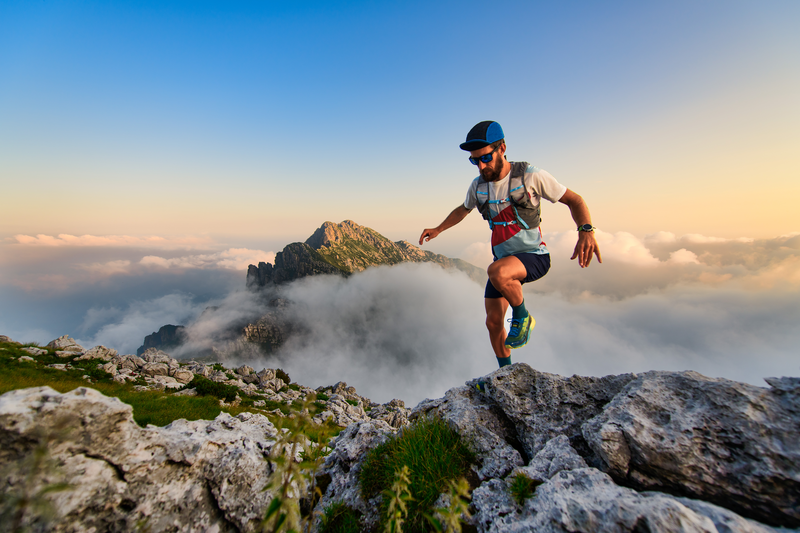
95% of researchers rate our articles as excellent or good
Learn more about the work of our research integrity team to safeguard the quality of each article we publish.
Find out more
MINI REVIEW article
Front. Pharmacol. , 19 March 2025
Sec. Inflammation Pharmacology
Volume 16 - 2025 | https://doi.org/10.3389/fphar.2025.1568828
This article is part of the Research Topic Therapeutic Effects of Endogenous Hormones in Pathologies Linked to Metabolic and/or Inflammatory Disorders View all 5 articles
The present review explores the role of endogenous hormones, such as cortisol, melatonin, thyroid hormones, sex hormones, and insulin, in the modulation of the immune response to a human metapneumovirus (hMPV) infection. hMPV is a respiratory pathogen responsible for severe infections, particularly in vulnerable populations like children and the elderly. The virus triggers inflammatory responses through various molecular processes, including cytokine production and immune signaling pathways. Notably, these processes can be influenced by endocrine factors, such as hormones. Cortisol, through hypothalamic-pituitary-adrenal (HPA) axis activation, modulates inflammation but may contribute to immunosuppression. Melatonin inhibits the NLRP3 inflammasome, reducing lung inflammation. Thyroid hormones regulate immune responses via nuclear factor kappa B (NF-κB) and JAK/STAT pathways, while hypothyroidism may alter infection severity. Sex hormones, particularly estrogens, enhance antiviral immunity, whereas androgens may have variable effects on immune modulation. Insulin influences inflammation through NF-κB suppression, with insulin resistance potentially worsening viral pathogenesis. Therapeutic implications suggest that modulating these hormonal pathways could aid in hMPV management. Strategies such as hormone therapy, glucocorticoid regulation, and nanoparticle-based drug delivery are potential routes of intervention. The aim of the present review is to understand the complex interplay between endogenous hormones and the immune system during an hMPV infection by describing the complex molecular mechanisms associated with these processes.
The human metapneumovirus (hMPV) is a single-stranded, negative-sense RNA virus classified within the genus Metapneumovirus (Oketch et al., 2019; Zhang et al., 2024; Piñana et al., 2024). hMPV is considered the primary cause of acute lower respiratory infection in both adults and children worldwide (Zhang et al., 2024). Infection with hMPV induces airway inflammation, coughing, shortness of breath, sore throat, and necrosis of the airway epithelium (Cox et al., 2015). In severe cases, the infection can progress to bronchiolitis or pneumonia (Van Den Bergh et al., 2024).
An increased prevalence and risk of hMPV infection have been reported in children under 2 years of age, the elderly, and individuals with cancer, AIDS, or a history of organ transplantation (Oketch et al., 2019; Zhang et al., 2024). Currently, no approved treatments exist for an hMPV infection; thus, management mainly involves supportive care. However, various potential treatments, including nucleoside analogs and immunoglobulins, are under investigation (Piñana et al., 2024).
hMPV is classified into two major groups (A and B) based on antigenic variation and nucleotide differences in the genes encoding the attachment (G), nucleoprotein (N), and fusion (F) glycoproteins. Additionally, each group is further divided into two subgroups (A1, A2, B1, and B2) according to their Open Reading Frame (ORF) sequences (Oketch et al., 2019).
hMPV is an enveloped virus; thus, its entry into the host cells is mediated by the fusion of the viral and cellular membranes (Cox et al., 2015). Notably, human cells are generally coated with glycoproteins, which are essential for various cellular processes and are often exploited by pathogens for cell entry. In the case of hMPV, the virus utilizes heparan sulfate proteoglycans (HSPGs), expressed in almost all human cell types, to attach to host cells and facilitate viral entry. Furthermore, the hMPV F protein has an arginine-glycine-aspartic acid (RGD) motif that binds to the cell surface integrins, thereby, promoting virus-cell membrane interaction and enhancing viral entry (Van Den Bergh et al., 2024).
The endocrine system influences immunity by releasing molecules such as hormones to modulate the immune response, either by enhancing or weakening it (Sciarra et al., 2023). Conversely, various viruses have developed specific mechanisms to disrupt the proper function of the endocrine system, potentially leading to endocrinopathies (Somasundaram and Gunatilake, 2021). Viruses can damage endocrine cells through direct viral infection or during their replication process using the host machinery, affecting endocrine organs by promoting inflammation and increasing the risks of an autoimmune response through antibody cross-reactivity (Somasundaram and Gunatilake, 2021).
The immune response to an hMPV infection is characterized by the activation of Th17-like cells, which secrete tumor necrosis factor-alpha TNF-α) and interleukin (IL)-6 in the lungs (Soto et al., 2018). Additionally, Th2-like cells are also activated during this process, leading to the secretion of IL-5 and IL-8, which are associated with the JAK/STAT pathway as well as the MAPK and nuclear factor kappa B (NF-κB) pathways, respectively, promoting inflammation (Takatsu et al., 2009; Chan et al., 2017). Moreover, studies in mice have shown that the Toll-like receptor-4 (TLR)-4, which is linked to NF-κB pathways and pro-inflammatory cytokines (Guevara-Ramírez et al., 2023; Ruiz-Pozo et al., 2023; Ruiz-Pozo et al., 2024), plays a role in the immune response to hMPV. A lack of TLR4 was correlated to a decreased immune response, including lower levels of chemokines and cytokines in mice (Velayutham et al., 2013).
On the other hand, hMPV can disrupt inflammatory pathways, including the inhibition of Interferon (IFN) signaling and production via its viral G and SH proteins (Soto et al., 2018; Bao et al., 2008). Similarly, the M2-2 protein of hMPV can inhibit mitochondrial antiviral signaling (MAVS). Since MAVS is required for NF-kB activation, this pathway remains inactive, leading to increased viral replication and cell death (Seth et al., 2005). Furthermore, certain strains of hMPV have evolved mechanisms to evade the type I IFN response because their phosphoprotein P can disrupt Retinoic acid-inducible gene (RIG)-1 activation, thereby inhibiting the inflammatory response (Velayutham et al., 2013).
The aim of the present review is to understand the intricate interaction between endogenous hormones—including cortisol, melatonin, thyroid hormones, sex hormones, and insulin—and the immune response to an hMPV infection by reviewing the complex molecular mechanisms associated with this interplay.
The hypothalamic-pituitary-adrenal (HPA) axis is a neuroendocrine system that connects the central nervous system with peripheral tissues. It regulates homeostasis, under conditions of physiological and pathological stress, such as viral infections (Sunstrum and Inoue, 2019; Weng et al., 2023). In hMPV infection, activation of the HPA axis and subsequent cortisol release attempt to prevent tissue damage caused by uncontrolled inflammation. Cortisol, a glucocorticoid with immunomodulatory properties, plays a central role in regulating the inflammatory response and modulating pro-inflammatory cytokines (Herman et al., 2016).
During hMPV infection, the release of pro-inflammatory cytokines activates the HPA axis as part of the immune response. This activation begins in the central nervous system, where the hypothalamus, in response to inflammatory signals, stimulates the release of corticotropin-releasing hormone (CRH). In the early stages of infection, innate cytokines such as IL-1, IL-6, and TNF- α induce CRH secretion by neurons in the paraventricular nucleus (PVN) of the hypothalamus. These neurons project their endings to the median eminence (ME), where CRH is released into the portal-pituitary circulation, promoting activation of the HPA axis and subsequent endocrine response (Somasundaram and Gunatilake, 2021; Silverman et al., 2005).
CRH binds to CRH-R1 receptors on anterior pituitary corticotropic cells, triggering the synthesis and release of adrenocorticotropic hormone (ACTH). ACTH travels through the circulation to the adrenal glands, where it acts on MC2-R type 2 melanocortin receptors in the adrenal cortex, inducing the production of glucocorticoids such as cortisol (Cox et al., 2015). Cortisol regulates excessive inflammation through its interaction with glucocorticoid receptors (GR) in the cytosol of target cells. Binding cortisol to GR causes translocation of the receptor-ligand complex to the nucleus, where it regulates the transcription of genes involved in inflammation and antiviral response, modulating transcription factors such as NF-κB and AP-1 (Borghetti et al., 2009; Somasundaram and Gunatilake, 2021).
In advanced stages of hMPV infection, adaptive cytokines such as TNF-α, INF-γ, and IL-2 maintain active glucocorticoid production through the HPA axis to control systemic inflammation (Silverman et al., 2005; Borghetti et al., 2009; Gjerstad et al., 2018). However, negative feedback from glucocorticoids regulates this response by inhibiting CRH and ACTH release, desensitizing CRH-R1 receptors, and limiting axis activity. The hippocampus, with a high density of glucocorticoid receptors, also contributes to this regulation of HPA, protecting the body from excessive glucocorticoid exposure and reducing the risk of catabolic and immunosuppressive effects (Gjerstad et al., 2018).
Although respiratory symptoms are the main manifestations of hMPV, studies have identified its presence in cerebrospinal fluid (CSF), indicating that the virus can spread from the lungs to the central nervous system (CNS), causing complications such as encephalitis (Jeannet et al., 2017; Schildgen et al., 2005; Kalergis et al., 2023). This behavior could be linked to generalized inflammatory activation and HPA axis response, highlighting the need to understand how interactions between the immune system, glucocorticoids, and viral shedding contribute to the neurological manifestations observed in severe hMPV infections (Bohmwald et al., 2018).
The pineal gland’s primary hormone, melatonin, is essential for both human and animal pharmacological and pathophysiological states (Hosseinzadeh et al., 2016; Daryani et al., 2018). This hormone has a variety of functions, including immunoregulatory, anti-inflammatory, anti-excitatory, sleep-initiating, and antioxidant (Bahrampour Juybari et al., 2020).
The NLRP3 inflammasome is an oligomeric complex essential for host defense against viruses, as it increases the release of IL-1β and IL-18 and induces pyroptosis. This inflammasome recognizes pathogen-associated molecular patterns (PAMPs) and damage-associated molecular patterns (DAMPs) generated during viral replication, leading to antiviral immune responses and viral elimination (Lupfer et al., 2020).
Interestingly, Le et al. (2019) found that the NLRP3 inflammasome has a detrimental effect during hMPV infection. Its inhibition protected mice from mortality and reduced weight loss and inflammation without affecting viral replication. This negative effect was attributed to IL-1β production, as IL-1β-deficient mice exhibited less mortality, weight loss, and inflammation compared to wild-type mice. The research group also observed that activation of the NLRP3 inflammasome by the hMPV SH protein promotes IL-1β maturation, exacerbating hMPV-induced inflammation. Therefore, blocking IL-1β production with NLRP3 inflammasome inhibitors could be a novel therapeutic and preventive strategy for hMPV infection (Le et al., 2019).
In this context, Zhang et al. (2016) demonstrated that melatonin is a potent NLRP3 inflammasome inhibitor in an LPS-induced acute lung injury (ALI) mouse model. This beneficial effect of melatonin ameliorates lung damage and reduces neutrophil and macrophage influx into the lungs (Zhang et al., 2016).
Similarly, Wu et al. (2020) suggested that melatonin could be a promising therapeutic drug for inflammatory airway diseases. They demonstrated that an endogenous TLR2-melatonin feedback loop regulates NLRP3 inflammasome activation in allergic airway inflammation. Suppression of melatonin synthesis by TLR2 activation results in the loss of its inhibitory effect on TLR2 signaling (Wu et al., 2020).
Triiodothyronine (T3) and thyroxine (T4) are the main thyroid hormones that regulate metabolism, development, cardiac frequency, and immune responses (Brent, 2012). Their production is regulated by the hypothalamic-pituitary-thyroid axis, which operates as a feedback loop to maintain hormonal balance. The hypothalamus secretes thyrotropin-releasing hormone (TRH), promoting the anterior pituitary to release thyrotropin-stimulating hormone (TSH). TSH acts on the thyroid gland, promoting the production of T4, which is then converted into the active form, T3, by enzymes called deiodinases (Astapova et al., 2011; Feldt-Rasmussen et al., 2021). Thyroid hormones are transported across the cell membrane by proteins such as monocarboxylate transporter 8 (MCT8) to enter the cells (Mebis and van den Berghe, 2009). Once inside, thyroid hormones can act by binding to different molecules located on the plasma membrane, mediated by binding to thyroid nuclear receptors (Lasa et al., 2022).
Thyroid hormones play an essential role in interacting with molecular pathways like the immune response to respiratory viruses (Lasa et al., 2022). They modulate the activity of various immune cells, such as neutrophils, macrophages, natural killer cells, dendritic cells, and B and T lymphocytes, while also influencing key inflammatory pathways (Rubingh et al., 2020). For example, thyroid hormones influence the transcription of genes involved in inflammatory pathways, such as the NF-kB pathway, p38MAPK signaling pathway, and JAK/STAT signal cascade (Lasa et al., 2022; Wenzek et al., 2022). During acute infection, pro-inflammatory cytokines can suppress the production and activity of T4 and T3, resulting in non-thyroidal illness syndrome (NTIS) (Astapova et al., 2011; Aranda, 2025). Additionally, studies demonstrated that the function of macrophages and cytokine production could be affected by thyroid hormones (Wenzek et al., 2022).
In the case of hMPV infections, thyroid hormones can influence immune responses and the progression of the disease. Research has investigated the immune response to hMPV under hypothyroxinemia (Hpx) in gestated mice and found that Hpx females had a more effective immune response in reducing viral loads compared to Hpx males. These findings suggest that disruptions in thyroid hormone levels during hMPV infections may compromise protective immune mechanisms, potentially exacerbating symptoms and delaying recovery (Funes et al., 2022).
Endogenous sex hormones, including estrogens, progesterone, and androgens, are synthesized mainly in the gonads and the adrenal cortex (Delchev and Georgieva, 2018). The regulation of these hormones is provided by the hypothalamic-pituitary-gonadal (HPG) axis that promotes the activation of Gonadotropin-releasing hormone (GnRH) to direct the pituitary gland to release Luteinizing hormone (LH) and follicle-stimulating hormone (FSH) (Hu et al., 2010). LH and FSH hormones act on the gonads and synthesize sex hormones such as estrogens (e.g., estradiol), progesterone, and androgens (Lomauro and Aliverti, 2021; Acevedo-Rodriguez et al., 2018).
In the process of respiratory virus infection in the host, sex hormones influence the immune response through different molecular pathways. Estrogens bind to estrogen receptors (ER), ERα, and ERβ on immune cells such as B and T lymphocytes, macrophages, and dendritic cells. In addition, ERs act on Calcium regulation by inducing the activation of some metabolic pathways such as ERK/MAPK and PI3K/AKT through proteins such as calmodulin and Calcium-dependent kinases and JAK-STAT activated by cytokines; metabolic processes that promote type I and III IFN responses involved in antiviral defense (Ghosh and Klein, 2017; Hu et al., 2023).
Androgens, acting through the androgen receptor (AR), form an androgen-AR complex to modulate the transcription of target genes and activate the calcium cascade to promote the ERK, AKT, and MAPK signaling pathways involved in virus defense. Thus, exposure of monocytes and macrophages to androgens triggers the decrease of pro-inflammatory responses by regulating cytokines and IFNs. For example, research mentions that alveolar macrophages enhance IL-4-induced M2 polarization of macrophages. However, in some cases, inflammatory processes are enhanced by androgens, which may be due to the different types of macrophages in the tissues and may reduce the efficacy of viral clearance (Becerra-Diaz et al., 2020).
Progesterone is another sex hormone involved in the innate antiviral response. Studies in mice infected with a respiratory virus identified an increased level of progesterone, suggesting that this hormone promotes the immune response by stimulating progesterone receptors (PGRs) (Kadel and Kovats, 2018), which activate the SRC tyrosine kinase. This activation induces phosphorylation of interferon regulatory factor 3 (IRF3) at tyrosine residue 107 (Y107), promoting the activation of antiviral genes and triggering the immune response against infection (Su et al., 2022). In addition, IRF3 induces the transcription of genes encoding for type I and type III IFN, promoting this immune pathway as an antiviral defense (Mogensen, 2019).
Some respiratory viruses could be associated with host proteases to infect cells, in the case of the respiratory virus hMPV, it has been observed that the transmembrane serine protease subtype 2 (TMPRSS2) activates the fusion protein for viral entry. Moreover, this protease would be regulated by androgenic hormones through its receptors (Böttcher-Friebertshäuser, 2018). Immunostaining studies of human respiratory tract tissues such as bronchi and alveoli have observed the expression of the TMPRSS2 protein as a factor that can favor proteolytic activation and, consequently, the propagation of hMPV (Wettstein et al., 2022).
Insulin is the main anabolic hormone that regulates carbohydrates, lipids, and protein metabolism. Insulin is synthesized in pancreatic beta cells where the insulin gene encodes a single chain prohormone, which is modified in the Endoplasmic Reticulum and Golgi apparatus to generate a mature insulin hormone. These mature molecules are secreted after high glucose plasma concentrations and promote the uptake of glucose in a GLUT transporter-dependent manner (Baumgard et al., 2016). However, this insulin production and signaling could be altered under pathological conditions. The insulin effect is through the stimulation of nitric oxide production and suppression of pro-inflammatory transcription factors like NF-κB, and subsequently, the reduction of pro-inflammatory cytokines (IL-1β, IL-6, TNF-α) (Dandona et al., 2006; Dandona et al., 2009). Insulin also suppresses the gene expression of TLRs, which leads to a less inflammatory response (Dandona et al., 2009; Ghanim et al., 2008). Therefore, insulin treatment in hMPV infections could improve the symptoms and prevent energy metabolic disorders. Nano-delivery strategies (Mendes et al., 2022) could be useful to allow enhanced insulin signaling in infected airway epithelial cells.
However, insulin treatment in viral infections must be closely monitored due to the possibility of hypoglycemic events, which could worsen the individual’s health status (Ursino et al., 2024). Therefore, co-treatment with adjuvants that prevent hypoglycemic events could be evaluated in animal models to optimize insulin treatment against hMPV infections.
Insulin Resistance (IR) is a complex condition that leads to an impaired sensitivity to insulin in peripheral tissues. This condition is characterized by hyperglycemia, hyperinsulinemia, abnormal lipid accumulation, altered cellular glucose uptake, and increased lipid oxidation in adipocytes (Zhao et al., 2023). The main cause of IR is linked to an inflammatory process promoted by overnutrition, which induces the production of pro-inflammatory cytokines like IL-1β, IL-6, TNF-α, adipokines, and chemokines (Rehman and Akash, 2016). The cytokines overproduction leads to the activation of the TLRs, which also induces several pro-inflammatory transcription factors and signaling cascades NF-κB, c-Jun N-terminal kinase (JNK), inhibitor of kappa B kinase-β (IKKβ)) (Rehman and Akash, 2016; Tilg and Moschen, 2008). Hence, chronic overnutrition triggers chronic inflammation, which causes deleterious effects in beta-cell pancreatic islets, promoting insulin depletion and worsening the inflammatory state (Tilg and Moschen, 2008; Yan et al., 2024). Therefore, the energy metabolism disorders produced by IR could worsen protein turnover and facilitate viral infection.
An inflammatory process due to viral infections also requires the interaction of viral particles with the TLRs. This process also promotes the cellular activation of the transcription factor NF-κB, which leads to the production of pro-inflammatory cytokines (IL-1β, IL-6, TNF-α); this process has been described in the airway epithelial cells infected with hMPV (Kolli et al., 2012). The role of insulin as an anti-inflammatory effector has been described previously (Sun et al., 2014). Therefore, insulin could be assessed as an anti-inflammatory agent against hMPV infection.
Immunosenescence refers to the age-related decline in immune function, this process is further exacerbated by hormonal imbalances associated with aging (Arlt and Hewison, 2004). Cortisol release increases with aging, potentially weakening the immune response following an infection (Stamou et al., 2023). Conversely, melatonin, thyroid hormones, and sex hormones production decreases over time, these processes have been linked to a diminished and dysregulated immune function (Yoo et al., 2016; De Vito et al., 2011; Bupp et al., 2018). Additionally, aging disrupts insulin-related processes, often resulting in hyperinsulinemia and insulin resistance, which can compromise immune function and promote chronic inflammation (Shimi et al., 2024).
The antiviral therapy used to control hMPV infection is still being tested. It employs ribavirin, immunoglobulin, fusion inhibitors, and interfering ribonucleic acids to treat and control hMPV (Pasikhova et al., 2018; Wyde et al., 2003; Shahani et al., 2017; Panda et al., 2014; Darniot et al., 2012; Shafagati and Williams, 2018). Thus, there is currently no approved therapeutic or preventive medication for the management of hMPV infection. Vaccine development is difficult without a reliable animal model, making preclinical studies and effectiveness testing even harder (Wyde et al., 2003; Stepanova et al., 2020).
In viral infections, glucocorticoids play a role in regulating immune responses by reducing pro-inflammatory cytokines and promoting anti-inflammatory mediators through glucocorticoid receptors. Specifically, in hMPV infections, the inflammation can be managed by glucocorticoid receptor modulators (Liberman et al., 2018; Li et al., 2021). However, researchers have found mixed results when using glucocorticoids and ribavirin in severe viral illnesses like hMPV infection (Hamelin et al., 2006). A study involving combination therapy with intravenous ribavirin and immunoglobulin has shown potential to manage hMPV pneumonia, but further studies are required (Bonney et al., 2009).
Hormonal signaling pathways present promising targets for anti-inflammatory strategies in managing hMPV infections (Kino and Chrousos, 2007). This relationship is illustrated in Figure 1. For instance, estrogen signaling has been shown to enhance antiviral responses by upregulating interferon-stimulated genes (ISGs) (Uche and Guerrero-Plata, 2018), while androgen signaling can potentially suppress these effects (Zou et al., 2022). By modulating these pathways through selective estrogen receptor modulators (SERMs) or AR antagonists, it may be possible to develop more targeted therapeutic interventions (Li et al., 2022; Abramenko et al., 2021). Similarly, melatonin’s anti-inflammatory and antioxidant properties can be harnessed for reducing lung injury and oxidative stress, offering a multi-faceted approach to treating hMPV (Silvestri and Rossi, 2013; Boga et al., 2012).
Figure 1. Overview of Endogenous Hormonal Pathways and Their Interaction with the Immune System. This figure highlights key endogenous hormones and signaling pathways. In addition, it describes how endocrine signaling modulates immune responses, including inflammation and immune cell activation. Abbreviations: CRH, corticotropin-releasing hormone; TRH, thyrotropin-releasing hormone; GnRH, Gonadotropin-releasing hormone; ACTH, adrenocorticotropic hormone; TSH, thyrotropin-stimulating hormone; FSH, follicle-stimulating hormone; LH, Luteinizing hormone; NE, Noradrenaline; IL-1, Interleukin 1; IL-6, Interleukin 6; TNF- α, Tumour Necrosis Factor alpha; interferon gamma, INF-γ; and IL-2, Interleukin 2; NF-kB, nuclear factor-kappa B; MAPK, mitogen-activated protein kinase; ERK, Extracellular signal-regulated kinase; AKT, Protein kinase B; IRF3, interferon regulatory factor 3, IFN, Interferon; and NLRP3, NOD-like receptor protein 3.
Nanoparticles, due to their diminutive size, tunable properties, and capacity for information transport, enhance the stability, efficacy, and precision of therapeutic delivery and represent a promising approach to improve the delivery of hormones and antiviral molecules, potentially resulting in more effective and personalized treatments for hMPV (Singh et al., 2017). (Darniot et al., 2012; Wen and Williams, 2015). Moreover, their antiviral and biomimetic properties strengthen treatment effectiveness, and when combined with other antiviral methods, they may further improve clinical outcomes (Castañeda Cataña et al., 2025; Castañeda Cataña et al., 2024). According to other studies, nanoparticles loaded with IFN are a new choice because they are straightforward to use (inhaled dry powder) and can be delivered by the nose to children and older adults without issues (Ramos et al., 2024).
In conclusion, hMPV infection is a complex process involving intricate interactions between the virus and the host, including the modulation of the immune response by endogenous hormones through various molecular pathways. Future research should focus on personalized medicine approaches and the study of hormone-based interventions in viral respiratory diseases.
VR-P: Conceptualization, Investigation, Methodology, Writing–original draft, Writing–review and editing. SC-U: Conceptualization, Investigation, Methodology, Writing–original draft, Writing–review and editing. RT-T: Conceptualization, Investigation, Methodology, Writing–original draft, Writing–review and editing. PG-R: Conceptualization, Investigation, Methodology, Writing–original draft, Writing–review and editing. EP-C: Conceptualization, Investigation, Methodology, Writing–original draft, Writing–review and editing. MC: Conceptualization, Investigation, Methodology, Writing–original draft, Writing–review and editing. AZ: Conceptualization, Funding acquisition, Investigation, Methodology, Project administration, Supervision, Writing–original draft, Writing–review and editing.
The author(s) declare that financial support was received for the research, authorship, and/or publication of this article. The experimentation and publication fee of this article are funded by Universidad UTE.
We are grateful to Universidad UTE for supporting the researchers.
The authors declare that the research was conducted in the absence of any commercial or financial relationships that could be construed as a potential conflict of interest.
The authors declare that no Generative AI was used in the creation of this manuscript.
All claims expressed in this article are solely those of the authors and do not necessarily represent those of their affiliated organizations, or those of the publisher, the editors and the reviewers. Any product that may be evaluated in this article, or claim that may be made by its manufacturer, is not guaranteed or endorsed by the publisher.
Abramenko, N., Vellieux, F., Tesařová, P., Kejík, Z., Kaplánek, R., Lacina, L., et al. (2021). Estrogen receptor modulators in viral infections such as sars−cov−2: therapeutic consequences. Int. J. Mol. Sci. 22, 6551. doi:10.3390/ijms22126551
Acevedo-Rodriguez, A., Kauffman, A. S., Cherrington, B. D., Borges, C. S., Roepke, T. A., and Laconi, M. (2018). Emerging insights into hypothalamic-pituitary-gonadal axis regulation and interaction with stress signalling. J. Neuroendocrinol. 30 (10), e12590. doi:10.1111/jne.12590
Aranda, A. (2025). Thyroid hormone action by genomic and nongenomic molecular mechanisms. Methods Mol. Biol. 2876, 17–34. doi:10.1007/978-1-0716-4252-8_2
Arlt, W., and Hewison, M. (2004). Hormones and immune function: implications of aging. Aging Cell 3, 209–216. doi:10.1111/j.1474-9728.2004.00109.x
Astapova, I., Vella, K. R., Ramadoss, P., Holtz, K. A., Rodwin, B. A., Liao, X. H., et al. (2011). The nuclear receptor corepressor (NCoR) controls thyroid hormone sensitivity and the set point of the hypothalamic-pituitary-thyroid axis. Mol. Endocrinol. 25 (2), 212–224. doi:10.1210/me.2010-0462
Bahrampour Juybari, K., Pourhanifeh, M. H., Hosseinzadeh, A., Hemati, K., and Mehrzadi, S. (2020). Melatonin potentials against viral infections including COVID-19: current evidence and new findings. Virus Res. 287, 198108. doi:10.1016/j.virusres.2020.198108
Bao, X., Liu, T., Shan, Y., Li, K., Garofalo, R. P., and Casola, A. (2008). Human metapneumovirus glycoprotein G inhibits innate immune responses. PLoS Pathog. 4 (5), e1000077. doi:10.1371/journal.ppat.1000077
Baumgard, L. H., Hausman, G. J., and Sanz Fernandez, M. V. (2016). Insulin: pancreatic secretion and adipocyte regulation. Domest. Anim. Endocrinol. 54, 76–84. doi:10.1016/j.domaniend.2015.07.001
Becerra-Diaz, M., Song, M., and Heller, N. (2020). Androgen and androgen receptors as regulators of monocyte and macrophage biology in the healthy and diseased lung. Front. Immunol. 11, 1698. doi:10.3389/fimmu.2020.01698
Boga, J. A., Coto-Montes, A., Rosales-Corral, S. A., Tan, D. X., and Reiter, R. J. (2012). Beneficial actions of melatonin in the management of viral infections: a new use for this “molecular handyman”. Rev. Med. Virol. 22 (5), 323–338. doi:10.1002/rmv.1714
Bohmwald, K., Gálvez, N. M. S., Ríos, M., and Kalergis, A. M. (2018). Neurologic alterations due to respiratory virus infections. Front. Cell Neurosci. 12, 386. doi:10.3389/fncel.2018.00386
Bonney, D., Razali, H., Turner, A., and Will, A. (2009). Successful treatment of human metapneumovirus pneumonia using combination therapy with intravenous ribavirin and immune globulin. Br. J. Haematol. 145 (5), 667–669. doi:10.1111/j.1365-2141.2009.07654.x
Borghetti, P., Saleri, R., Mocchegiani, E., Corradi, A., and Martelli, P. (2009). Infection, immunity and the neuroendocrine response. Vet. Immunol. Immunopathol. 130 (3), 141–162. doi:10.1016/j.vetimm.2009.01.013
Böttcher-Friebertshäuser, E. (2018). “Membrane-anchored serine proteases: host cell factors in proteolytic activation of viral glycoproteins,” in Activation of viruses by host proteases (Springer International Publishing), 153–203.
Brent, G. A. (2012). Mechanisms of thyroid hormone action. J. Clin. Investigation 122 (9), 3035–3043. doi:10.1172/JCI60047
Bupp, M. R. G., Potluri, T., Fink, A. L., and Klein, S. L. (2018). The confluence of sex hormones and aging on immunity. Front. Immunol. 9, 1269. doi:10.3389/fimmu.2018.01269
Castañeda Cataña, M. A., Dodes Traian, M. M., Rivas Marquina, A. P., Marquez, A. B., Arrúa, E. C., Carlucci, M. J., et al. (2024). Design and characterization of BSA-mycophenolic acid nanocomplexes: antiviral activity exploration. Int. J. Biol. Macromol. 265 (Pt 2), 131023. doi:10.1016/j.ijbiomac.2024.131023
Castañeda Cataña, M. A., Rivas Marquina, A. P., Dodes Traian, M. M., Carlucci, M. J., Damonte, E. B., Pérez, O. E., et al. (2025). Bovine serum albumin nanoparticle-mediated delivery of ribavirin and mycophenolic acid for enhanced antiviral therapeutics. Viruses 17 (2), 138. doi:10.3390/v17020138
Chan, L. P., Liu, C., Chiang, F. Y., Wang, L. F., Lee, K. W., Chen, W. T., et al. (2017). IL-8 promotes inflammatory mediators and stimulates activation of p38 MAPK/ERK-NF-κB pathway and reduction of JNK in HNSCC. Oncotarget 8, 56375–56388. doi:10.18632/oncotarget.16914
Cox, R. G., Mainou, B. A., Johnson, M., Hastings, A. K., Schuster, J. E., Dermody, T. S., et al. (2015). Human metapneumovirus is capable of entering cells by fusion with endosomal membranes. PLoS Pathog. 11 (12), e1005303. doi:10.1371/journal.ppat.1005303
Dandona, P., Chaudhuri, A., Ghanim, H., and Mohanty, P. (2006). Anti-inflammatory effects of insulin and the pro-inflammatory effects of glucose. Semin. Thorac. Cardiovasc Surg. 18 (4), 293–301. doi:10.1053/j.semtcvs.2006.06.003
Dandona, P., Chaudhuri, A., Ghanim, H., and Mohanty, P. (2009). Insulin as an anti-inflammatory and antiatherogenic modulator. J. Am. Coll. Cardiol. 53 (5), S14–S20. doi:10.1016/j.jacc.2008.10.038
Darniot, M., Schildgen, V., Schildgen, O., Sproat, B., Kleines, M., Ditt, V., et al. (2012). RNA interference in vitro and in vivo using DsiRNA targeting the nucleocapsid N mRNA of human metapneumovirus. Antivir. Res. 93 (3), 364–373. doi:10.1016/j.antiviral.2012.01.004
Daryani, A., Montazeri, M., Pagheh, A. S., Sharif, M., Sarvi, S., Hosseinzadeh, A., et al. (2018). The potential use of melatonin to treat protozoan parasitic infections: a review. Biomed. and Pharmacother. 97, 948–957. doi:10.1016/j.biopha.2017.11.007
Delchev, S., and Georgieva, K. (2018). “Cellular and molecular mechanisms of the effects of sex hormones on the nervous system,” in Sex hormones in neurodegenerative processes and diseases (London, United Kingdom: InTech).
De Vito, P., Incerpi, S., Pedersen, J. Z., Luly, P., Davis, F. B., and Davis, P. J. (2011). Thyroid hormones as modulators of immune activities at the cellular level. Thyroid 21 (8), 879–890. doi:10.1089/thy.2010.0429
Feldt-Rasmussen, U., Effraimidis, G., and Klose, M. (2021). The hypothalamus-pituitary-thyroid (HPT)-axis and its role in physiology and pathophysiology of other hypothalamus-pituitary functions. Mol. Cell Endocrinol., 525. doi:10.1016/j.mce.2021.111173
Funes, S. C., Ríos, M., Fernández-Fierro, A., Rivera-Pérez, D., Soto, J. A., Valbuena, J. R., et al. (2022). Female offspring gestated in hypothyroxinemia and infected with human Metapneumovirus (hMPV) suffer a more severe infection and have a higher number of activated CD8+ T lymphocytes. Front. Immunol. 13, 966917. doi:10.3389/fimmu.2022.966917
Ghanim, H., Mohanty, P., Deopurkar, R., Sia, C. L., Korzeniewski, K., Abuaysheh, S., et al. (2008). Acute modulation of toll-like receptors by insulin. Diabetes Care 31 (9), 1827–1831. doi:10.2337/dc08-0561
Ghosh, S., and Klein, R. S. (2017). Sex drives dimorphic immune responses to viral infections. J. Immunol. 198 (5), 1782–1790. doi:10.4049/jimmunol.1601166
Gjerstad, J. K., Lightman, S. L., and Spiga, F. (2018). Role of glucocorticoid negative feedback in the regulation of HPA axis pulsatility. Stress 21 (5), 403–416. doi:10.1080/10253890.2018.1470238
Guevara-Ramírez, P., Paz-Cruz, E., Cadena-Ullauri, S., Ruiz-Pozo, V. A., Tamayo-Trujillo, R., Felix, M. L., et al. (2023). Molecular pathways and nutrigenomic review of insulin resistance development in gestational diabetes mellitus. Front. Nutr. 10, 1228703. doi:10.3389/fnut.2023.1228703
Hamelin, M. È., Prince, G. A., and Boivin, G. (2006). Effect of ribavirin and glucocorticoid treatment in a mouse model of human metapneumovirus infection. Antimicrob. Agents Chemother. 50 (2), 774–777. doi:10.1128/AAC.50.2.774-777.2006
Herman, J. P., McKlveen, J. M., Ghosal, S., Kopp, B., Wulsin, A., Makinson, R., et al. (2016). Regulation of the hypothalamic-pituitary-adrenocortical stress response. Compr. Physiol. 6 (2), 603–621. doi:10.1002/cphy.c150015
Hosseinzadeh, A., Kamrava, S. K., Joghataei, M. T., Darabi, R., Shakeri-Zadeh, A., Shahriari, M., et al. (2016). Apoptosis signaling pathways in osteoarthritis and possible protective role of melatonin. J. Pineal Res. 61 (4), 411–425. doi:10.1111/jpi.12362
Hu, J., Zhang, Z., Shen, W. J., and Azhar, S. (2010). Cellular cholesterol delivery, intracellular processing and utilization for biosynthesis of steroid hormones. Nutr. and Metabolism 7, 47. doi:10.1186/1743-7075-7-47
Hu, Q., Bian, Q., Rong, D., Wang, L., Song, J., Huang, H. S., et al. (2023). JAK/STAT pathway: Extracellular signals, diseases, immunity, and therapeutic regimens. Front. Bioeng. Biotechnol. 11, 1110765. doi:10.3389/fbioe.2023.1110765
Jeannet, N., Van Den Hoogen, B. G., Schefold, J. C., Suter-Riniker, F., Sommerstein, R., Jeannet, N., et al. (2017). Cerebrospinal fluid findings in an adult with human metapneumovirus–associated encephalitis. Emerg. Infect. Dis. 23, 370. doi:10.3201/eid2302.161337
Kadel, S., and Kovats, S. (2018). Sex hormones regulate innate immune cells and promote sex differences in respiratory virus infection. Front. Immunol. 9, 1653. doi:10.3389/fimmu.2018.01653
Kalergis, A. M., bohmwald, K., and Andrade, C. A. (2023). Pneumoviruses can impair the central nervous system by different mechanisms. J. Immunol. 210 (1_Suppl), 236.22. doi:10.4049/jimmunol.210.supp.236.22
Kino, T., and Chrousos, G. P. (2007). Virus-mediated modulation of the host endocrine signaling systems: clinical implications. Trends Endocrinol. Metabolism 18, 159–166. doi:10.1016/j.tem.2007.03.003
Kolli, D., Bao, X., and Casola, A. (2012). Human metapneumovirus antagonism of innate immune responses. Viruses 4 (12), 3551–3571. doi:10.3390/v4123551
Lasa, M., Contreras-Jurado, C., Incerpi, S., Teng, W., and Di, C. E. (2022). Thyroid hormones act as modulators of inflammation through their nuclear receptors. Front. Endocrinol. (Lausanne) 13, 937099. doi:10.3389/fendo.2022.937099
Le, V. B., Dubois, J., Couture, C., Cavanagh, M. H., Uyar, O., Pizzorno, A., et al. (2019). Human metapneumovirus activates NOD-like receptor protein 3 inflammasome via its small hydrophobic protein which plays a detrimental role during infection in mice. PLoS Pathog. 15 (4), e1007689. doi:10.1371/journal.ppat.1007689
Li, F., Boon, A. C. M., Michelson, A. P., Foraker, R. E., Zhan, M., and Payne, P. R. O. (2022). Estrogen hormone is an essential sex factor inhibiting inflammation and immune response in COVID-19. Sci. Rep. 12 (1), 9462. doi:10.1038/s41598-022-13585-4
Li, J., Liao, X., Zhou, Y., Wang, L., Yang, H., Zhang, W., et al. (2021). Association between glucocorticoids treatment and viral clearance delay in patients with COVID-19: a systematic review and meta-analysis. BMC Infect. Dis. 21 (1), 1063. doi:10.1186/s12879-021-06548-z
Liberman, A. C., Budziñski, M. L., Sokn, C., Gobbini, R. P., Steininger, A., and Arzt, E. (2018). Regulatory and mechanistic actions of glucocorticoids on T and inflammatory cells. Front. Endocrinol. (Lausanne) 9, 235. doi:10.3389/fendo.2018.00235
Lomauro, A., and Aliverti, A. (2021). Sex and gender in respiratory physiology. Eur. Respir. Rev. 30 (162), 210038. doi:10.1183/16000617.0038-2021
Lupfer, C., Kuriakose, T., Han, C., Zhao, W., and Zhao, C. (2020). NLRP3 inflammasome—a key player in antiviral responses. Front. Immunol. 11, 211. doi:10.3389/fimmu.2020.00211
Mebis, L., and van den Berghe, G. (2009). The hypothalamus-pituitary-thyroid axis in critical illness. Neth. J. Med. 67, 332–340.
Mendes, B. B., Conniot, J., Avital, A., Yao, D., Jiang, X., Zhou, X., et al. (2022). Nanodelivery of nucleic acids. Nat. Rev. Methods Prim. 2 (1), 24–21. doi:10.1038/s43586-022-00104-y
Mogensen, T. H. (2019). IRF and STAT transcription factors - from basic biology to roles in infection, protective immunity, and primary immunodeficiencies. Front. Immunol. 10. doi:10.3389/fimmu.2018.03047
Oketch, J. W., Kamau, E., Otieno, G. P., Otieno, J. R., Agoti, C. N., and Nokes, D. J. (2019). Human metapneumovirus prevalence and patterns of subgroup persistence identified through surveillance of pediatric pneumonia hospital admissions in coastal Kenya, 2007-2016. BMC Infect. Dis. 19 (1), 757. doi:10.1186/s12879-019-4381-9
Panda, S., Mohakud, N. K., Pena, L., and Kumar, S. (2014). Human metapneumovirus: review of an important respiratory pathogen. Int. J. Infect. Dis. 25, 45–52. doi:10.1016/j.ijid.2014.03.1394
Pasikhova, Y., Hayne, J., and Baluch, A. (2018). Oral ribavirin for the treatment of respiratory syncytial virus (RSV) and human metapneumovirus (hMPV) infections in hematology patients and stem cell transplant (SCT) recipients at a nci-designated cancer center. Biol. Blood Marrow Transplant. 24 (3), S383. doi:10.1016/j.bbmt.2017.12.471
Piñana, J. L., Tridello, G., Xhaard, A., Wendel, L., Montoro, J., Vazquez, L., et al. (2024). Upper and/or lower respiratory tract infection caused by human metapneumovirus after allogeneic hematopoietic stem cell transplantation. J. Infect. Dis. 229 (1), 83–94. doi:10.1093/infdis/jiad268
Ramos, T. I., Villacis-Aguirre, C. A., Sandoval, F. S., Martin-Solano, S., Manrique-Suárez, V., Rodríguez, H., et al. (2024). Multilayer nanocarrier for the codelivery of interferons: a promising strategy for biocompatible and long-acting antiviral treatment. Pharmaceutics 16 (11), 1349. doi:10.3390/pharmaceutics16111349
Rehman, K., and Akash, M. S. H. (2016). Mechanisms of inflammatory responses and development of insulin resistance: how are they interlinked? J. Biomed. Sci. 23 (1), 87–18. doi:10.1186/s12929-016-0303-y
Rubingh, J., van der Spek, A., Fliers, E., and Boelen, A. (2020). The role of thyroid hormone in the innate and adaptive immune response during infection. Compr. Physiol. 10 (4), 1277–1287. doi:10.1002/cphy.c200003
Ruiz-Pozo, V. A., Paz-Cruz, E., Cadena-Ullauri, S., Tamayo-Trujillo, R., Guevara-Ramírez, P., Simancas-Racines, D., et al. (2024). Effect of diet on the microbiota and immune system in patients with systemic lupus erythematosus. Food Agric. Immunol. 35 (1). doi:10.1080/09540105.2024.2434475
Ruiz-Pozo, V. A., Tamayo-Trujillo, R., Cadena-Ullauri, S., Frias-Toral, E., Guevara-Ramírez, P., Paz-Cruz, E., et al. (2023). The molecular mechanisms of the relationship between insulin resistance and Parkinson’s disease pathogenesis. Nutrients 15, 3585, doi:10.3390/nu15163585
Schildgen, O., Glatzel, T., Geikowski, T., Scheibner, B., Matz, B., Bindl, L., et al. (2005). Human metapneumovirus RNA in encephalitis patient. Emerg. Infect. Dis. 11 (3), 467–470. doi:10.3201/eid1103.040676
Sciarra, F., Campolo, F., Franceschini, E., Carlomagno, F., and Venneri, M. A. (2023). Gender-specific impact of sex hormones on the immune system. Int. J. Mol. Sci. 24, 6302. doi:10.3390/ijms24076302
Seth, R. B., Sun, L., Ea, C. K., and Chen, Z. J. (2005). Identification and characterization of MAVS, a mitochondrial antiviral signaling protein that activates NF-kappaB and IRF 3. Cell 122 (5), 669–682. doi:10.1016/j.cell.2005.08.012
Shafagati, N., and Williams, J. (2018). Human metapneumovirus - what we know now. F1000Res 7, 135. doi:10.12688/f1000research.12625.1
Shahani, L., Ariza-Heredia, E. J., and Chemaly, R. F. (2017). Antiviral therapy for respiratory viral infections in immunocompromised patients. Expert Rev. Anti Infect. Ther. 15 (4), 401–415. doi:10.1080/14787210.2017.1279970
Shimi, G., Sohouli, M. H., Ghorbani, A., Shakery, A., and Zand, H. (2024). The interplay between obesity, immunosenescence, and insulin resistance. Immun. Ageing. 21, 13. doi:10.1186/s12979-024-00414-7
Silverman, M. N., Pearce, B. D., Biron, C. A., and Miller, A. H. (2005). Immune modulation of the hypothalamic-pituitary-adrenal (HPA) Axis during viral infection. Viral Immunol. 18 (1), 41–78. doi:10.1089/vim.2005.18.41
Silvestri, M., and Rossi, G. A. (2013). Melatonin: its possible role in the management of viral infections-a brief review. Ital. J. Pediatr. 39 (1), 61. doi:10.1186/1824-7288-39-61
Singh, L., Kruger, H. G., Maguire, G. E. M., Govender, T., and Parboosing, R. (2017). The role of nanotechnology in the treatment of viral infections. Ther. Adv. Infect. Dis. 4 (4), 105–131. doi:10.1177/2049936117713593
Somasundaram, N. P., and Gunatilake, S. S. C. (2021). Infections in endocrinology: viruses. South Dartmouth, EE.UU: Endotext. Available online at: https://www.ncbi.nlm.nih.gov/books/NBK568565/.
Soto, J. A., Gálvez, N. M. S., Benavente, F. M., Pizarro-Ortega, M. S., Lay, M. K., Riedel, C., et al. (2018). Human metapneumovirus: mechanisms and molecular targets used by the virus to avoid the immune system. Front. Immunol. 9, 2466. doi:10.3389/fimmu.2018.02466
Stamou, M. I., Colling, C., and Dichtel, L. E. (2023). Adrenal aging and its effects on the stress response and immunosenescence. Maturitas 168, 13–19. doi:10.1016/j.maturitas.2022.10.006
Stepanova, E., Matyushenko, V., Rudenko, L., and Isakova-Sivak, I. (2020). Prospects of and barriers to the development of epitope-based vaccines against human metapneumovirus. Pathogens 9 (6), 481. doi:10.3390/pathogens9060481
Su, S., Hua, D., Li, J. P., Zhang, X. N., Bai, L., Cao, L. B., et al. (2022). Modulation of innate immune response to viruses including SARS-CoV-2 by progesterone. Signal Transduct. Target Ther. 7 (1), 137. doi:10.1038/s41392-022-00981-5
Sun, Q., Li, J., and Gao, F. (2014). New insights into insulin: the anti-inflammatory effect and its clinical relevance. World J. Diabetes 5 (2), 89–96. doi:10.4239/wjd.v5.i2.89
Sunstrum, J. K., and Inoue, W. (2019). Heterosynaptic modulation in the paraventricular nucleus of the hypothalamus. Neuropharmacology 154, 87–95. doi:10.1016/j.neuropharm.2018.11.004
Takatsu, K., Kouro, T., and Nagai, Y. (2009). Interleukin 5 in the link between the innate and acquired immune response. Advances in immunology 101, 191–236. doi:10.1016/S0065-2776(08)01006-7
Tilg, H., and Moschen, A. R. (2008). Inflammatory mechanisms in the regulation of insulin resistance. Mol. Med. 14 (3), 222–231. doi:10.2119/2007-00119.Tilg
Uche, I. K., and Guerrero-Plata, A. (2018). Interferon-mediated response to human metapneumovirus infection. Viruses 10 (9), 505. doi:10.3390/v10090505
Ursino, G., Lucibello, G., Teixeira, P. D. S., Höfler, A., Veyrat-Durebex, C., Odouard, S., et al. (2024). S100A9 exerts insulin-independent antidiabetic and anti-inflammatory effects. Sci. Adv. 10 (1), 4686. doi:10.1126/sciadv.adj4686
Van Den Bergh, A., Bailly, B., Guillon, P., von Itzstein, M., and Dirr, L. (2024). Novel insights into the host cell glycan binding profile of human metapneumovirus. J. Virol. 98 (6), e0164123. doi:10.1128/jvi.01641-23
Velayutham, T. S., Kolli, D., Ivanciuc, T., Garofalo, R. P., and Casola, A. (2013). Critical role of TLR4 in human metapneumovirus mediated innate immune responses and disease pathogenesis. PLoS One 8 (10), e78849. doi:10.1371/journal.pone.0078849
Wen, S. C., and Williams, J. V. (2015). New approaches for immunization and therapy against human metapneumovirus. Clin. Vaccine Immunol. 22 (8), 858–866. doi:10.1128/CVI.00230-15
Weng, C. Y., Lin, T. K., and Hsu, B. C. (2023). “Psychopathophysiology and compassion-based cognitive-behavior group therapy for patients with coronary artery disease,” in Handbook of cognitive behavioral therapy by disorder: case studies and application for adults, 307–320.
Wenzek, C., Boelen, A., Westendorf, A. M., Engel, D. R., Moeller, L. C., and Führer, D. (2022). The interplay of thyroid hormones and the immune system-where we stand and why we need to know about it. Eur. J. Endocrinol. 186, R65–R77. doi:10.1530/EJE-21-1171
Wettstein, L., Kirchhoff, F., and Münch, J. (2022). The transmembrane protease TMPRSS2 as a therapeutic target for COVID-19 treatment. Int. J. Mol. Sci. 23 (3), 1351. doi:10.3390/ijms23031351
Wu, H. M., Zhao, C. C., Xie, Q. M., Xu, J., and Fei, G. H. (2020). TLR2-Melatonin feedback loop regulates the activation of NLRP3 inflammasome in murine allergic airway inflammation. Front. Immunol. 11, 172. doi:10.3389/fimmu.2020.00172
Wyde, P. R., Chetty, S. N., Jewell, A. M., Boivin, G., and Piedra, P. A. (2003). Comparison of the inhibition of human metapneumovirus and respiratory syncytial virus by ribavirin and immune serum globulin in vitro. Antivir. Res. 60 (1), 51–59. doi:10.1016/s0166-3542(03)00153-0
Yan, S., Santoro, A., Niphakis, M. J., Pinto, A. M., Jacobs, C. L., Ahmad, R., et al. (2024). Inflammation causes insulin resistance in mice via interferon regulatory factor 3 (IRF3)-mediated reduction in FAHFA levels. Nat. Commun. 15 (1), 4605–4619. doi:10.1038/s41467-024-48220-5
Yoo, Y. M., Jang, S. K., Kim, G. H., Park, J. Y., and Joo, S. S. (2016). Pharmacological advantages of melatonin in immunosenescence by improving activity of T lymphocytes. J. Biomed. Res. 30 (4), 314–321. doi:10.7555/JBR.30.2016K0010
Zhang, Y., Li, X., Grailer, J. J., Wang, N., Wang, M., Yao, J., et al. (2016). Melatonin alleviates acute lung injury through inhibiting the NLRP3 inflammasome. J. Pineal Res. 60 (4), 405–414. doi:10.1111/jpi.12322
Zhang, Y., Xu, J., Miranda-Katz, M., Sojati, J., Tollefson, S. J., Manni, M. L., et al. (2024). Distinct roles for type I and type III interferons in virulent human metapneumovirus pathogenesis. PLoS Pathog. 20 (2 February), e1011840. doi:10.1371/journal.ppat.1011840
Zhao, X., An, X., Yang, C., Sun, W., Ji, H., and Lian, F. (2023). The crucial role and mechanism of insulin resistance in metabolic disease. Front. Endocrinol. (Lausanne) 14, 1149239. doi:10.3389/fendo.2023.1149239
Keywords: healthcare, disease prevention, epidemiology, inflammation, human metapneumovirus, hormones
Citation: Ruiz-Pozo VA, Cadena-Ullauri S, Tamayo-Trujillo R, Guevara-Ramírez P, Paz-Cruz E, Castañeda Cataña MA and Zambrano AK (2025) Interplay between endogenous hormones and immune systems in human metapneumovirus pathogenesis and management. Front. Pharmacol. 16:1568828. doi: 10.3389/fphar.2025.1568828
Received: 30 January 2025; Accepted: 03 March 2025;
Published: 19 March 2025.
Edited by:
Alana Aragón-Herrera, Health Research Institute of Santiago de Compostela (IDIS), SpainReviewed by:
Oliver Schildgen, Kliniken der Stadt Köln, GermanyCopyright © 2025 Ruiz-Pozo, Cadena-Ullauri, Tamayo-Trujillo, Guevara-Ramírez, Paz-Cruz, Castañeda Cataña and Zambrano. This is an open-access article distributed under the terms of the Creative Commons Attribution License (CC BY). The use, distribution or reproduction in other forums is permitted, provided the original author(s) and the copyright owner(s) are credited and that the original publication in this journal is cited, in accordance with accepted academic practice. No use, distribution or reproduction is permitted which does not comply with these terms.
*Correspondence: Ana Karina Zambrano, YW5hemFtYnJhbm8xN0Bob3RtYWlsLmNvbQ==
†These authors have contributed equally to this work and share first authorship
Disclaimer: All claims expressed in this article are solely those of the authors and do not necessarily represent those of their affiliated organizations, or those of the publisher, the editors and the reviewers. Any product that may be evaluated in this article or claim that may be made by its manufacturer is not guaranteed or endorsed by the publisher.
Research integrity at Frontiers
Learn more about the work of our research integrity team to safeguard the quality of each article we publish.