaling in cells (Zhao et al., 2021). In addition to its anti-apoptotic effects, PCA exerts anti-tumor activity through various mechanisms. PCA significantly reduces the expression of matrix metalloproteinase 2 (MMP2) by inhibiting signal transduction in the RhoB/PKCε and Ras/Akt pathways, thereby preventing tumor cell migration and invasion (Cadena-Iniguez et al., 2024; Lin et al., 2011). PCA also inhibits the Heme Oxygenase-1 (HO-1) mediated activation of p21, reducing colorectal cancer cell viability and inducing apoptosis (Acquaviva et al., 2021). Moreover, when combined with anticancer drugs such as 5-fluorouracil, PCA enhances the efficacy of chemotherapy while minimizing drug-induced side effects (Motamedi et al., 2020). Through these various mechanisms, PCA shows promising anti-tumor activity and potential for use in combination with other anticancer drugs.
Gallic Acid is one of the major phenolic acid metabolites in pomegranate, particularly abundant in the peel. The concentration of gallic acid in every 100 g of pomegranate peel is approximately 128 mg (Singh et al., 2023). Gallic acid exhibits various biological properties, with its antioxidant capacity being the most notable (Wianowska and Olszowy-Tomczyk, 2023). For instance, gallic acid is used as a standard antioxidant in the DPPH method (Pyrzynska and Pękal, 2013), and its antioxidant activity has been shown to surpass common antioxidants such as uric acid, water-soluble vitamin C, and ascorbic acid (Antolovich et al., 2002). Methyl gallate, an ester derivative of gallic acid, also exhibits significant antioxidant activity (Liang et al., 2023). Regarding anti-inflammatory activity, gallic acid can inhibit inflammation induced by lipopolysaccharides, including the release of nitric oxide, prostaglandin E2, interleukin-6, and cyclooxygenase-2 (BenSaad et al., 2017; Marinov et al., 2019). Its anti-inflammatory mechanism may involve the suppression of pro-inflammatory signaling pathways such as NF-κB, thereby alleviating inflammatory responses and exerting protective effects (Zhu et al., 2019). In terms of antitumor activity, Sun et al. investigated the effect of gallic acid on the HepG2 and SMMC-7721 human hepatocellular carcinoma (HCC) cell lines (Sun et al., 2016). The results showed that gallic acid effectively inhibited the growth of liver cancer cells, with IC50 values of 28.5 ± 1.6 μg/mL and 22.1 ± 1.4 μg/mL, respectively. Gallic acid suppressed the proliferation of both HepG2 and SMMC-7721 human liver cancer cells in a time- and dose-dependent manner by activating caspase-3 and caspase-9 and promoting the generation of reactive oxygen species. For antibacterial activity, gallic acid can inhibit the growth of pathogenic bacteria such as Escherichia coli, S. aureus, and Campylobacter jejuni by reducing membrane permeability and regulating pH levels (Kang et al., 2008; Sarjit et al., 2015). Moreover, gallic acid demonstrates anti-apoptotic activity by counteracting apoptosis mediators such as 6-hydroxydopamine (Erol-Dayi et al., 2012). Similarly, vanillic acid, which contains a phenolic hydroxyl group, can scavenge ROS and exhibits antioxidant properties (Parvin et al., 2020). Additionally, numerous studies have highlighted the pharmacological potential of vanillic acid in cardiovascular diseases (Khoshnam et al., 2018), inflammatory pain (Calixto-Campos et al., 2015), and asthma (Bai et al., 2019).
Pomegranate peel is rich in bioactive metabolites, exhibiting multiple health benefits, including antioxidant, anti-inflammatory, antibacterial, anti-apoptotic, and anticancer effects. The bioactive substances and biocompatibility of pomegranate peel make it an ideal candidate for the preparation of biomedical materials such as nanodrug carriers and hydrogels, offering promising applications for PPE in drug delivery, tissue repair, and regenerative medicine. Specifically, the antioxidant and anti-inflammatory properties of PPE help mitigate oxidative stress and inflammation caused by biomedical materials during their application, thereby enhancing the stability and therapeutic efficacy of these materials. Furthermore, PPE’s inherent antibacterial activity provides infection resistance to biomedical materials, significantly improving their potential applications in wound healing and infection control. For instance, in the context of tissue engineering scaffolds, PPE can significantly enhance the bioactivity and antioxidant properties of scaffolds, thereby promoting cell adhesion, proliferation, and tissue repair. The bioactive molecules in PPE regulate cellular signaling pathways, effectively improving the inflammatory microenvironment and further accelerating tissue regeneration. Consequently, PPE demonstrates significant advantages in drug delivery, tissue repair, and regenerative medicine, paving the way for the development of novel biomedical materials with multifunctional properties.
Drug carriers can improve therapeutic outcomes by altering the pharmacokinetics and biodistribution of conventional drugs (bare drugs) or by acting as reservoirs and slow-release systems for conventional drugs (Cheng et al., 2021; Guo et al., 2021; Tenchov et al., 2021). To summarize, drug carriers must exhibit good biocompatibility and efficiently and safely deliver drugs to target sites. By providing sustained-release characteristics, these carriers can prolong drug action, reduce dosing frequency, and improve therapeutic outcomes. Nanodrug carriers can be broadly categorized into three types based on the materials used for their preparation: inorganic nanodrug carriers, lipid-based nanodrug carriers, and polymer-based nanodrug carriers.
Inorganic nanodrug carriers are typically made from metals, metal oxides, or carbon-based materials (such as gold nanoparticles, silver nanoparticles, silica nanoparticles, carbon nanotubes, etc.), which often possess unique optical, electrical, and magnetic properties, making them highly advantageous in cancer treatment, imaging, diagnostics, and other fields (Du et al., 2023; Khan et al., 2022; Khizar et al., 2023; Raza et al., 2021; Shang et al., 2022; Song et al., 2021). Taherian et al. prepared nanoparticles BPPE-CCMNPs containing PPE through a two-step precipitation method and evaluated their anticancer capabilities (Taherian et al., 2021). These nanoparticles, with a size of 37 nm, demonstrated stability in biological environments and showed a favorable release profile in tumor-simulating conditions. Cytotoxicity results indicated that these nanoparticles were toxic to cancer cells but non-toxic to normal cells, suggesting better prospects compared to chemotherapy drugs. Additionally, PPE can serve as a reducing agent and stabilizer for the green synthesis of gold and silver nanoparticles, which are used in antibacterial and cancer treatments (Fu et al., 2023; Ghobadi et al., 2024; Khan et al., 2021). Although researchers have developed various PPE-based inorganic nanodrug carriers using different methods, the potential of inorganic materials, particularly in exploring their optical, electrical, and magnetic properties, has not been fully harnessed. For instance, gold and silver nanoparticles hold significant promise in photothermal therapy and imaging. Further research is needed to combine the physical properties of these nanomaterials with the bioactivity of PPE to achieve dual or multiple therapeutic effects.
Lipid-based nanodrug carriers, composed of natural or synthetic lipid molecules (such as phospholipids, cholesterol, etc.), can spontaneously form bilayer structures called liposomes (Liu P. et al., 2022; Nsairat et al., 2022). Lipid-based nanodrug carriers can efficiently encapsulate both hydrophilic and hydrophobic drugs, and they offer high drug-loading capacity and good stability (Albertsen et al., 2022). Okumus et al. used maltodextrin and soybean lecithin to encapsulate PPE, with Zeta potentials of −18.05, −15.51, and −57.6 for PPE and the encapsulated nanoparticles, respectively, indicating that liposomal encapsulation can enhance PPE’s stability (Okumus et al., 2021). Wang et al. prepared lipid carriers containing PPE using a warm microemulsion technique, and the nano-liposomes demonstrated sustained release for 12 h, with strong anti-tyrosinase activity (Tokton et al., 2014). However, despite the advantages of lipid-based nanodrug carriers, they still face challenges related to solubility, stability, and aggregation (Andishmand et al., 2023). Future efforts could focus on optimizing formulations or modifying the surface of nanoparticles to enhance the application potential of PPE in cancer therapy and other fields.
Polymer-based nanodrug carriers are another widely used carrier system, typically made from synthetic or natural polymer materials such as polylactic acid (PLA), polyethylene glycol (PEG), and poly (D,L-lactic-co-glycolic acid) (PLGA) (De et al., 2022; Dou et al., 2020; Du et al., 2020; Ibrahim et al., 2022; Shi et al., 2022). These carriers possess tunable physicochemical properties and excellent biocompatibility, allowing for sustained drug release (Arkaban et al., 2022). Shirode et al. developed PLGA-PEG nanoparticles loaded with PPE (Shirode et al., 2015), while Soltanzadeh et al. employed chitosan nanoparticles to encapsulate PPE (Soltanzadeh et al., 2021), both nanoparticles exhibited good anticancer and antibacterial activity, along with sustained drug release. However, one major advantage of polymer-based nanocarriers is their ability to achieve targeted delivery to specific cells or tissues through surface functionalization (Ghosh and Biswas, 2021; Shi et al., 2021), research on PPE-based polymer nanocarriers remains largely unexplored.
In recent studies, many nano-drug carriers have been prepared using PPE. These studies highlight that PPE’s biocompatibility and bioactivities—such as antibacterial and anticancer properties—endow nano-drug carriers with excellent compatibility and the ability to inhibit bacterial and cancer cell growth. However, these studies still face numerous challenges. Inorganic nano-drug carriers exhibit various properties depending on the materials used. For instance, metallic nanoparticles can be utilized to prepare photothermal and imaging systems for photodynamic therapy (Ouyang et al., 2021; Xing et al., 2018; Zhan et al., 2024), offering unique advantages in cancer treatment. Current research has yet to fully explore and leverage these properties. Combining these physical properties with the bioactivities of PPE could lead to more effective therapies. Additionally, targeting is a critical factor in evaluating the performance of drug carrier systems. Most anticancer nano-drug carriers prepared with PPE rely on the enhanced permeability and retention (EPR) effect for passive targeting, which has limitations, such as uneven distribution within tumor tissues or incomplete drug release (Gawali et al., 2023; Takakura and Takahashi, 2022). Future studies could explore strategies such as ligand or antibody modifications to enhance active targeting, ensuring precise delivery of carriers to target regions and effective release of drugs to maximize PPE’s bioactivities.
Hydrogels are a class of soft polymer materials with a three-dimensional network structure that can absorb large amounts of water or biological fluids while maintaining their shape and structure (Norahan et al., 2023; Qiao et al., 2023; Shao et al., 2023). Due to their good biocompatibility, tunable mechanical properties, and ability to mimic natural tissues, hydrogels are widely used in wound dressings and tissue engineering. The polyphenolic metabolites in PPE have antibacterial and antioxidant properties, making it an ideal additive for the preparation of functional hydrogels.
In terms of wound dressings, hydrogels containing PPE offer significant advantages (Figure 5). First, the polyphenolic metabolites in PPE effectively inhibit bacterial growth, reducing the risk of infection. Ul-Islam et al. developed an antibacterial wound dressing based on bacterial cellulose loaded with PPE (Ul-Islam et al., 2023). This dressing demonstrated good water absorption and retention capabilities, with excellent antibacterial performance, achieving 100% inhibition of S. aureus growth and 50% inhibition of Escherichia coli. Secondly, PPE can accelerate wound healing by regulating signaling pathways. Savekar et al. prepared a hydrogel film from PPE, citric acid, and β-cyclodextrin-carboxymethyl cassava starch, which could prevent wound infection and accelerate the healing process (Savekar et al., 2024). This film is biodegradable and exhibits pH-dependent ellagic acid release within 24 h. The released ellagic acid promotes cell division, proliferation, and migration to the wound site by inhibiting Glycogen Synthase Kinase-3 Beta and Casein Kinase 1, thereby promoting wound healing.
Figure 5. Hydrogels containing PPE exhibit properties such as injectable, antibacterial activity, and drug sustained release, making them suitable for applications in wound healing, brain defect repair, and bone tissue repair.
In the field of regenerative medicine, PPE-based hydrogels also show broad application potential. Hosseini et al. developed an injectable nanocomposite hydrogel based on polyethylene glycol and chitosan for sustained PPE release (Hosseini et al., 2023). This hydrogel can steadily release PPE for up to 360 h and effectively repair bone injuries by promoting osteoblast differentiation. Similarly, Garcia et al. developed a hydrogel for bone tissue repair, loaded with extracts from pomegranate peel, grape seed, and jabuticaba peel. The study showed that only the hydrogel containing PPE could form an inhibition zone around S. aureus, demonstrating significant antibacterial activity (Garcia et al., 2021). In addition to bone tissue repair, Ju et al. prepared an injectable hydrogel using PPE, hyaluronic acid, and collagen for brain defect repair (Ju et al., 2023). This hydrogel could be precisely injected through a 23-gauge needle (inner diameter 337 μm) to adapt to the deep folds of brain tissue and specific defect areas. The loaded PPE not only exhibited ROS scavenging properties but also promoted neuronal regeneration and differentiation, effectively repairing brain tissue defects.
Hydrogels, with their three-dimensional network structure, provide high hydration capacity, effectively maintaining a moist wound environment and promoting healing, making them ideal wound dressings. Hydrogels prepared with PPE can exhibit antibacterial and antioxidant properties, making them highly effective in preventing infections and accelerating wound healing. The polyphenolic metabolites in PPE effectively inhibit bacterial growth, reducing the risk of wound infection, while its antioxidant properties alleviate oxidative stress and promote cell repair. Additionally, PPE can regulate cellular signaling pathways, facilitating cell proliferation and migration to accelerate the wound healing process. However, despite the significant antibacterial and wound-healing effects of PPE-based hydrogels, challenges remain. For example, the mechanical properties and durability of hydrogels can sometimes be weak, which may affect their performance in long-term applications. Future research could explore combining PPE with cross-linking agents such as polyvinyl alcohol or extracellular matrix metabolites to enhance the mechanical properties and stability of hydrogels in complex wound environments (Chen et al., 2024; Morton et al., 2023). Furthermore, improving the controlled release mechanisms and structural design of hydrogels to introduce responsiveness to stimuli such as temperature and pH (Haidari et al., 2021; Yu et al., 2024) could enhance their effectiveness and reliability in long-term treatments.
Tissue engineering scaffolds are three-dimensional porous solid materials that typically possess high mechanical strength. Their main purpose is to provide a temporary physical framework for damaged tissues, facilitating cell attachment, migration, and proliferation through their porous structure, thereby offering the necessary physical support for the growth of new tissue (Arif et al., 2022; Janmohammadi et al., 2023; Zhang et al., 2022). PPE promotes osteoblast differentiation and proliferation, inhibits osteoclast activity, and exhibits significant anti-inflammatory and antibacterial properties, enabling it to accelerate tissue repair and reduce inflammatory responses. With these advantages, PPE has been utilized in the design of tissue engineering scaffolds.
PPE has been shown to promote osteoblast differentiation and proliferation, inhibit osteoclast activity, and exhibit significant anti-inflammatory and antibacterial properties, accelerating bone tissue repair and reducing inflammatory responses (Bahtiar et al., 2014; Kim et al., 2009; Siddiqui and Arshad, 2014; Spilmont et al., 2015). Owing to these advantages, PPE has been widely applied in the design of scaffolds for bone tissue repair (Figure 6). Sadek et al. used electrospinning technology to prepare a tissue engineering scaffold containing PPE, which demonstrated a nearly 96% improvement in antioxidant activity compared to scaffolds without PPE, along with significant enhancements in osteoblast attachment and proliferation (Sadek et al., 2021). Oliveira et al. further evaluated the effect of polymer scaffolds with PPE on repairing mandibular defects in rats (de Oliveira et al., 2024). The experiment involved three groups: no graft, polymer scaffolds with Brazilian palm resin, and polymer scaffolds with PPE. The average percentages of bone volume formed in these groups were 17.17% ± 2.68%, 27.45% ± 1.65%, and 34.07% ± 0.64% (mean ± standard deviation), with the PPE-containing polymer scaffolds showing the best bone repair ability. Additionally, Karabulut et al. used 3D printing technology to create dental membrane scaffolds containing PPE, and their study showed that the addition of PPE significantly enhanced the scaffold’s biocompatibility and antibacterial activity (Karabulut et al., 2023).
Figure 6. Schematic illustration of the preparation of PPE-containing tissue engineering scaffolds using electrospinning technology.
Tissue engineering scaffolds based on PPE have achieved notable results in the field of bone tissue repair, owing to their exceptional bioactivity. Currently, tissue engineering scaffolds have been widely applied in the repair of soft tissue, cardiovascular systems, and skin (Chen et al., 2023; Roacho-Perez et al., 2022; Weems et al., 2021). By incorporating drugs or enabling controlled release of gases (e.g., oxygen, nitrogen, etc.) (Augustine et al., 2023; Li et al., 2020; Mo et al., 2023), these scaffolds can provide more personalized therapeutic effects. Future studies could explore the potential of PPE in repairing other types of tissues and facilitating combination therapies.
In addition to being an important component of nanodrug carriers, hydrogels, and tissue engineering scaffolds, PPE is also widely applied in fields such as skin repair in the form of ointments and films. In skin repair studies, Luo et al. successfully developed a pomegranate polyphenol ointment and applied it to a scarred rat model (Luo et al., 2022). The results showed that the ointment significantly reduced the damaged area on the rats’ skin and decreased the expression levels of proteins such as Alpha-Smooth Muscle Actin, Phosphorylated Extracellular Signal-Regulated Kinase 1/2, Phosphorylated Janus Kinase 2, Phosphorylated Signal Transducer and Activator of Transcription 3, and Phosphorylated Protein Kinase B. These effects effectively alleviated inflammatory responses, inhibited scar formation, and promoted the skin repair process. Similarly, Hayouni et al. prepared a skin repair ointment using PPE, which demonstrated outstanding antioxidant and antibacterial activities. The ointment significantly accelerated wound contraction and epithelialization in guinea pigs and exhibited potent antibacterial effects against P. aeruginosa, S. aureus, and Escherichia coli (Hayouni et al., 2011). Additionally, Barbalinardo et al. combined PPE with natural silk fibroin to create a transparent, flexible, and bioactive film (Barbalinardo et al., 2022). This film, when applied to skin repair, effectively reduced oxidative stress-induced cell damage and promoted skin regeneration and repair. Beyond skin repair, Jebahi et al. developed a medical dressing using PPE for the treatment of gastric ulcers (Jebahi et al., 2022). In a rabbit gastric ulcer model, the dressing exhibited high blood compatibility (with a hemolysis rate of only 0.42%) and significant antibacterial activity against S. aureus and Helicobacter pylori, with inhibition rates of 84.62% and 88.41%, respectively, after 18 h of contact. The dressing effectively reduced the incidence and severity of gastric tissue lesions in rabbits, demonstrating excellent anti-ulcer effects. Furthermore, Silva developed an oral adhesive ointment using PPE to improve canine oral hygiene. This ointment showed significant antibacterial activity against oral microbiota in Labrador dogs (Silva et al., 2020). In summary, PPE, with its excellent antioxidant, antibacterial, and anti-inflammatory properties, provides innovative solutions for skin repair, gastric ulcer treatment, and oral care.
With the continued advancement of biomedical materials research, more natural biomaterials with favorable bioactivity are being developed and applied in emerging fields such as microneedle patches and medical adhesives (Ding et al., 2024; George and Suchithra, 2019; Medlej et al., 2022; Ryall et al., 2022). Although there are currently no studies on the application of PPE in these areas, its natural bioactivity and good biocompatibility suggest tremendous application potential. Future research could focus on further developing PPE for use in microneedle patches, tissue adhesives, and other biomedical materials, opening up new directions for research and application.
PPE is rich in bioactive metabolites such as ellagic acid, phenolic acids, and flavonoids, which possess multiple functions including antioxidant, anti-inflammatory, antimicrobial, anti-apoptotic, and cell regeneration-promoting properties. These qualities present a wide range of potential applications in biomedical fields such as nanoparticle drug delivery systems, hydrogels, and tissue engineering scaffolds. Through various preparation techniques, PPE can be effectively integrated into medical materials, significantly enhancing their biocompatibility and functionality, promoting wound healing and tissue regeneration, and showing potential in anticancer therapy. The combination of natural extracts with modern technologies not only promotes the development of green biomaterials but also provides new avenues for efficient and safe treatments. However, further research is needed to address issues such as long-term stability, precise delivery, and the mechanisms of action of PPE. In the future, its application techniques should be optimized, and its clinical therapeutic potential should be further explored to achieve broader medical applications.
JD: Validation, Writing–original draft, Conceptualization, Writing–review and editing. HW: Data curation, Writing–original draft. LZ: Data curation, Writing–original draft. SW: Data curation, Writing–original draft. XM: Visualization, Writing–original draft. HD: Visualization, Writing–original draft. XZ: Visualization, Writing–original draft. MZ: Funding acquisition, Writing–review and editing. YH: Funding acquisition, Writing–review and editing.
The author(s) declare that financial support was received for the research and/or publication of this article. This research was supported by the Industry-Academia Cooperation for Synergistic Education Project (No. 2410141228), the Horizontal Project of Zaozhuang University (No. HX20240266), the Exploration of the Laws of Characteristic Processing Techniques—Bran Processing (GZY-KJS-2022-051), the Jiangxi Key Laboratory of Inheritance, Innovation and Transformation of Traditional Chinese Medicine Processing (2024SSY07091), the Team for Inheritance and Innovation of Traditional Chinese Medicine Processing Techniques (CXTD22003), the Industrial Chain Collaborative Research Project of Jiangxi Traditional Chinese Medicine Industry Technology Innovation Consortium (20224BBG72001), and the Zaozhuang University Teaching Research and Reform Project.
Author MZ was employed by Lanshu Cosmetics Co., Ltd.
The remaining authors declare that the research was conducted in the absence of any commercial or financial relationships that could be construed as a potential conflict of interest.
The author(s) declare that no Generative AI was used in the creation of this manuscript.
All claims expressed in this article are solely those of the authors and do not necessarily represent those of their affiliated organizations, or those of the publisher, the editors and the reviewers. Any product that may be evaluated in this article, or claim that may be made by its manufacturer, is not guaranteed or endorsed by the publisher.
Abdelrahman, R. S., and El-Tanbouly, G. S. (2022). Protocatechuic acid protects against thioacetamide-induced chronic liver injury and encephalopathy in mice via modulating mTOR, p53 and the IL-6/IL-17/IL-23 immunoinflammatory pathway. Toxicol. Appl. Pharmacol. 440, 115931. doi:10.1016/j.taap.2022.115931
Abuelsaad, A. S., Mohamed, I., Allam, G., and Al-Solumani, A. A. (2013). Antimicrobial and immunomodulating activities of hesperidin and ellagic acid against diarrheic Aeromonas hydrophila in a murine model. Life Sci. 93 (20), 714–722. doi:10.1016/j.lfs.2013.09.019
Acquaviva, R., Tomasello, B., Di Giacomo, C., Santangelo, R., La Mantia, A., Naletova, I., et al. (2021). Protocatechuic acid, a simple plant secondary metabolite, induced apoptosis by promoting oxidative stress through HO-1 downregulation and p21 upregulation in colon cancer cells. Biomolecules 11 (10), 1485. doi:10.3390/biom11101485
Adili, G., Sun, G., Abuduaini, M., Zhao, Y., Abdulla, R., and Aisa, H. A. (2020). Simultaneous determination of loading capacity and selectivity in preparative off-line two-dimensional separation: an application for purification of corilagin from Pomegranate flower extracts. J. Chromatogr. A 1622, 461129. doi:10.1016/j.chroma.2020.461129
Ain, H. B. U., Tufail, T., Bashir, S., Ijaz, N., Hussain, M., Ikram, A., et al. (2023). Nutritional importance and industrial uses of pomegranate peel: a critical review. FOOD Sci. and Nutr. 11 (6), 2589–2598. doi:10.1002/fsn3.3320
Albertsen, C. H., Kulkarni, J. A., Witzigmann, D., Lind, M., Petersson, K., and Simonsen, J. B. (2022). The role of lipid components in lipid nanoparticles for vaccines and gene therapy. Adv. DRUG Deliv. Rev. 188, 114416. doi:10.1016/j.addr.2022.114416
Aldulaimi, O. A. J. P. R. (2017). General overview of phenolics from plant to laboratory, good antibacterials or not. Pharmacogn. Rev. 11 (22), 123. doi:10.4103/phrev.phrev_43_16
Al-Khayri, J. M., Sahana, G. R., Nagella, P., Joseph, B. V., Alessa, F. M., and Al-Mssallem, M. Q. (2022). Flavonoids as potential anti-inflammatory molecules: a review. MOLECULES 27 (9), 2901. doi:10.3390/molecules27092901
Al-Numair, K. S., Chandramohan, G., Veeramani, C., and Alsaif, M. A. (2015). Ameliorative effect of kaempferol, a flavonoid, on oxidative stress in streptozotocin-induced diabetic rats. Redox Rep. 20 (5), 198–209. doi:10.1179/1351000214Y.0000000117
Alvarado-Martinez, Z., Bravo, P., Kennedy, N.-F., Krishna, M., Hussain, S., Young, A. C., et al. (2020). Antimicrobial and antivirulence impacts of phenolics on Salmonella enterica serovar Typhimurium. Antibiotics 9 (10), 668. doi:10.3390/antibiotics9100668
Andishmand, H., Azadmard-damirchi, S., Hamishekar, H., Torbati, M., Kharazmi, M. S., Savage, G. P., et al. (2023). Nano-delivery systems for encapsulation of phenolic compounds from pomegranate peel. Adv. COLLOID INTERFACE Sci. 311, 102833. doi:10.1016/j.cis.2022.102833
Andishmand, H., Masoumi, B., Yousefi, M., Sadeghizadeh-Yazdi, J., Rostami, M., Dolatabadi, J. E. N., et al. (2025). Pharmacodynamics, pharmacokinetics, and cell signaling pathways of pomegranate peel extract. Phytochem. Rev. doi:10.1007/s11101-025-10076-y
Andreux, P. A., Blanco-Bose, W., Ryu, D., Burdet, F., Ibberson, M., Aebischer, P., et al. (2019). The mitophagy activator urolithin A is safe and induces a molecular signature of improved mitochondrial and cellular health in humans. Nat. Metab. 1 (6), 595–603. doi:10.1038/s42255-019-0073-4
Angeles Avila-Galvez, M., Garcia-Villalba, R., Martinez-Diaz, F., Ocana-Castillo, B., Monedero-Saiz, T., Torrecillas-Sanchez, A., et al. (2019). Metabolic profiling of dietary polyphenols and methylxanthines in normal and malignant mammary tissues from breast cancer patients. Mol. Nutr. and FOOD Res. 63 (9), e1801239. doi:10.1002/mnfr.201801239
Antolovich, M., Prenzler, P. D., Patsalides, E., McDonald, S., and Robards, K. (2002). Methods for testing antioxidant activity. Analyst 127 (1), 183–198. doi:10.1039/b009171p
Arif, Z. U., Khalid, M. Y., Noroozi, R., Sadeghianmaryan, A., Jalalvand, M., and Hossain, M. (2022). Recent advances in 3D-printed polylactide and polycaprolactone-based biomaterials for tissue engineering applications. Int. J. Biol. Macromol. 218, 930–968. doi:10.1016/j.ijbiomac.2022.07.140
Arkaban, H., Barani, M., Akbarizadeh, M. R., Chauhan, N. P. S., Jadoun, S., Soltani, M. D., et al. (2022). Polyacrylic acid nanoplatforms: antimicrobial, tissue engineering, and cancer theranostic applications. POLYMERS 14 (6), 1259. doi:10.3390/polym14061259
Arun, K. B., Madhavan, A., Anoopkumar, A. N., Surendhar, A., Kuriakose, L. L., Tiwari, A., et al. (2022). Integrated biorefinery development for pomegranate peel: prospects for the production of fuel, chemicals and bioactive molecules. Bioresour. Technol. 362, 127833. doi:10.1016/j.biortech.2022.127833
Askari, M., and Nikoonahad Lotfabadi, N. J. D. M. (2020). Evaluation of niosomal nano-carriers capabilities on toxicity preservation and delivery of pomegranate peel extract in cell culture conditions. MCF-7 Cell line breast cancer 26 (5), 9–20.
Ateşşahín, A., Çeríbaşi, A. O., Yuce, A., Bulmus, Ö., and Çikim, G. (2007). Role of ellagic acid against cisplatin-induced nephrotoxicity and oxidative stress in rats. Basic Clin. Pharmacol. Toxicol. Appl. Pharmacol. 100 (2), 121–126. doi:10.1111/j.1742-7843.2006.00015.x
Augustine, R., Gezek, M., Bostanci, N. S., Nguyen, A., and Camci-Unal, G. (2023). Oxygen-generating scaffolds: one step closer to the clinical translation of tissue engineered products. Chem. Eng. J. 455, 140783. doi:10.1016/j.cej.2022.140783
Azzini, E., Vitaglione, P., Intorre, F., Napolitano, A., Durazzo, A., Foddai, M. S., et al. (2010). Bioavailability of strawberry antioxidants in human subjects. Br. J. Nutr. 104 (8), 1165–1173. doi:10.1017/S000711451000187X
Baccarin, T., and Lemos-Senna, E. (2017). Potential application of nanoemulsions for skin delivery of pomegranate peel polyphenols. AAPS PHARMSCITECH 18 (8), 3307–3314. doi:10.1208/s12249-017-0818-x
Bahtiar, A., Arifin, S., Razalifha, A., Qomariah, N., Wuyung, P. E., and Arsianti, A. (2014). Polar fraction of Punica granatum L. Peel Extr. increased osteoblast number ovariectomized rat bone.
Bai, F., Fang, L., Hu, H., Yang, Y., Feng, X., and Sun, D. (2019). Vanillic acid mitigates the ovalbumin (OVA)-induced asthma in rat model through prevention of airway inflammation. Biosci. Biotechnol. Biochem. 83 (3), 531–537. doi:10.1080/09168451.2018.1543015
Bai, L., Han, X., Kee, H. J., He, X., Kim, S. H., Jeon, M. J., et al. (2023). Protocatechuic acid prevents isoproterenol-induced heart failure in mice by downregulating kynurenine-3-monooxygenase. J. Cell. Mol. Med. 27 (16), 2290–2307. doi:10.1111/jcmm.17869
Barbalinardo, M., Giannelli, M., Forcini, L., Luppi, B., Donnadio, A., Navacchia, M. L., et al. (2022). Eco-sustainable silk fibroin/pomegranate peel extract film as an innovative green material for skin repair. Int. J. Mol. Sci. 23 (12), 6805. doi:10.3390/ijms23126805
Begley, M., Gahan, C. G., and Hill, C. (2005). The interaction between bacteria and bile. FEMS Microbiol. Rev. 29 (4), 625–651. doi:10.1016/j.femsre.2004.09.003
Benedetti, G., Zabini, F., Tagliavento, L., Meneguzzo, F., Calderone, V., and Testai, L. (2023). An overview of the health benefits, extraction methods and improving the properties of pomegranate. ANTIOXIDANTS 12 (7), 1351. doi:10.3390/antiox12071351
BenSaad, L. A., Kim, K. H., Quah, C. C., Kim, W. R., and Shahimi, M. (2017). Anti-inflammatory potential of ellagic acid, gallic acid and punicalagin A&B isolated from Punica granatum. BMC Complement. Altern. Med. 17, 1–10. doi:10.1186/s12906-017-1555-0
Bernal-Mercado, A. T., Gutierrez-Pacheco, M. M., Encinas-Basurto, D., Mata-Haro, V., Lopez-Zavala, A. A., Islas-Osuna, M. A., et al. (2020). Synergistic mode of action of catechin, vanillic and protocatechuic acids to inhibit the adhesion of uropathogenic Escherichia coli on silicone surfaces. J. Appl. Microbiol. 128 (2), 387–400. doi:10.1111/jam.14472
Bertolo, M. R. V., Martins, V. C. A., Plepis, A. M. G., and Bogusz Junior, S. (2021). Utilization of pomegranate peel waste: natural deep eutectic solvents as a green strategy to recover valuable phenolic compounds. J. Clean. Prod. 327, 129471. doi:10.1016/j.jclepro.2021.129471
Caballero, V., Estevez, M., Tomas-Barberan, F. A., Morcuende, D., Martin, I., and Delgado, J. (2022). Biodegradation of punicalagin into ellagic acid by selected probiotic bacteria: a study of the underlying mechanisms by MS-based proteomics. J. Agric. FOOD Chem. 70 (51), 16273–16285. doi:10.1021/acs.jafc.2c06585
Cadena-Iniguez, J., Santiago-Osorio, E., Sanchez-Flores, N., Salazar-Aguilar, S., Soto-Hernandez, R. M., de la Luz Riviello-Flores, M., et al. (2024). The cancer-protective potential of protocatechuic acid: a narrative review. MOLECULES 29 (7), 1439. doi:10.3390/molecules29071439
Calixto-Campos, C., Carvalho, T. T., Hohmann, M. S., Pinho-Ribeiro, F. A., Fattori, V., Manchope, M. F., et al. (2015). Vanillic acid inhibits inflammatory pain by inhibiting neutrophil recruitment, oxidative stress, cytokine production, and NFκB activation in mice. J. Nat. Prod. 78 (8), 1799–1808. doi:10.1021/acs.jnatprod.5b00246
Cerdá, B., Cerón, J. J., Tomás-Barberán, F. A., and Espín, J. C. (2003a). Repeated oral administration of high doses of the pomegranate ellagitannin punicalagin to rats for 37 days is not toxic. J. Agric. FOOD Chem. 51 (11), 3493–3501. doi:10.1021/jf020842c
Cerdá, B., Llorach, R., Cerón, J. J., Espín, J. C., and Tomás-Barberán, F. A. (2003b). Evaluation of the bioavailability and metabolism in the rat of punicalagin, an antioxidant polyphenol from pomegranate juice. Eur. J. Nutr. 42 (1), 18–28. doi:10.1007/s00394-003-0396-4
Chang, Y.-W., Huang, W.-C., Lin, C.-Y., Wang, W.-H., Hung, L.-C., and Chen, Y.-H. (2019). Tellimagrandin II, a type of plant polyphenol extracted from Trapa bispinosa inhibits antibiotic resistance of drug-resistant Staphylococcus aureus. Int. J. Mol. Sci. 20 (22), 5790. doi:10.3390/ijms20225790
Chen, J., Fan, Y., Dong, G., Zhou, H., Du, R., Tang, X., et al. (2023). Designing biomimetic scaffolds for skin tissue engineering. BIOMATERIALS Sci. 11 (9), 3051–3076. doi:10.1039/d3bm00046j
Chen, Z., Ezzo, M., Zondag, B., Rakhshani, F., Ma, Y., Hinz, B., et al. (2024). Intrafibrillar crosslinking enables decoupling of mechanical properties and structure of a composite fibrous hydrogel. Adv. Mater. 36 (2), e2305964. doi:10.1002/adma.202305964
Cheng, A.-W., Tan, X., Sun, J.-Y., Gu, C.-M., Liu, C., and Guo, X. (2019). Catechin attenuates TNF-α induced inflammatory response via AMPK-SIRT1 pathway in 3T3-L1 adipocytes. PLOS ONE 14 (5), e0217090. doi:10.1371/journal.pone.0217090
Cheng, H. Y., Lin, C. C., and Lin, T. C. (2002). Antiherpes simplex virus type 2 activity of casuarinin from the bark of Terminalia arjuna Linn. Antivir. Res. 55 (3), 447–455. doi:10.1016/S0166-3542(02)00077-3
Cheng, Z., Li, M., Dey, R., and Chen, Y. (2021). Nanomaterials for cancer therapy: current progress and perspectives. J. Hematol. and Oncol. 14 (1), 85. doi:10.1186/s13045-021-01096-0
Cortes-Martin, A., Iglesias-Aguirre, C. E., Marin, A., Romo-Vaquero, M., Vallejo, F., Espin, J. C., et al. (2024). Urolithin A production drives the effects of pomegranate on the gut microbial metabolism of bile acids and cholesterol in mild dyslipidaemic overweight and obese individuals. FOOD and Funct. 15 (5), 2422–2432. doi:10.1039/d3fo05014a
Crozier, A., Del Rio, D., and Clifford, M. N. (2010). Bioavailability of dietary flavonoids and phenolic compounds. Mol. ASPECTS Med. 31 (6), 446–467. doi:10.1016/j.mam.2010.09.007
Cuevas-Magana, M. Y., Vega-Garcia, C. C., Leon-Contreras, J. C., Hernandez-Pando, R., Zazueta, C., and Garcia-Nino, W. R. (2022). Ellagic acid ameliorates hexavalent chromium-induced renal toxicity by attenuating oxidative stress, suppressing TNF-α and protecting mitochondria. Toxicol. Appl. Pharmacol. 454, 116242. doi:10.1016/j.taap.2022.116242
De, R., Mahata, M. K., and Kim, K.-T. (2022). Structure-based varieties of polymeric nanocarriers and influences of their physicochemical properties on drug delivery profiles. Adv. Sci. 9 (10), e2105373. doi:10.1002/advs.202105373
De, R., Sarkar, A., Ghosh, P., Ganguly, M., Karmakar, B. C., Saha, D. R., et al. (2018). Antimicrobial activity of ellagic acid against Helicobacter pylori isolates from India and during infections in mice. J. Antimicrob. Chemother. 73 (6), 1595–1603. doi:10.1093/jac/dky079
de Oliveira, B. E. G., Maia, F. L. M., Massimino, L. C., Garcia, C. F., Plepis, A. M. D., Martins, V. D. A., et al. (2024). Use of plant extracts in polymeric scaffolds in the regeneration of mandibular injuries. PHARMACEUTICS 16 (4), 491. doi:10.3390/pharmaceutics16040491
Derakhshan, Z., Ferrante, M., Tadi, M., Ansari, F., Heydari, A., Hosseini, M. S., et al. (2018). Antioxidant activity and total phenolic content of ethanolic extract of pomegranate peels, juice and seeds. FOOD Chem. Toxicol. 114, 108–111. doi:10.1016/j.fct.2018.02.023
Ding, W., Shao, X., Ding, S., Du, Y., Hong, W., Yang, Q., et al. (2024). Natural herb wormwood-based microneedle array for wound healing. DRUG Deliv. Transl. Res. 14 (9), 2461–2473. doi:10.1007/s13346-024-01520-1
Dou, J., Wu, Q., Li, Y. M., Du, J. S., Wan, X. Z., Han, X., et al. (2020). Keratin-Poly(2-methacryloxyethyl phosphatidylcholine) conjugate-based micelles as a tumor micro-environment-responsive drug-delivery system with long blood circulation. LANGMUIR 36 (13), 3540–3549. doi:10.1021/acs.langmuir.0c00044
Du, J. S., Chen, G. P., Yuan, X. Y., Yuan, J., and Li, L. (2023). Multi-stimuli responsive Cu-MOFs@Keratin drug delivery system for chemodynamic therapy. Front. Bioeng. Biotechnol. 11, 1125348. doi:10.3389/fbioe.2023.1125348
Du, J. S., Wu, Q., Li, Y. M., Liu, P. C., Han, X., Wang, L. J., et al. (2020). Preparation and characterization of Keratin-PEG conjugate-based micelles as a tumor microenvironment-responsive drug delivery system. J. BIOMATERIALS SCIENCE-POLYMER Ed. 31 (9), 1163–1178. doi:10.1080/09205063.2020.1747044
Duan, H., Wang, F., Wang, K., Yang, S., Zhang, R., Xue, C., et al. (2024). Quercetin ameliorates oxidative stress-induced apoptosis of granulosa cells in dairy cow follicular cysts by activating autophagy via the SIRT1/ROS/AMPK signaling pathway. J. Animal Sci. Biotechnol. Lett. 15 (1), 119. doi:10.1186/s40104-024-01078-5
Erol-Dayi, Ö., Arda, N., and Erdem, G. (2012). Protective effects of olive oil phenolics and gallic acid on hydrogen peroxide-induced apoptosis. Eur. J. Nutr. 51, 955–960. doi:10.1007/s00394-011-0273-5
Evtyugin, D. D., Magina, S., and Evtuguin, D. V. (2020). Recent advances in the production and applications of ellagic acid and its derivatives. A review. MOLECULES 25 (12), 2745. doi:10.3390/molecules25122745
Fifere, A., Turin-Moleavin, I.-A., and Rosca, I. (2022). Does protocatechuic acid affect the activity of commonly used antibiotics and antifungals? LIFE-BASEL 12 (7), 1010. doi:10.3390/life12071010
Flores-Maldonado, O., Lezcano-Domínguez, C. I., Dávila-Aviña, J., González, G. M., and Ríos-López, A. L. (2024). Methyl gallate attenuates virulence and decreases antibiotic resistance in extensively drug-resistant Pseudomonas aeruginosa. Microb. Pathog. 194, 106830. doi:10.1016/j.micpath.2024.106830
Fu, Y., Li, J., and Almasi, M. (2023). Pomegranate peel extract-mediated green synthesis of silver nanoparticles: evaluation of cytotoxicity, antioxidant, and anti-esophageal cancer effects. CHEMISTRYSELECT 8 (15). doi:10.1002/slct.202204841
Ganeshkumar, M., Sathishkumar, M., Ponrasu, T., Dinesh, M. G., and Suguna, L. (2013). Spontaneous ultra fast synthesis of gold nanoparticles using Punica granatum for cancer targeted drug delivery. COLLOIDS SURFACES B-BIOINTERFACES 106, 208–216. doi:10.1016/j.colsurfb.2013.01.035
Gao, J., Hua, Q., Chen, L., Zhang, J., Zhao, H., Meng, X., et al. (2024). Punicalagin prevents the bone loss of diabetic mice induced by high-fat diet via the metabolism of gut microbiota. eFood 5 (5), e186. doi:10.1002/efd2.186
Garcia, C. F., Marangon, C. A., Massimino, L. C., Klingbeil, M. F. G., Martins, V. C. A., and Plepis, A. M. D. (2021). Development of collagen/nanohydroxyapatite scaffolds containing plant extract intended for bone regeneration. Mater. Sci. Eng. C-MATERIALS Biol. Appl. 123, 111955. doi:10.1016/j.msec.2021.111955
Gawali, P., Saraswat, A., Bhide, S., Gupta, S., and Patel, K. (2023). Human solid tumors and clinical relevance of the enhanced permeation and retention effect: a 'golden gate' for nanomedicine in preclinical studies? NANOMEDICINE 18 (2), 169–190. doi:10.2217/nnm-2022-0257
Gaya, P., Peirotén, Á., Medina, M., Álvarez, I., and Landete, J. M. (2018). Bifidobacterium pseudocatenulatum INIA P815: the first bacterium able to produce urolithins A and B from ellagic acid. J. Funct. FOODS 45, 95–99. doi:10.1016/j.jff.2018.03.040
Ge, S., Duo, L., Wang, J., Gegen, Z., Yang, J., Li, Z., et al. (2021). A unique understanding of traditional medicine of pomegranate, Punica granatum L. and its current research status. J. Ethnopharmacol. 271, 113877. doi:10.1016/j.jep.2021.113877
George, B., and Suchithra, T. V. (2019). Plant-derived bioadhesives for wound dressing and drug delivery system. FITOTERAPIA 137, 104241. doi:10.1016/j.fitote.2019.104241
Ghobadi, M., Salehi, S., Ardestani, M. T. S., Mousavi-Khattat, M., Shakeran, Z., Khosravi, A., et al. (2024). Amine-functionalized mesoporous silica nanoparticles decorated by silver nanoparticles for delivery of doxorubicin in breast and cervical cancer cells. Eur. J. Pharm. Biopharm. 201, 114349. doi:10.1016/j.ejpb.2024.114349
Ghosh, B., and Biswas, S. (2021). Polymeric micelles in cancer therapy: state of the art. J. Control. RELEASE 332, 127–147. doi:10.1016/j.jconrel.2021.02.016
Giri, N. A., Gaikwad, N. N., Raigond, P., Damale, R., and Marathe, R. A. (2023). Exploring the potential of pomegranate peel extract as a natural food additive: a review. Curr. Nutr. Rep. 12 (2), 270–289. doi:10.1007/s13668-023-00466-z
Gong, J.-H., Shin, D., Han, S.-Y., Park, S.-H., Kang, M.-K., Kim, J.-L., et al. (2013). Blockade of airway inflammation by kaempferol via disturbing Tyk-STAT signaling in airway epithelial cells and in asthmatic mice. Evidence-based Complement. Altern. Med. 2013 (1), 250725. doi:10.1155/2013/250725
Gu, L., Deng, W.-s., Liu, Y., Jiang, C.-h., Sun, L.-c., Sun, X.-f., et al. (2014). Ellagic acid protects Lipopolysaccharide/D-galactosamine-induced acute hepatic injury in mice. Int. Immunopharmacol. 22 (2), 341–345. doi:10.1016/j.intimp.2014.07.005
Guo, Y., Sun, Q., Wu, F.-G., Dai, Y., and Chen, X. (2021). Polyphenol-containing nanoparticles: synthesis, properties, and therapeutic delivery. Adv. Mater. 33 (22), e2007356. doi:10.1002/adma.202007356
Gupta, A., Singh, A. K., Kumar, R., Ganguly, R., Rana, H. K., Pandey, P. K., et al. (2019). Corilagin in cancer: a critical evaluation of anticancer activities and molecular mechanisms. MOLECULES 24 (18), 3399. doi:10.3390/molecules24183399
Haidari, H., Kopecki, Z., Sutton, A. T., Garg, S., Cowin, A. J., and Vasilev, K. (2021). pH-Responsive smart hydrogel for controlled delivery of silver nanoparticles to infected wounds. ANTIBIOTICS-BASEL 10 (1), 49. doi:10.3390/antibiotics10010049
Han, X., Sun, S., Sun, Y., Song, Q., Zhu, J., Song, N., et al. (2019). Small molecule-driven NLRP3 inflammation inhibition via interplay between ubiquitination and autophagy: implications for Parkinson disease. Autophagy 15 (11), 1860–1881. doi:10.1080/15548627.2019.1596481
Hanafy, S. M., Abd El-Shafea, Y. M., Saleh, W. D., and Fathy, H. M. (2021). Chemical profiling, in vitro antimicrobial and antioxidant activities of pomegranate, orange and banana peel-extracts against pathogenic microorganisms. J. Genet. Eng. Biotechnol. Lett. 19 (1), 80. doi:10.1186/s43141-021-00151-0
Hara-Kudo, Y., Yamasaki, A., Sasaki, M., Okubo, T., Minai, Y., Haga, M., et al. (2005). Antibacterial action on pathogenic bacterial spore by green tea catechins. J. Sci. Food Agric. 85 (14), 2354–2361. doi:10.1002/jsfa.2259
Hayouni, E. A., Miled, K., Boubaker, S., Bellasfar, Z., Abedrabba, M., Iwaski, H., et al. (2011). Hydroalcoholic extract based-ointment from Punica granatum L. peels with enhanced in vivo healing potential on dermal wounds. PHYTOMEDICINE 18 (11), 976–984. doi:10.1016/j.phymed.2011.02.011
Henning, S. M., Yang, J., Lee, R.-P., Huang, J., Thames, G., Korn, M., et al. (2022). Pomegranate juice alters the microbiota in breast milk and infant stool: a pilot study. FOOD and Funct. 13 (10), 5680–5689. doi:10.1039/d2fo00280a
Hirano, T. (2021). IL-6 in inflammation, autoimmunity and cancer. Int. Immunol. 33 (3), 127–148. doi:10.1093/intimm/dxaa078
Hosseini, S., Eslahi, N., and Jahanmardi, R. (2023). Self-healing nanocomposite hydrogels based on chitosan/modified polyethylene glycol/graphene. Mater. TODAY Commun. 37, 107417. doi:10.1016/j.mtcomm.2023.107417
Huang, M., Wu, K., Zeng, S., Liu, W., Cui, T., Chen, Z., et al. (2021). Punicalagin inhibited inflammation and migration of fibroblast-like synoviocytes through NF-κB pathway in the experimental study of rheumatoid arthritis. J. Inflamm. Res. 14, 1901–1913. doi:10.2147/JIR.S302929
Hussain, M. S., Altamimi, A. S. A., Afzal, M., Almalki, W. H., Kazmi, I., Alzarea, S. I., et al. (2024). Kaempferol: paving the path for advanced treatments in aging-related diseases. Exp. Gerontol. 188, 112389. doi:10.1016/j.exger.2024.112389
Huwait, E., Ayoub, M., and Karim, S. (2022). Investigation of the molecular mechanisms underlying the antiatherogenic actions of kaempferol in human THP-1 macrophages. Int. J. Mol. Sci. 23 (13), 7461. doi:10.3390/ijms23137461
Ibrahim, M., Ramadan, E., Elsadek, N. E., Emam, S. E., Shimizu, T., Ando, H., et al. (2022). Polyethylene glycol (PEG): the nature, immunogenicity, and role in the hypersensitivity of PEGylated products. J. Control. RELEASE 351, 215–230. doi:10.1016/j.jconrel.2022.09.031
Ismail, T., Calcabrini, C., Diaz, A. R., Fimognari, C., Turrini, E., Catanzaro, E., et al. (2016). Ellagitannins in cancer chemoprevention and therapy. Toxins 8 (5), 151. doi:10.3390/toxins8050151
Janmohammadi, M., Nazemi, Z., Salehi, A. O. M., Seyfoori, A., John, J., Nourbakhsh, M. S., et al. (2023). Cellulose-based composite scaffolds for bone tissue engineering and localized drug delivery. Bioact. Mater. 20, 137–163. doi:10.1016/j.bioactmat.2022.05.018
Jebahi, S., Ben Salah, G., Jarray, S., Naffati, M., Ahmad, M. A., Brahmi, F., et al. (2022). Chitosan-based gastric dressing materials loaded with pomegranate peel as bioactive agents: pharmacokinetics and effects on experimentally induced gastric ulcers in rabbits. METABOLITES 12 (12), 1158. doi:10.3390/metabo12121158
Ju, J., Kim, S. D., and Shin, M. (2023). Pomegranate polyphenol-derived injectable therapeutic hydrogels to enhance neuronal regeneration. Mol. Pharm. 20 (9), 4786–4795. doi:10.1021/acs.molpharmaceut.3c00623
Kakkar, S., and Bais, S. (2014). A review on protocatechuic Acid and its pharmacological potential. ISRN Pharmacol. 2014, 952943. doi:10.1155/2014/952943
Kang, M.-S., Oh, J.-S., Kang, I.-C., Hong, S.-J., and Choi, C.-H. (2008). Inhibitory effect of methyl gallate and gallic acid on oral bacteria. J. Microbiol. 46, 744–750. doi:10.1007/s12275-008-0235-7
Karabulut, H., Ulag, S., Dalbayrak, B., Arisan, E. D., Taskin, T., Guncu, M. M., et al. (2023). A novel approach for the fabrication of 3D-printed dental membrane scaffolds including antimicrobial pomegranate extract. PHARMACEUTICS 15 (3), 737. doi:10.3390/pharmaceutics15030737
Khan, A. A., Alanazi, A. M., Alsaif, N., Wani, T. A., and Bhat, M. A. (2021). Pomegranate peel induced biogenic synthesis of silver nanoparticles and their multifaceted potential against intracellular pathogen and cancer. SAUDI J. Biol. Sci. 28 (8), 4191–4200. doi:10.1016/j.sjbs.2021.06.022
Khan, J., Masood, A., Noor, A., and Qadir, M. I. (2017). Drug development for PCGF2 protein, involved in formation of human breast cancer by polyphenols and their derivatives extracted from Cowania maxicana. Chemistry 3 (6), 77–81. doi:10.11648/j.ijpc.20170306.12
Khan, M. I., Hossain, M. I., Hossain, M. K., Rubel, M. H. K., Hossain, K. M., Mahfuz, A. M. U. B., et al. (2022). Recent progress in nanostructured smart drug delivery systems for cancer therapy: a review. ACS Appl. BIO Mater. 5 (3), 971–1012. doi:10.1021/acsabm.2c00002
Khizar, S., Alrushaid, N., Khan, F. A., Zine, N., Jaffrezic-Renault, N., Errachid, A., et al. (2023). Nanocarriers based novel and effective drug delivery system. Int. J. Pharm. 632, 122570. doi:10.1016/j.ijpharm.2022.122570
Khoshnam, S. E., Sarkaki, A., Rashno, M., and Farbood, Y. (2018). Memory deficits and hippocampal inflammation in cerebral hypoperfusion and reperfusion in male rats: neuroprotective role of vanillic acid. Life Sci. 211, 126–132. doi:10.1016/j.lfs.2018.08.065
Kim, Y. H., Choi, E., and Derivatives, T. E. o. N. P. (2009). Stimulation of osteoblastic differentiation and inhibition of interleukin-6 and nitric oxide in MC3T3-E1 cells by pomegranate ethanol extract. Phytother. Res. 23 (5), 737–739. doi:10.1002/ptr.2587
Kujawska, M., Jourdes, M., Kurpik, M., Szulc, M., Szaefer, H., Chmielarz, P., et al. (2020). Neuroprotective effects of pomegranate juice against Parkinson's disease and presence of ellagitannins-derived metabolite-urolithin A-in the brain. Int. J. Mol. Sci. 21 (1), 202. doi:10.3390/ijms21010202
Kujawska, M., Jourdes, M., Witucki, L., Karazniewicz-Lada, M., Szulc, M., Gorska, A., et al. (2021). Pomegranate juice ameliorates dopamine release and behavioral deficits in a rat model of Parkinson's disease. Brain Sci. 11 (9), 1127. doi:10.3390/brainsci11091127
Kumar, N., Pratibha, N., Sami, R., Khojah, E., Aljahani, A. H., Al-Mushhin, A. A. M., et al. (2022). Effects of drying methods and solvent extraction on quantification of major bioactive compounds in pomegranate peel waste using HPLC. Sci. Rep. 12 (1), 8000. doi:10.1038/s41598-022-11881-7
Kumari, S., Kamboj, A., Wanjari, M., and Sharma, A. K. (2021). Nephroprotective effect of Vanillic acid in STZ-induced diabetic rats. J. Diabetes Metabolic Disord. 20, 571–582. doi:10.1007/s40200-021-00782-7
Langgut, D. (2024). The core area of fruit-tree cultivation: central Jordan Valley (Levant), ca. 7000 BP. PALYNOLOGY 48. doi:10.1080/01916122.2024.2347905
Larrosa, M., Gonzalez-Sarrias, A., Yanez-Gascon, M. J., Selma, M. V., Azorin-Ortuno, M., Toti, S., et al. (2010). Anti-inflammatory properties of a pomegranate extract and its metabolite urolithin-A in a colitis rat model and the effect of colon inflammation on phenolic metabolism. J. Nutr. Biochem. 21 (8), 717–725. doi:10.1016/j.jnutbio.2009.04.012
Laskar, Y. B., and Mazumder, P. B. (2020). Insight into the molecular evidence supporting the remarkable chemotherapeutic potential of Hibiscus sabdariffa L. Biomed. Pharmacother. 127, 110153. doi:10.1016/j.biopha.2020.110153
Lee, C.-J., Chen, L.-G., Liang, W.-L., and Wang, C.-C. (2010). Anti-inflammatory effects of punica granatum linne in vitro and in vivo. FOOD Chem. 118 (2), 315–322. doi:10.1016/j.foodchem.2009.04.123
Li, B., Weng, Q., Liu, Z., Shen, M., Zhang, J., Wu, W., et al. (2017). Selection of antioxidants against ovarian oxidative stress in mouse model. J. Biochem. Mol. Toxicol. 31 (12), e21997. doi:10.1002/jbt.21997
Li, J., Huang, H., Feng, M., Zhou, W., Shi, X., and Zhou, P. (2008). In vitro and in vivo anti-hepatitis B virus activities of a plant extract from Geranium carolinianum L. Antivir. Res. 79 (2), 114–120. doi:10.1016/j.antiviral.2008.03.001
Li, P., Wang, Y., Jin, X., Dou, J., Han, X., Wan, X., et al. (2020). Fabrication of PCL/keratin composite scaffolds for vascular tissue engineering with catalytic generation of nitric oxide potential. J. Mater. Chem. B 8 (28), 6092–6099. doi:10.1039/d0tb00857e
Li, S., and Pu, X.-P. (2011). Neuroprotective effect of kaempferol against a 1-methyl-4-phenyl-1, 2, 3, 6-tetrahydropyridine-induced mouse model of Parkinson's disease. Biol. Pharm. Bull. 34 (8), 1291–1296. doi:10.1248/bpb.34.1291
Li, X., Deng, Y., Zheng, Z., Huang, W., Chen, L., Tong, Q., et al. (2018). Corilagin, a promising medicinal herbal agent. Biomed. and Pharmacother. 99, 43–50. doi:10.1016/j.biopha.2018.01.030
Li, Y. F., Guo, C. J., Yang, J. J., Wei, J. Y., Xu, J., and Cheng, S. (2006). Evaluation of antioxidant properties of pomegranate peel extract in comparison with pomegranate pulp extract. FOOD Chem. 96 (2), 254–260. doi:10.1016/j.foodchem.2005.02.033
Liang, H., Huang, Q., Zou, L., Wei, P., Lu, J., and Zhang, Y. (2023). Methyl gallate: review of pharmacological activity. Pharmacol. Res. 194, 106849. doi:10.1016/j.phrs.2023.106849
Lin, H. H., Chen, J. H., Chou, F. P., and Wang, C. (2011). Protocatechuic acid inhibits cancer cell metastasis involving the down-regulation of Ras/Akt/NF-κB pathway and MMP-2 production by targeting RhoB activation. Br. J. Pharmacol. 162 (1), 237–254. doi:10.1111/j.1476-5381.2010.01022.x
Lin, R., Piao, M., and Song, Y. (2019). Dietary quercetin increases colonic microbial diversity and attenuates colitis severity in citrobacter rodentium-infected mice. Front. Microbiol. 10, 1092. doi:10.3389/fmicb.2019.01092
Liu, F. C., Liao, C.-C., Lee, H.-C., Chou, A.-H., and Yu, H.-P. (2022). Effects of corilagin on lipopolysaccharide-induced acute lung injury via regulation of NADPH oxidase 2 and ERK/NF-κ B signaling pathways in a mouse model. Biology 11 (7), 1058. doi:10.3390/biology11071058
Liu, G., Xu, X., Gong, Y., He, L., and Gao, Y. (2012). Effects of supercritical CO2 extraction parameters on chemical composition and free radical-scavenging activity of pomegranate (Punica granatum L.) seed oil. FOOD Bioprod. Process. 90 (C3), 573–578. doi:10.1016/j.fbp.2011.11.004
Liu, H., Yan, C., Teng, Y., Guo, J., Liang, C., and Xia, X. (2024). Gut microbiota and D-ribose mediate the anti-colitic effect of punicalagin in DSS-treated mice. FOOD and Funct. 15 (13), 7108–7123. doi:10.1039/d4fo00741g
Liu, P., Chen, G., and Zhang, J. (2022). A review of liposomes as a drug delivery system: current status of approved products, regulatory environments, and future perspectives. MOLECULES 27 (4), 1372. doi:10.3390/molecules27041372
Liu, P., Wang, W., Li, Q., Hu, X., Xu, B., Wu, C., et al. (2021). Methyl gallate improves hyperuricemia nephropathy mice through inhibiting NLRP3 pathway. Front. Pharmacol. 12, 759040. doi:10.3389/fphar.2021.759040
Lloyd-Price, J., Arze, C., Ananthakrishnan, A. N., Schirmer, M., Avila-Pacheco, J., Poon, T. W., et al. (2019). Multi-omics of the gut microbial ecosystem in inflammatory bowel diseases. Nature 569 (7758), 655–662. doi:10.1038/s41586-019-1237-9
Longtin, R. (2003). The pomegranate: nature’s power fruit? JNCI J. Natl. Cancer Inst. 95 (5), 346–348. doi:10.1093/jnci/95.5.346
López-Romero, J. C., Valenzuela-Melendres, M., Juneja, V. K., García-Dávila, J., Camou, J. P., Peña-Ramos, A., et al. (2018). Effects and interactions of gallic acid, eugenol and temperature on thermal inactivation of Salmonella spp. in ground chicken. Food Res. Int. 103, 289–294. doi:10.1016/j.foodres.2017.10.055
Luo, J., Long, Y., Ren, G., Zhang, Y., Chen, J., Huang, R., et al. (2019). Punicalagin reversed the hepatic injury of Tetrachloromethane by Antioxidation and enhancement of autophagy. J. Med. food 22 (12), 1271–1279. doi:10.1089/jmf.2019.4411
Luo, L. N., Du, J., Zhao, C. C., and Hu, X. G. (2022). Pomegranate peel polyphenol ointment plays an anti-scar role by regulating the erkl-2 and jak2-stat3-akt pathways. ACTA MEDICA Mediterr. 38 (2), 813–818. doi:10.19193/0393-6384_2022_2_123
Lyu, A., Chen, J.-j., Wang, H.-c., Yu, X.-h., Zhang, Z.-c., Gong, P., et al. (2017). Punicalagin protects bovine endometrial epithelial cells against lipopolysaccharide-induced inflammatory injury. J. Zhejiang Univ. Sci. B 18 (6), 481–491. doi:10.1631/jzus.B1600224
Magangana, T. P., Makunga, N. P., Fawole, O. A., and Opara, U. L. (2020). Processing factors affecting the phytochemical and nutritional properties of pomegranate punica granatum L. peel waste: a review. MOLECULES 25 (20), 4690. doi:10.3390/molecules25204690
Manach, C., Morand, C., Texier, O., Favier, M.-L., Agullo, G., Demigné, C., et al. (1995). Quercetin metabolites in plasma of rats fed diets containing rutin or quercetin. J. Nutr. 125 (7), 1911–1922. doi:10.1093/jn/125.7.1911
Manikandan, R., Beulaja, M., Arulvasu, C., Sellamuthu, S., Dinesh, D., Prabhu, D., et al. (2012). Synergistic anticancer activity of curcumin and catechin: an in vitro study using human cancer cell lines. Microsc. Res. Tech. 75 (2), 112–116. doi:10.1002/jemt.21032
Marinov, V., Tzaneva, M., Zhelyazkova-Savova, M., Gancheva, S., and Valcheva-Kuzmanova, S. (2019). Effects of gallic acid in a rat model of inflammatory bowel disease induced by trinitrobenzenesulfonic acid. Bulg. Chem. Commun. 51, 22–28.
Medlej, M. K., Le Floch, S., Nasser, G., Li, S., Hijazi, A., and Pochat-Bohatier, C. (2022). Correlations between rheological and mechanical properties of fructo-polysaccharides extracted from Ornithogalum billardieri as biobased adhesive for biomedical applications. Int. J. Biol. Macromol. 209, 1100–1110. doi:10.1016/j.ijbiomac.2022.04.106
Melgarejo-Sánchez, P., Núñez-Gómez, D., Martínez-Nicolás, J. J., Hernández, F., Legua, P., and Melgarejo, P. (2021). Pomegranate variety and pomegranate plant part, relevance from bioactive point of view: a review. Bioresour. Bioprocess. 8, 1–29. doi:10.1186/s40643-020-00351-5
Min, S.-W., Ryu, S.-N., and Kim, D.-H. (2010). Anti-inflammatory effects of black rice, cyanidin-3-O-β-D-glycoside, and its metabolites, cyanidin and protocatechuic acid. Int. Immunopharmacol. 10 (8), 959–966. doi:10.1016/j.intimp.2010.05.009
Min, Z., Yangchun, L., Yuquan, W., and Changying, Z. (2019). Quercetin inhibition of myocardial fibrosis through regulating MAPK signaling pathway via ROS. Pak. J. Pharm. Sci. 32, 1355–1359.
Mo, J., Panichayupakaranant, P., Kaewnopparat, N., Nitiruangjaras, A., and Reanmongkol, W. (2013). Topical anti-inflammatory and analgesic activities of standardized pomegranate rind extract in comparison with its marker compound ellagic acid in vivo. J. Ethnopharmacol. 148 (3), 901–908. doi:10.1016/j.jep.2013.05.040
Mo, X., Zhang, D., Liu, K., Zhao, X., Li, X., and Wang, W. (2023). Nano-hydroxyapatite composite scaffolds loaded with bioactive factors and drugs for bone tissue engineering. Int. J. Mol. Sci. 24 (2), 1291. doi:10.3390/ijms24021291
Molnar, M., Kovac, M. J., and Pavic, V. (2024). A comprehensive analysis of diversity, structure, biosynthesis and extraction of biologically active tannins from various plant-based materials using deep eutectic solvents. MOLECULES 29 (11), 2615. doi:10.3390/molecules29112615
Morton, L. D., Castilla-Casadiego, D. A., Palmer, A. C., and Rosales, A. M. (2023). Crosslinker structure modulates bulk mechanical properties and dictates hMSC behavior on hyaluronic acid hydrogels. ACTA BIOMATER. 155, 258–270. doi:10.1016/j.actbio.2022.11.027
Motamedi, Z., Amini, S. A., Raeisi, E., Lemoigne, Y., and Heidarian, E. (2020). Combined effects of protocatechuic acid and 5-fluorouracil on p53 gene expression and apoptosis in gastric adenocarcinoma cells. Turkish J. Pharm. Sci. 17 (6), 578–585. doi:10.4274/tjps.galenos.2019.69335
Nguyen, H. V., Meile, J. C., Lebrun, M., Caruso, D., Chu-Ky, S., and Sarter, S. (2018). Litsea cubeba leaf essential oil from Vietnam: chemical diversity and its impacts on antibacterial activity. Lett. Appl. Microbiol. 66 (3), 207–214. doi:10.1111/lam.12837
Nguyen-Ngo, C., Willcox, J. C., and Lappas, M. (2020). Anti-inflammatory effects of phenolic acids punicalagin and curcumin in human placenta and adipose tissue. PLACENTA 100, 1–12. doi:10.1016/j.placenta.2020.08.002
Nijveldt, R. J., Van Nood, E., Van Hoorn, D. E., Boelens, P. G., Van Norren, K., and Van Leeuwen, P. A. (2001). Flavonoids: a review of probable mechanisms of action and potential applications. Am. J. Clin. Nutr. 74 (4), 418–425. doi:10.1093/ajcn/74.4.418
Norahan, M. H., Pedroza-Gonz, S. C., Sanchez-Salazar, M. G., Alvarez, M. M., and Santiago, G. T. D. (2023). Structural and biological engineering of 3D hydrogels for wound healing. Bioact. Mater. 24, 197–235. doi:10.1016/j.bioactmat.2022.11.019
Nsairat, H., Khater, D., Sayed, U., Odeh, F., Al Bawab, A., and Alshaer, W. (2022). Liposomes: structure, composition, types, and clinical applications. HELIYON 8 (5), e09394. doi:10.1016/j.heliyon.2022.e09394
Nuncio-Jáuregui, N., Munera-Picazo, S., Calín-Sánchez, Á., Wojdyło, A., Hernández, F., and Carbonell-Barrachina, Á. A. (2015). Bioactive compound composition of pomegranate fruits removed during thinning. J. Food Compos. Analysis 37, 11–19. doi:10.1016/j.jfca.2014.06.015
Okumus, E., Bakkalbasi, E., Javidipour, I., Meral, R., and Ceylan, Z. (2021). A novel coating material: ellagitannins-loaded maltodextrin and lecithin-based nanomaterials. FOOD Biosci. 42, 101158. doi:10.1016/j.fbio.2021.101158
Ouyang, R., Cao, P., Jia, P., Wang, H., Zong, T., Dai, C., et al. (2021). Bistratal Au@Bi2S3 nanobones for excellent NIR-triggered/multimodal imaging-guided synergistic therapy for liver cancer. Bioact. Mater. 6 (2), 386–403. doi:10.1016/j.bioactmat.2020.08.023
Oyagbemi, A. A., Omobowale, T. O., Ola-Davies, O. E., Asenuga, E. R., Ajibade, T. O., Adejumobi, O. A., et al. (2018). Quercetin attenuates hypertension induced by sodium fluoride via reduction in oxidative stress and modulation of HSP 70/ERK/PPARγ signaling pathways. Biofactors 44 (5), 465–479. doi:10.1002/biof.1445
Ozkan, C. K. (2023). Tanning with pomegranate peel tannin. J. Am. LEATHER Chem. Assoc. 118 (7), 301–306. doi:10.34314/jalca.v118i7.7860
Padma, V. V., Selvi, P. K., and Sravani, S. (2014). Protective effect of ellagic acid against TCDD-induced renal oxidative stress: modulation of CYP1A1 activity and antioxidant defense mechanisms. Mol. Biol. Rep. 41 (7), 4223–4232. doi:10.1007/s11033-014-3292-5
Parvez, M. A. K., Saha, K., Rahman, J., Munmun, R. A., Rahman, M. A., Dey, S. K., et al. (2019). Antibacterial activities of green tea crude extracts and synergistic effects of epigallocatechingallate (EGCG) with gentamicin against MDR pathogens. HELIYON 5 (7), e02126. doi:10.1016/j.heliyon.2019.e02126
Parvez, M. K., Al-Dosari, M. S., Basudan, O. A., and Herqash, R. N. (2022). The anti-hepatitis B virus activity of sea buckthorn is attributed to quercetin, kaempferol and isorhamnetin. Biomed. Rep. 17 (5), 89. doi:10.3892/br.2022.1573
Parvin, K., Nahar, K., Hasanuzzaman, M., Bhuyan, M. B., Mohsin, S. M., and Fujita, M. (2020). Exogenous vanillic acid enhances salt tolerance of tomato: insight into plant antioxidant defense and glyoxalase systems. Plant Physiol. Biochem. 150, 109–120. doi:10.1016/j.plaphy.2020.02.030
Pereira, S. C., Parente, J. M., Belo, V. A., Mendes, A. S., Gonzaga, N. A., do Vale, G. T., et al. (2018). Quercetin decreases the activity of matrix metalloproteinase-2 and ameliorates vascular remodeling in renovascular hypertension. Atherosclerosis 270, 146–153. doi:10.1016/j.atherosclerosis.2018.01.031
Pyrzynska, K., and Pękal, A. (2013). Application of free radical diphenylpicrylhydrazyl (DPPH) to estimate the antioxidant capacity of food samples. Anal. METHODS 5 (17), 4288–4295. doi:10.1039/c3ay40367j
Qiao, L. P., Liang, Y. P., Chen, J. Y., Huang, Y., Alsareii, S. A., Alamri, A. M., et al. (2023). Antibacterial conductive self-healing hydrogel wound dressing with dual dynamic bonds promotes infected wound healing. Bioact. Mater. 30, 129–141. doi:10.1016/j.bioactmat.2023.07.015
Rafey, A., Amin, A., Kamran, M., Haroon, U., Farooq, K., Foubert, K., et al. (2021). Analysis of plant origin antibiotics against oral bacterial infections using in vitro and in silico techniques and characterization of active constituents. ANTIBIOTICS-BASEL 10 (12), 1504. doi:10.3390/antibiotics10121504
Ragunath, R., Pratima, B. J., Premnath, B. J., and Nalini, N. (2022). Quercetin prevents bisphenol S induced behavioral changes and oxidative stress iZebrafish by modulating brain antioxidant defense mechanism. Biosci. Biotechnol. Res. Asia 19 (3), 589–600. doi:10.13005/bbra/3012
Rai, A., Gill, M., Kinra, M., Shetty, R., Krishnadas, N., Rao, C. M., et al. (2019). Catechin ameliorates depressive symptoms in Sprague Dawley rats subjected to chronic unpredictable mild stress by decreasing oxidative stress. Biomed. Rep. 11 (2), 79–84. doi:10.3892/br.2019.1226
Ranjha, M. M. A. N., Shafique, B., Wang, L., Irfan, S., Safdar, M. N., Murtaza, M. A., et al. (2023). A comprehensive review on phytochemistry, bioactivity and medicinal value of bioactive compounds of pomegranate (Punica granatum). Adv. TRADITIONAL Med. 23 (1), 37–57. doi:10.1007/s13596-021-00566-7
Rao, F., Tian, H., Li, W., Hung, H., and Sun, F. (2016). Potential role of punicalagin against oxidative stress induced testicular damage. Asian J. Androl. 18 (4), 627–632. doi:10.4103/1008-682X.168792
Raza, S., Ansari, A., Siddiqui, N. N., Ibrahim, F., Abro, M. I., and Aman, A. (2021). Biosynthesis of silver nanoparticles for the fabrication of non cytotoxic and antibacterial metallic polymer based nanocomposite system. Sci. Rep. 11 (1), 10500. doi:10.1038/s41598-021-90016-w
Ren, Q., Guo, F., Tao, S., Huang, R., Ma, L., and Fu, P. (2020). Flavonoid fisetin alleviates kidney inflammation and apoptosis via inhibiting Src-mediated NF-κB p65 and MAPK signaling pathways in septic AKI mice. Biomed. and Pharmacother. 122, 109772. doi:10.1016/j.biopha.2019.109772
Reyes, A. W. B., Arayan, L. T., Hop, H. T., Huy, T. X. N., Vu, S. H., Min, W., et al. (2018). Effects of gallic acid on signaling kinases in murine macrophages and immune modulation against Brucella abortus 544 infection in mice. Microb. Pathog. 119, 255–259. doi:10.1016/j.micpath.2018.04.032
Reyes, A. W. B., Hong, T. G., Hop, H. T., Arayan, L. T., Huy, T. X. N., Min, W., et al. (2017). The in vitro and in vivo protective effects of tannin derivatives against Salmonella enterica serovar Typhimurium infection. Microb. Pathog. 109, 86–93. doi:10.1016/j.micpath.2017.05.034
Ridlon, J. M., Harris, S. C., Bhowmik, S., Kang, D.-J., and Hylemon, P. B. (2016). Consequences of bile salt biotransformations by intestinal bacteria. Gut microbes 7 (1), 22–39. doi:10.1080/19490976.2015.1127483
Roacho-Perez, J. A., Garza-Trevino, E. N., Moncada-Saucedo, N. K., Carriquiry-Chequer, P. A., Valencia-Gomez, L. E., Matthews, E. R., et al. (2022). Artificial scaffolds in cardiac tissue engineering. LIFE-BASEL 12 (8), 1117. doi:10.3390/life12081117
Ryall, C., Chen, S., Duarah, S., and Wen, J. (2022). Chitosan-based microneedle arrays for dermal delivery of Centella asiatica. Int. J. Pharm. 627, 122221. doi:10.1016/j.ijpharm.2022.122221
Sadek, K. M., Mamdouh, W., Habib, S. I., El Deftar, M., and Habib, A. N. A. (2021). In vitro biological evaluation of a fabricated polycaprolactone/pomegranate electrospun scaffold for bone regeneration. ACS OMEGA 6 (50), 34447–34459. doi:10.1021/acsomega.1c04608
Sagar, N. A., Pareek, S., Sharma, S., Yahia, E. M., and Gloria Lobo, M. (2018). Fruit and vegetable waste: bioactive compounds, their extraction, and possible utilization. Compr. Rev. FOOD Sci. FOOD Saf. 17 (3), 512–531. doi:10.1111/1541-4337.12330
Sanchez-Terron, G., Martinez, R., Morcuende, D., Caballero, V., and Estevez, M. (2024). Pomegranate supplementation alleviates dyslipidemia and the onset of non-alcoholic fatty liver disease in Wistar rats by shifting microbiota and producing urolithin-like microbial metabolites. FOOD and Funct. 15 (14), 7348–7363. doi:10.1039/d4fo00688g
Sarjit, A., Wang, Y., and Dykes, G. A. (2015). Antimicrobial activity of gallic acid against thermophilic Campylobacter is strain specific and associated with a loss of calcium ions. Food Microbiol. 46, 227–233. doi:10.1016/j.fm.2014.08.002
Satomi, H., Umemura, K., Ueno, A., Hatano, T., Okuda, T., and Noro, T. (1993). Carbonic anhydrase inhibitors from the pericarps of Punica granatum L. Biol. Pharm. Bull. 16 (8), 787–790. doi:10.1248/bpb.16.787
Savekar, P. L., Nadaf, S. J., Killedar, S. G., Kumbar, V. M., Hoskeri, J. H., Bhagwat, D. A., et al. (2024). Citric acid cross-linked pomegranate peel extract-loaded pH-responsive Ş-cyclodextrin/carboxymethyl tapioca starch hydrogel film for diabetic wound healing. Int. J. Biol. Macromol. 274, 133366. doi:10.1016/j.ijbiomac.2024.133366
Seo, Y., Mun, C. H., Park, S.-H., Jeon, D., Kim, S. J., Yoon, T., et al. (2020). Punicalagin ameliorates lupus nephritis via inhibition of PAR2. Int. J. Mol. Sci. 21 (14), 4975. doi:10.3390/ijms21144975
Shabir, I., Dar, A. H., Dash, K. K., Manzoor, S., Srivastava, S., Pandey, V. K., et al. (2024). Bioactive potential of punicalagin: a comprehensive review. Appl. Food Res. 4, 100572. doi:10.1016/j.afres.2024.100572
Shahidi, F., and Ambigaipalan, P. (2015). Phenolics and polyphenolics in foods, beverages and spices: antioxidant activity and health effects - a review. J. Funct. FOODS 18, 820–897. doi:10.1016/j.jff.2015.06.018
Shang, Y. S., Du, J. S., Wang, B. R., Lu, P. F., Zhao, Y. A., and Yuan, J. (2022). Preparation of MSNs@Keratin as pH/GSH dual responsive drug delivery system. J. BIOMATERIALS SCIENCE-POLYMER Ed. 33 (11), 1369–1382. doi:10.1080/09205063.2022.2056940
Shao, Z. J., Yin, T. Y., Jiang, J. B., He, Y., Xiang, T., and Zhou, S. B. (2023). Wound microenvironment self-adaptive hydrogel with efficient angiogenesis for promoting diabetic wound healing. Bioact. Mater. 20, 561–573. doi:10.1016/j.bioactmat.2022.06.018
Sharma, K., Kesharwani, P., Prajapati, S. K., Jain, A., Jain, D., Mody, N., et al. (2022). An insight into anticancer bioactives from punica granatum pomegranate. ANTI-CANCER AGENTS Med. Chem. 22 (4), 694–702. doi:10.2174/1871520621666210726143553
Shi, D., Beasock, D., Fessler, A., Szebeni, J., Ljubimova, J. Y., Afonin, K. A., et al. (2022). To PEGylate or not to PEGylate: immunological properties of nanomedicine's most popular component, polyethylene glycol and its alternatives. Adv. DRUG Deliv. Rev. 180, 114079. doi:10.1016/j.addr.2021.114079
Shi, L. W., Zhang, J. Q., Zhao, M., Tang, S. K., Cheng, X., Zhang, W. Y., et al. (2021). Effects of polyethylene glycol on the surface of nanoparticles for targeted drug delivery. NANOSCALE 13 (24), 10748–10764. doi:10.1039/d1nr02065j
Shikha, D., Singh, A., Rangra, N. K., Monga, V., and Bhatia, R. (2024). Insights to therapeutic potentials, pharmaceutical formulations, chemistry and analytical methods of catechin. Phytochem. Rev. 23, 1557–1598. doi:10.1007/s11101-024-09929-9
Shirode, A. B., Bharali, D. J., Nallanthighal, S., Coon, J. K., Mousa, S. A., and Reliene, R. (2015). Nanoencapsulation of pomegranate bioactive compounds for breast cancer chemoprevention. Int. J. nanomedicine 10, 475–484. doi:10.2147/IJN.S65145
Shukla, A. S., Jha, A. K., Kumari, R., Rawat, K., Syeda, S., and Shrivastava, A. (2018). “Role of catechins in chemosensitization,” in Role of nutraceuticals in cancer chemosensitization (Elsevier), 169–198.
Siddiqui, S., and Arshad, M. (2014). Osteogenic potential of punica granatum through matrix mineralization, cell cycle progression and runx2 gene expression in primary rat osteoblasts. Daru 22, 72–78. doi:10.1186/s40199-014-0072-7
Siddiqui, S. A., Singh, S., and Nayik, G. A. (2024). Bioactive compounds from pomegranate peels - biological properties, structure-function relationships, health benefits and food applications - a comprehensive review. J. Funct. FOODS 116, 106132. doi:10.1016/j.jff.2024.106132
Silva, T. M., Bolzan, T. C. A., Zanini, M. S., Alencar, T., Rodrigues, W. D., Bastos, K. A., et al. (2020). Development and evaluation of a novel oral mucoadhesive ointment containing pomegranate peel extract as an adjuvant for oral hygiene of dogs. J. VETERINARY Dent. 37 (3), 133–140. doi:10.1177/0898756420973470
Singh, A., D'Amico, D., Andreux, P. A., Dunngalvin, G., Kern, T., Blanco-Bose, W., et al. (2022). Direct supplementation with Urolithin A overcomes limitations of dietary exposure and gut microbiome variability in healthy adults to achieve consistent levels across the population. Eur. J. Clin. Nutr. 76 (2), 297–308. doi:10.1038/s41430-021-00950-1
Singh, J., Kaur, H. P., Verma, A., Chahal, A. S., Jajoria, K., Rasane, P., et al. (2023). Pomegranate peel phytochemistry, pharmacological properties, methods of extraction, and its application: a comprehensive review. ACS OMEGA 8 (39), 35452–35469. doi:10.1021/acsomega.3c02586
Singh, J. P., Kaur, A., Shevkani, K., and Singh, N. (2016). Composition, bioactive compounds and antioxidant activity of common Indian fruits and vegetables. J. FOOD Sci. TECHNOLOGY-MYSORE 53 (11), 4056–4066. doi:10.1007/s13197-016-2412-8
Sinha, S. R., Haileselassie, Y., Nguyen, L. P., Tropini, C., Wang, M., Becker, L. S., et al. (2020). Dysbiosis-induced secondary bile acid deficiency promotes intestinal inflammation. Cell host microbe 27 (4), 659–670.e5. doi:10.1016/j.chom.2020.01.021
Soltanzadeh, M., Peighambardoust, S. H., Ghanbarzadeh, B., Mohammadi, M., and Lorenzo, J. M. (2021). Chitosan nanoparticles as a promising nanomaterial for encapsulation of pomegranate (Punica granatum L.) peel extract as a natural source of antioxidants. NANOMATERIALS 11 (6), 1439. doi:10.3390/nano11061439
Song, J., He, Y., Luo, C., Feng, B., Ran, F., Xu, H., et al. (2020). New progress in the pharmacology of protocatechuic acid: a compound ingested in daily foods and herbs frequently and heavily. Pharmacol. Res. 161, 105109. doi:10.1016/j.phrs.2020.105109
Song, N., Zhang, J., Zhai, J., Hong, J., Yuan, C., and Liang, M. (2021). Ferritin: a multifunctional nanoplatform for biological detection, imaging diagnosis, and drug delivery. ACCOUNTS Chem. Res. 54 (17), 3313–3325. doi:10.1021/acs.accounts.1c00267
Spilmont, M., Léotoing, L., Davicco, M.-J., Lebecque, P., Miot-Noirault, E., Pilet, P., et al. (2015). Pomegranate peel extract prevents bone loss in a preclinical model of osteoporosis and stimulates osteoblastic differentiation in vitro. Nutrients 7 (11), 9265–9284. doi:10.3390/nu7115465
Sun, G., Zhang, S., Xie, Y., Zhang, Z., and Zhao, W. (2016). Gallic acid as a selective anticancer agent that induces apoptosis in SMMC-7721 human hepatocellular carcinoma cells. Oncol. Lett. 11 (1), 150–158. doi:10.3892/ol.2015.3845
Sun, H., Yin, M., Hao, D., and Shen, Y. (2020). Anti-cancer activity of catechin against A549 lung carcinoma cells by induction of cyclin kinase inhibitor P21 and suppression of cyclin E1 and P–AKT. Appl. Sci. 10 (6), 2065. doi:10.3390/app10062065
Sun, W., and Shahrajabian, M. H. (2023). Therapeutic potential of phenolic compounds in medicinal plants-natural health products for human health. MOLECULES 28 (4), 1845. doi:10.3390/molecules28041845
Sun, Y.-q., Xin, T., Men, X.-m., Xu, Z.-w., and Tian, W. (2017). In vitro and in vivo antioxidant activities of three major polyphenolic compounds in pomegranate peel: ellagic acid, punicalin, and punicalagin. J. Integr. Agric. 16 (8), 1808–1818. doi:10.1016/s2095-3119(16)61560-5
Taherian, A., Esfandiari, N., and Rouhani, S. (2021). Breast cancer drug delivery by novel drug-loaded chitosan-coated magnetic nanoparticles. CANCER Nanotechnol. 12 (1), 15. doi:10.1186/s12645-021-00086-8
Takakura, Y., and Takahashi, Y. (2022). Strategies for persistent retention of macromolecules and nanoparticles in the blood circulation. J. Control. RELEASE 350, 486–493. doi:10.1016/j.jconrel.2022.05.063
Tang, Y., Li, Y., Yu, H., Gao, C., Liu, L., Xing, M., et al. (2014). Quercetin attenuates chronic ethanol hepatotoxicity: implication of “free” iron uptake and release. Food Chem. Toxicol. 67, 131–138. doi:10.1016/j.fct.2014.02.022
Tenchov, R., Bird, R., Curtze, A. E., and Zhou, Q. (2021). Lipid nanoparticles-from liposomes to mRNA vaccine delivery, a landscape of research diversity and advancement. ACS Nano 15 (11), 16982–17015. doi:10.1021/acsnano.1c04996
Tian, L., Wu, M., Li, H., and Gong, G. (2022). Transcriptome analysis of Micrococcus luteus in response to treatment with protocatechuic acid. J. Appl. Microbiol. 133 (5), 3139–3149. doi:10.1111/jam.15743
Tian, Q., Wei, S., Su, H., Zheng, S., Xu, S., Liu, M., et al. (2022). Bactericidal activity of gallic acid against multi-drug resistance Escherichia coli. Microb. Pathog. 173, 105824. doi:10.1016/j.micpath.2022.105824
Tiyaboonchai, W., Rodleang, I., and Ounaroon, A. (2015). Mucoadhesive polyethylenimine-dextran sulfate nanoparticles containing Punica granatum peel extract as a novel sustained-release antimicrobial. Pharm. Dev. Technol. 20 (4), 426–432. doi:10.3109/10837450.2013.879884
Toda, K., Ueyama, M., Tanaka, S., Tsukayama, I., Mega, T., Konoike, Y., et al. (2020). Ellagitannins from Punica granatum leaves suppress microsomal prostaglandin E synthase-1 expression and induce lung cancer cells to undergo apoptosis. Biosci. Biotechnol. Biochem. 84 (4), 757–763. doi:10.1080/09168451.2019.1706442
Tokton, N., Ounaroon, A., Panichayupakaranant, P., and Tiyaboonchai, W. (2014). Development of ellagic acid rich pomegranate peel extract loaded nanostructured lipid carriers (NLCS). Int. J. Pharm. Sci. 6 (4), 259–265.
Torzewska, A., and Rozalski, A. J. M. r. (2014). Inhibition of crystallization caused by Proteus mirabilis during the development of infectious urolithiasis by various phenolic substances. Microbiol. Res. 169 (7-8), 579–584. doi:10.1016/j.micres.2013.09.020
Tu, S., Xiao, F., Min, X., Chen, H., Fan, X., and Cao, K. (2018). Catechin attenuates coronary heart disease in a rat model by inhibiting inflammation. Cardiovasc. Toxicol. 18 (5), 393–399. doi:10.1007/s12012-018-9449-z
Ul-Islam, M., Alhajaim, W., Fatima, A., Yasir, S., Kamal, T., Abbas, Y., et al. (2023). Development of low-cost bacterial cellulose-pomegranate peel extract-based antibacterial composite for potential biomedical applications. Int. J. Biol. Macromol. 231, 123269. doi:10.1016/j.ijbiomac.2023.123269
Valero-Mendoza, A., Meléndez-Rentería, N., Chávez-González, M., Flores-Gallegos, A., Wong-Paz, J., Govea-Salas, M., et al. (2023). The whole pomegranate (Punica granatum. L), biological properties and important findings: a review. Food Chem. Adv. 2, 100153. doi:10.1016/j.focha.2022.100153
Wang, D., Wei, X., Yan, X., Jin, T., and Ling, W. (2010). Protocatechuic acid, a metabolite of anthocyanins, inhibits monocyte adhesion and reduces atherosclerosis in apolipoprotein E-deficient mice. J. Agric. FOOD Chem. 58 (24), 12722–12728. doi:10.1021/jf103427j
Wang, H., Yan, Y., Pathak, J. L., Hong, W., Zeng, J., Qian, D., et al. (2023). Quercetin prevents osteoarthritis progression possibly via regulation of local and systemic inflammatory cascades. J. Cell Mol. Med. 27 (4), 515–528. doi:10.1111/jcmm.17672
Wang, T., Liu, B., Zhang, C., Fang, T., Ding, Y., Song, G., et al. (2022). Kaempferol-Driven inhibition of listeriolysin O pore formation and inflammation suppresses Listeria monocytogenes infection. Microbiol. Spectr. 10 (4), 01810222–e201822. doi:10.1128/spectrum.01810-22
Wani, M. Y., Ganie, N. A., Wani, D. M., Wani, A. W., Dar, S. Q., Khan, A. H., et al. (2023). The phenolic components extracted from mulberry fruits as bioactive compounds against cancer: a review. Phytotherapy Res. 37 (3), 1136–1152. doi:10.1002/ptr.7713
Weems, A. C., Arno, M. C., Yu, W., Huckstepp, R. T. R., and Dove, A. P. (2021). 4D polycarbonates via stereolithography as scaffolds for soft tissue repair. Nat. Commun. 12 (1), 3771. doi:10.1038/s41467-021-23956-6
Wianowska, D., and Olszowy-Tomczyk, M. (2023). A concise profile of gallic acid—from its natural sources through biological properties and chemical methods of determination. MOLECULES 28 (3), 1186. doi:10.3390/molecules28031186
Wu, M., Tian, L., Fu, J., Liao, S., Li, H., Gai, Z., et al. (2022). Antibacterial mechanism of Protocatechuic acid against Yersinia enterocolitica and its application in pork. Food control. 133, 108573. doi:10.1016/j.foodcont.2021.108573
Xiang, Q. W., Li, M. F., Wen, J. Y., Ren, F. J., Yang, Z., Jiang, X. Y., et al. (2022). The bioactivity and applications of pomegranate peel extract: a review. J. FOOD Biochem. 46 (7), e14105. doi:10.1111/jfbc.14105
Xiao, G., Zhang, M., Peng, X., and Jiang, G. (2021). Protocatechuic acid attenuates cerebral aneurysm formation and progression by inhibiting TNF-alpha/Nrf-2/NF-kB-mediated inflammatory mechanisms in experimental rats. Open life Sci. 16 (1), 128–141. doi:10.1515/biol-2021-0012
Xing, S., Zhang, X., Luo, L., Cao, W., Li, L., He, Y., et al. (2018). Doxorubicin/gold nanoparticles coated with liposomes for chemo-photothermal synergetic antitumor therapy. NANOTECHNOLOGY 29 (40), 405101. doi:10.1088/1361-6528/aad358
Xu, J., Cao, K., Liu, X., Zhao, L., Feng, Z., and Liu, J. (2022). Punicalagin regulates signaling pathways in inflammation-associated chronic diseases. ANTIOXIDANTS 11 (1), 29. doi:10.3390/antiox11010029
Yamada, H., Wakamori, S., Hirokane, T., Ikeuchi, K., and Matsumoto, S. (2018). Structural revisions in natural ellagitannins. MOLECULES 23 (8), 1901. doi:10.3390/molecules23081901
Yu, W., Liu, M., Song, P., Shen, C., and Yang, Y. (2023). The biological activity and detection methods of ellagic acid. Shengwu Jiagong Guocheng 21 (1), 83–90. doi:10.3969/j.issn.1672-3678.2023.01.010
Yu, Y.-M., Chang, W.-C., Wu, C.-H., and Chiang, S.-Y. (2005). Reduction of oxidative stress and apoptosis in hyperlipidemic rabbits by ellagic acid. J. Nutr. Biochem. 16 (11), 675–681. doi:10.1016/j.jnutbio.2005.03.013
Yu, Z., Huang, W., Wang, F., Nie, X., Chen, G., Zhang, L., et al. (2024). An adhesion-switchable hydrogel dressing for painless dressing removal without secondary damage. J. Mater. Chem. B 12 (23), 5628–5644. doi:10.1039/d4tb00621f
Yuan, S., Wang, B., Wang, M., Sun, M., Wang, X., Li, X., et al. (2024). Antifungal mechanism of protocatechuic acid methyl ester against Botrytis cinerea in postharvest strawberry fruit. Postharvest Biol. Technol. Health Care 211, 112787. doi:10.1016/j.postharvbio.2024.112787
Zhan, R., Xu, X., Cui, Y., Ma, J., Liu, H., Wang, Y., et al. (2024). Dual-functional nano-carrier system based on NIR-laser-triggered dynamic Au-Se interaction for chemo-photothermal synergistic tumor therapy. COLLOID INTERFACE Sci. Commun. 59, 100774. doi:10.1016/j.colcom.2024.100774
Zhang, J., Tong, D. M., Song, H. H., Ruan, R. J., Sun, Y. F., Lin, Y. D., et al. (2022). Osteoimmunity-regulating biomimetically hierarchical scaffold for augmented bone regeneration. Adv. Mater. 34 (36), e2202044. doi:10.1002/adma.202202044
Zhang, W., Zheng, Y., Yan, F., Dong, M., and Ren, Y. (2023). Research progress of quercetin in cardiovascular disease. Front. Cardiovasc. Med. 10, 1203713. doi:10.3389/fcvm.2023.1203713
Zhang, X., Fan, J., Xiao, D., Edirisinghe, I., Burton-Freeman, B. M., and Sandhu, A. K. (2021). Pharmacokinetic evaluation of red raspberry (Poly)phenols from two doses and association with metabolic indices in adults with prediabetes and insulin resistance. J. Agric. FOOD Chem. 69 (32), 9238–9248. doi:10.1021/acs.jafc.1c02404
Zhang, X., Zhao, A., Sandhu, A. K., Edirisinghe, I., and Burton-Freeman, B. M. (2020). Functional deficits in gut microbiome of young and middle-aged adults with prediabetes apparent in metabolizing bioactive (Poly)phenols. NUTRIENTS 12 (11), 3595. doi:10.3390/nu12113595
Zhao, L., Zhou, N., Zhang, H., Pan, F., Ai, X., Wang, Y., et al. (2021). Cyanidin-3-O-glucoside and its metabolite protocatechuic acid ameliorate 2-amino-1-methyl-6-phenylimidazo[4,5-b]pyridine (PhIP) induced cytotoxicity in HepG2 cells by regulating apoptotic and Nrf2/p62 pathways. FOOD Chem. Toxicol. 157, 112582. doi:10.1016/j.fct.2021.112582
Zhou, C., Wang, Y., Li, C., Xie, Z., and Dai, L. (2023). Amelioration of colitis by a gut bacterial Consortium producing anti-inflammatory secondary bile acids. Microbiol. Spectr. 11 (2), e03330-22. doi:10.1128/spectrum.03330-22
Zhu, L., Gu, P., and Shen, H. (2019). Gallic acid improved inflammation via NF-κB pathway in TNBS-induced ulcerative colitis. Int. Immunopharmacol. 67, 129–137. doi:10.1016/j.intimp.2018.11.049
Zhuang, Z., Ye, G., and Huang, B. (2017). Kaempferol alleviates the interleukin-1β-induced inflammation in rat osteoarthritis chondrocytes via suppression of NF-κB. Med. Sci. Monit. Int. Med. J. Exp. Clin. Res. 23, 3925–3931. doi:10.12659/msm.902491
Keywords: pomegranate peel extract, bioactivity, biomedical applications, nanodrug carriers, hydrogels, tissue engineering scaffolds
Citation: Du J, Wang H, Zhong L, Wei S, Min X, Deng H, Zhang X, Zhong M and Huang Y (2025) Bioactivity and biomedical applications of pomegranate peel extract: a comprehensive review. Front. Pharmacol. 16:1569141. doi: 10.3389/fphar.2025.1569141
Received: 31 January 2025; Accepted: 07 March 2025;
Published: 26 March 2025.
Edited by:
Ruyu Yao, Chinese Academy of Sciences (CAS), ChinaReviewed by:
Adnan Amin, Gomal University, PakistanCopyright © 2025 Du, Wang, Zhong, Wei, Min, Deng, Zhang, Zhong and Huang. This is an open-access article distributed under the terms of the Creative Commons Attribution License (CC BY). The use, distribution or reproduction in other forums is permitted, provided the original author(s) and the copyright owner(s) are credited and that the original publication in this journal is cited, in accordance with accepted academic practice. No use, distribution or reproduction is permitted which does not comply with these terms.
*Correspondence: Ming Zhong, emhvbmdtMDEyNUAxNjMuY29tJiN4MDIwMGE7; Yi Huang, aHVhbmd5MTY2OUAxNjMuY29t
†These authors have contributed equally to this work
Disclaimer: All claims expressed in this article are solely those of the authors and do not necessarily represent those of their affiliated organizations, or those of the publisher, the editors and the reviewers. Any product that may be evaluated in this article or claim that may be made by its manufacturer is not guaranteed or endorsed by the publisher.
Research integrity at Frontiers
Learn more about the work of our research integrity team to safeguard the quality of each article we publish.