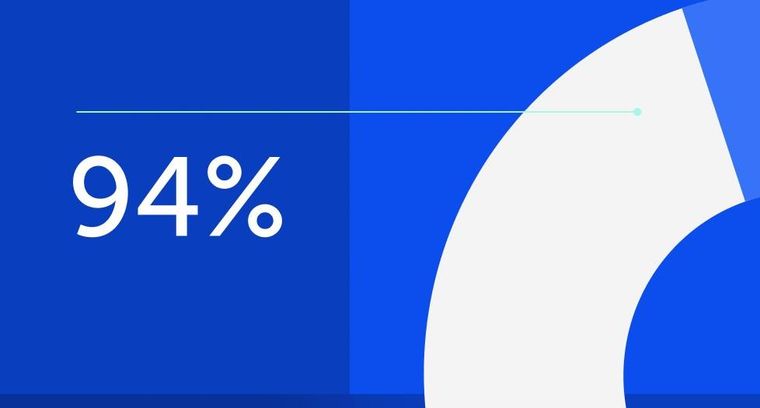
94% of researchers rate our articles as excellent or good
Learn more about the work of our research integrity team to safeguard the quality of each article we publish.
Find out more
REVIEW article
Front. Pharmacol., 17 April 2025
Sec. Cardiovascular and Smooth Muscle Pharmacology
Volume 16 - 2025 | https://doi.org/10.3389/fphar.2025.1567312
Doxorubicin (DOX) has received widespread attention as a broad-spectrum antitumor drug. However, it has been a recognized challenge that long-term DOX injections can lead to severe cardiotoxicity. There are numerous interventions to DOX-induced cardiotoxicity, and the most cost-effective is phytochemicals. It has been reported that phytochemicals have complex and diverse biological properties, facilitating the mitigation of DOX-induced cardiotoxicity. DOX-induced cardiotoxicity has numerous pathological mechanisms, and the nod-like receptor family pyrin domain-containing 3 (NLRP3) inflammasome-mediated cardiomyocyte pyroptosis is one of them. This review initially presents an overview of the pathological mechanisms that underlie cardiotoxicity induced by DOX. Subsequently, we present a comprehensive elucidation of the structure and activation of the NLRP3 inflammasome. Finally, we provide a detailed summary of phytochemicals that can mitigate DOX-induced cardiotoxicity by influencing the expression of the NLRP3 inflammasome in cardiomyocytes.
Doxorubicin (DOX) is recognized for its remarkable antitumor efficacy (Agudelo et al., 2014; Xu et al., 2024). However, it is essential to acknowledge that extended administration of DOX can result in significant organ damage, particularly affecting the heart (Carvalho et al., 2009; Wu et al., 2022; Zhao et al., 2023). Available clinical data suggests that prolonged administration of DOX leads to reduced left ventricular ejection fraction (LVEF), arrhythmias and in severe cases, even heart failure (Lipshultz et al., 2002; Volkova and Russell, 2011; Milano et al., 2014; Gupta et al., 2019; Tan et al., 2021; Yun et al., 2021; Harding et al., 2023; Hu et al., 2023; Zhu M. et al., 2024). Recent epidemiologic data indicates that the toxicity of DOX on the heart increases with dose, with a probability of heart failure exceeding 40% for patients when the cumulative dose reaches 700 mg/m2 (Gianni et al., 2008; Curigliano et al., 2016). This highly morbid and lethal disease imposes a significant strain on the family of patient and society. Therefore, it is urgent to find appropriate ways to intervene in DOX-induced cardiotoxicity.
Contemporary treatments for DOX-induced cardiotoxicity mainly involve clinical drugs (Zilinyi et al., 2018). Although their efficacy is unquestionably assured, most patients cannot afford the high cost, while long-term administration can lead to adverse effects. Currently, there are several studies indicating that phytochemicals can enhance cardiovascular health (Ditano-Vázquez et al., 2019; Wen et al., 2024). Moreover, numerous investigations have confirmed the potential efficacy of this cost-effective medication in mitigating DOX-induced cardiotoxicity (Abushouk et al., 2017).
DOX-induced cardiotoxicity encompasses various pathological processes, with the nod-like receptor family pyrin domain-containing 3 (NLRP3) inflammasome-mediated cellular pyroptosis being among them and currently one of the most extensively researched (Rawat et al., 2021; Yang et al., 2022). It has been extensively documented that many phytochemicals can reduce DOX-induced cardiotoxicity by affecting the NLRP3 inflammasome and thereby ameliorating cardiomyocyte pyroptosis. This review will summarize and discuss this aspect in detail.
When screening the core literature, we used the following search terms: (((heart*) OR (cardia*) OR (myocardi*) OR (cardiomyocyte*) OR (cardiomyoblast*) OR (cardiomyopathy) OR (H9c2) OR (cardiotoxicity)) AND ((Doxorubicin) OR (DOX))) AND ((NLRP3) OR (nucleotide-binding oligomerization domain-like receptor protein 3)). Using this search strategy, we screened out all research articles that included phytochemicals.
Recent studies have been devoted to digging deeper into the pathological mechanisms of DOX-induced cardiotoxicity. Although there has been significant progress, there are still many controversial contents. In this section, we will condense the pathological mechanisms of DOX-induced cardiotoxicity based on the contents of the existing literature.
The first pathological mechanism we will discuss is oxidative stress (Songbo et al., 2019). According to the available studies, DOX can induce oxidative stress in cardiomyocytes in three manners. At the very beginning, DOX can damage the “energy supply centers” in cardiomyocytes, i.e., mitochondria, which can cause the accumulation of reactive oxygen species (ROS) (Octavia et al., 2012). Second, DOX can induce ROS production by affecting Fe2+ homeostasis in cardiomyocytes (Sangweni et al., 2022). Finally, DOX can also promote ROS accumulation in cardiomyocytes by increasing nicotinamide adenine nucleotide phosphate (NADPH) oxidase, which in turn induces oxidative stress injury in cardiomyocytes (Priya et al., 2017). Multiple programmed cell deaths are also significant for DOX-induced cardiotoxicity (Christidi and Brunham, 2021). Cardiomyocyte apoptosis is one of them (Qu et al., 2022). Two pathways mainly participate in DOX-induced cardiomyocyte apoptosis. The first is the endogenous pathway (i.e., the mitochondrial pathway), in which DOX can lead to DNA fragmentation by disrupting the outer mitochondrial membrane (OMM) in cardiomyocytes, which can cause the leakage of cytochrome C (Cyt C), which recruits caspase-9 and in turn activates caspase-3 and induces its translocation to the nucleus (Kitazumi and Tsukahara, 2011). The exogenous apoptotic pathway is mainly closely linked to the activation of caspase-8. DOX can activate caspase-3/7 by activating caspase-8, which in turn leads to the development of cardiomyocyte apoptosis (Font-Belmonte et al., 2020). Cardiomyocyte ferroptosis is also one of them (Tai et al., 2023). Available evidence suggests that DOX can induce cardiomyocyte ferroptosis by inducing a massive accumulation of Fe2+, thereby disrupting iron homeostasis within cardiomyocytes (Zhao et al., 2023). Meanwhile, excessive Fe2+ accumulation induces the Fenton Reaction, which leads to a massive accumulation of lipid peroxides, causing secondary damage (Koppenol, 2001; Kaźmierczak-Barańska et al., 2020; Ai et al., 2021). Cellular autophagy, a particular form of programmed cell death, also participates in DOX-induced cardiotoxicity (Shabalala et al., 2017). In addition, the induction of cellular inflammation is also an important factor in myocardial injury caused by DOX (Vitale et al., 2024). According to relevant reports, DOX can induce the expression of pro-inflammatory factors [e.g., interleukin-1 beta (IL-1β), tumor necrosis factor-alpha (TNF-α), etc.], which induces myocardial cell inflammation (El-Agamy et al., 2019; Li et al., 2019). Of course, many other pathological mechanisms are involved besides the above. For example, DOX can induce endoplasmic reticulum dysfunction in cardiomyocytes, leading to the accumulation of unfolded or misfolded proteins, which in turn induces endoplasmic reticulum stress injury (Sun et al., 2024). DOX also causes damage to mitochondria in cardiomyocytes, leading to mitochondrial dysfunction (Wu et al., 2022). The pathological mechanism of DOX-induced cardiotoxicity may also be related to epigenetic, Ca2+ overload and disturbed energy metabolism (Chen et al., 2017; Rawat et al., 2021; Dantas et al., 2022).
Notably, cellular pyroptosis mediated by NLRP3 inflammasome has received much attention, and many reports suggest that DOX-induced cardiotoxicity is intimately linked to the activation of NLRP3 inflammasome-mediated cardiomyocytes pyroptosis. Therefore, our review will target NLRP3 inflammasome-mediated cardiomyocyte pyroptosis and provide insights into phytochemicals to ameliorate DOX-induced cardiotoxicity. Figure 1 illustrates the pathological processes of DOX-induced cardiotoxicity.
Figure 1. The main pathological mechanisms of doxorubicin-induced cardiotoxicity. Doxorubicin-induced cardiotoxicity involves multiple pathological mechanisms. The main can include oxidative stress, apoptosis, ferroptosis, pyroptosis, autophagy, inflammation, endoplasmic reticulum stress, epigenetics, Ca2+ overload, disturbed energy metabolism and mitochondrial dysfunction (Created with BioRender.com).
Nucleotide-binding structural domains Leucine-rich repeat proteins (NLRs) constitute a family of pattern recognition receptors (PRRs) predominantly engaged in innate immune defense mechanisms, responding to various damage-associated molecular patterns (DAMPs) and pathogen-associated molecular patterns (PAMPs) (Singh and Jha, 2018; Xia et al., 2023). Notably, there are four subgroups within the NLR family: NLRA, NLRB, NLRC, and NLRP (Xia et al., 2023). Activated NLRPs can form inflammasomes, of which the NLRP3 inflammasome is representative (Wicherska-Pawłowska et al., 2021; Xia et al., 2023). Available reports suggest that the NLRP3 inflammasome comprises three parts: NLRP3 (sensor), apoptosis-associated speckle-like protein (ASC) (adaptor) and caspase-1 (effector) (Li S. et al., 2021). Nucleotide-binding and oligomerization domain (NACHT), C-terminal leucine-rich repeats (LRRs) and an N-terminal pyrin domain (PYD) are the three primary components of NLRP3 (Platnich and Muruve, 2019; Yi, 2020; Li S. et al., 2021; Xia et al., 2023). ASC contains two domains: one at the N-terminal end, the PYD, and the other, the C-terminal caspase recruit domain (CARD) (Platnich and Muruve, 2019; Yi, 2020). Pro-caspase-1 possesses a three-part structure comprising a CARD at the N-terminal, p10 at the C-terminal and p20 centrally located (Platnich and Muruve, 2019; Yi, 2020).
The NLRP3 inflammasome rests by forming a double-loop structure (Andreeva et al., 2021). The NLRP3 inflammasome cannot be activated by the basal level of NLRP3 (Sharma and Kanneganti, 2021). Based on the available studies, it is found that the activation of NLRP3 inflammasome mainly relies on two different signals (Sharma and Kanneganti, 2021; Toldo et al., 2022; Xia et al., 2023). The first is the “priming” signal (Xia et al., 2023). This signal is mainly involved by a variety of PRRs (toll-like receptors (TLRs) as well as multiple cytokine receptors, including the TNF receptor and the IL-1 receptor), which recognize PAMPs and DAMPs, thereby triggering the nuclear translocation of nuclear factor kappa B (NF-κB), which in turn induces the transcription of NLRP3, IL-1β and IL-18 (Sharma and Kanneganti, 2021; Toldo et al., 2022; Xia et al., 2023). This is immediately followed by the “triggering” signal (Sharma and Kanneganti, 2021; Toldo et al., 2022; Xia et al., 2023). This signal allows for the oligomerization of NLRP3 through the NACHT, and the PYD in NLRP3 is primarily responsible for connecting with the PYD in the ASC. This interaction, in turn, makes it easier for the ASC to bind to pro-caspase-1, ultimately forming the NLRP3 inflammasome (Ludlow et al., 2005; Tattoli et al., 2007; Kumar et al., 2009; Rathinam et al., 2012; Latz et al., 2013; Lu et al., 2014; Motta et al., 2015; Toldo et al., 2015; Yin et al., 2015; Murphy et al., 2016; Yang et al., 2016; Pavillard et al., 2017; Arterbery et al., 2018; Komada et al., 2018; Li et al., 2018; Toldo and Abbate, 2018; Hausmann et al., 2020; Mezzaroma et al., 2021). At the same time, pro-caspase-1 undergoes cleavage, resulting in the release of active caspase-1. This released caspase-1 then activates pro-IL-1β, pro-IL-18 and gasdermin-D (GSDMD) (Toldo et al., 2022). The formation of membrane pores by activated N-terminal GSDMD (NT-GSDMD) leads to the release of IL-1β and IL-18 from the intracellular space, which ultimately leads to the induction of cellular pyroptosis (Shao et al., 2007; Liu et al., 2016; Toldo and Abbate, 2018; Abbate et al., 2020). “Triggering” signal is induced by many factors, such as ion flow (K+ efflux, Na+ influx), adenosine triphosphate (ATP), pore-forming toxins, particulate matter, silica crystals, etc. (Sharma and Kanneganti, 2021). Figure 2 illustrates the activation pathway of the NLRP3 inflammasome.
Figure 2. The activation process of NLRP3 inflammasome. The activation of NLRP3 inflammasome depends on two main signals. First is the “priming” signal. This signal is primarily mediated by a variety of PRRs, including TLRs. These receptors recognize PAMPs or DAMPs, thereby triggering the nuclear translocation of NF-κB. This process subsequently induces the transcription of NLRP3, IL-1β, and IL-18. The next is the “triggering” signal. The signal promotes NLRP3 oligomerization and NLRP3 interacts with ASC. ASC binds to pro-caspase-1 to form the NLRP3 inflammasome. Pro-caspase-1 is cleaved into active caspase-1, which activates pro-IL-1β, pro-IL-18 and GSDMD. Activated NT-GSDMD forms membrane pores, releasing IL-1β and IL-18 and inducing pyroptosis. This signal is triggered by factors like ion flow (K+ efflux, Na+ influx), ATP, pore-forming toxins, particulate matter and silica crystals (Created with BioRender.com). (PRRs, pattern recognition receptors; PAMPs, pathogen-associated molecular patterns; DAMPs, damage-associated molecular patterns; TLRs, toll-like receptors; NF-κB, nuclear factor kappa B; IκK, IkappaB kinase; IL-1β, interleukin-1beta; NLRP3, nod-like receptor family pyrin domain-containing 3; ASC, apoptosis-associated speckle-like protein; GSDMD, gasdermin-D; ATP, adenosine triphosphate; P2X7, purinergic 2X7).
There are numerous reports on phytochemicals ameliorating DOX-induced cardiotoxicity. He et al. has shown that phytochemical could ameliorate DOX-induced cardiotoxicity by modulating cardiomyocyte autophagy (He et al., 2021). Lin et al. demonstrate that phytochemical could ameliorate DOX-induced cardiotoxicity by affecting cardiomyocyte oxidative stress (Lin and Wang, 2023). NLRP3 inflammasome-mediated pyroptosis of cardiomyocytes is a significant pathological mechanism of DOX-induced cardiotoxicity. Therefore, in this section, based on the available literature, we will classify and summarize which phytochemicals can ameliorate DOX-induced cardiotoxicity by inhibiting NLRP3 inflammasome-mediated cardiomyocyte pyroptosis. Table 1 provides a detailed summary of this section.
Table 1. Phytochemicals ameliorate doxorubicin-induced cardiotoxicity by affecting NLRP3 inflammasome-mediated cardiomyocyte pyroptosis.
Polyphenols are organic compounds distinguished by many phenolic units and are extensively found in diverse plants (Salucci et al., 2017; Tian et al., 2021). There are numerous reports that polyphenols can be involved in relieving DOX-induced cardiotoxicity by modulating multiple biological functions (Gu et al., 2015; Yi et al., 2024). Based on the available literature, four polyphenolic phytochemicals could ameliorate DOX-induced cardiotoxicity by inhibiting NLRP3 inflammasome-mediated cardiomyocyte pyroptosis.
Curcumin is a bright yellow polyphenolic compound isolated and extracted from turmeric, which has significant anti-inflammatory and anti-oxidative stress properties (Vollono et al., 2019; Ms et al., 2020; D'Andurain et al., 2023). Available studies suggest its crucial function in mitigating DOX-induced cardiotoxicity. According to a report, curcumin could reduce inflammation and oxidative stress in cardiomyocytes, thereby ameliorating DOX-induced cardiotoxicity (Ibrahim Fouad and Ahmed, 2022). Interestingly, an existing report suggests that curcumin’s amelioration of DOX-induced cardiotoxicity is also strongly associated with its inhibition of NLRP3 inflammasome (Yu et al., 2020). This study has shown that DOX has a number of negative effects on the heart, including a decrease in heart weight, an increase in blood levels of the biomarkers of myocardial injury like creatine kinase (CK), lactate dehydrogenase (LDH), aspartate transaminase (AST) and cardiac troponin I (cTnI) and a noticeable impact on the efficiency and contractility of the cardiac pumping function. Further experiments show that DOX can dramatically raise the production of NLRP3 inflammasome and proteins associated with cell pyroptosis, including caspase-1, IL-1β and IL-18, in the myocardium. This indicates that the cardiac injury induced by DOX is likely associated with the triggering of the NLRP3 inflammasome and its subsequent mediation of cellular pyroptosis in the myocardium. After curcumin intervention, the severe cardiac damage caused by DOX is significantly reversed, and the expression of NLRP3 inflammasome and its downstream-mediated cellular pyroptosis-associated proteins are significantly reduced. This suggests that curcumin’s amelioration of DOX-induced cardiotoxicity may connect to its inhibition of NLRP3 inflammasome and cardiomyocyte pyroptosis. How does curcumin affect DOX-induced expression of NLRP3 inflammasome in cardiomyocytes? Unfortunately, this article does not explore this. However, the effects of curcumin on DOX-induced expression of ROS as well as the phosphatidylinositol 3-kinase (PI3K)/protein kinase B (AKT)/mammalian target of the rapamycin (mTOR) signaling pathway in cardiomyocytes are also examined in this study. Is there a link between curcumin’s altered expression of NLRP3 inflammasome and ROS or PI3K/AKT/mTOR signaling pathway? This will hopefully be confirmed in future studies. Meanwhile, it is noteworthy that there are no clinical reports on the amelioration of DOX-induced cardiotoxicity by curcumin, which may be attributed to its lower oral utilization (Maiti et al., 2007; Chen et al., 2015).
Resveratrol, a polyphenolic compound, is present in various plants, including grapes and peanuts, is known for its substantial anti-oxidant and anti-aging capabilities and is essential in the treatment of numerous cardiovascular illnesses (Petrovski et al., 2011; Zhang et al., 2021). A recent report demonstrates that resveratrol significantly reduces ferroptosis in cardiomyocytes, thereby ameliorating DOX-induced cardiotoxicity (Chen L. et al., 2024). Interestingly, available evidence suggests that resveratrol can also reduce the severity of cardiac damage caused by DOX by suppressing the NLRP3 inflammasome and cardiomyocyte pyroptosis (Maayah et al., 2021). The study shows that DOX significantly increases blood pressure in mice, induces perivascular fibrosis in the myocardium and severely affects the normal structure of the myocardium. This may be due to the activation of NLRP3 inflammasome and the proteins related to cellular pyroptosis, IL-1β and IL-18. The intervention of resveratrol could well reverse the above pathological effects and significantly reduce the DOX-induced activation of NLRP3 inflammasome and downstream cellular pyroptosis-related proteins. This suggests that resveratrol can significantly ameliorate DOX-induced cardiotoxicity, and this effect may be attributed to the inhibition of NLRP3 inflammasome and downstream cellular pyroptosis-related proteins in cardiomyocytes. However, is there some link between NLRP3 inflammasome and ferroptosis regulated by resveratrol in DOX-induced cardiotoxicity? We propose a novel idea, that whether resveratrol can influence ferroptosis by influencing the expression of NLRP3 inflammasome in DOX-induced cardiomyocytes? This question is still unanswered. However, it has been shown that a significant upstream-downstream relationship between NLRP3 inflammasome and ferroptosis has also been reported (Hacioglu, 2024; Yuan et al., 2024; Zhang et al., 2024). Therefore, there is a theoretical basis for speculating that resveratrol can also affect DOX-induced ferroptosis in cardiomyocytes by influencing the expression of NLRP3 inflammasome, and thus ameliorating DOX-induced cardiotoxicity. Meanwhile, there are no clinical studies on resveratrol ameliorating DOX-induced cardiotoxicity, and resveratrol’s solubility may explain why. Resveratrol has been reported to have a low solubility of 0.005 mg/mL in water, and its chemical structure is unstable when exposed to the intestine and stomach, which leads to its inefficient utilization after oral administration (Francioso et al., 2014; Robinson et al., 2015).
Honokiol, a polyphenolic small molecule compound stemming from the bark of Magnolia officinalis, exhibits many effects, including anti-allergic and anti-anxiety, and is crucial in the treatment of cardiovascular disorders (Munroe et al., 2010; Guillermo et al., 2012; Wang et al., 2018; Liu et al., 2023). A recent report shows that honokiol can significantly ameliorate DOX-induced mitochondrial damage, thereby attenuating DOX-induced cardiotoxicity (Pillai et al., 2017). Another study shows honokiol could ameliorate DOX-induced cardiotoxicity by modulating NLRP3 inflammasome-mediated cardiomyocyte cellular pyroptosis (Huang et al., 2020). This study demonstrates that DOX significantly reduces cardiomyocyte viability and promotes senescence-related proteins such as p16INK4A and p21. This suggests that DOX can increase cardiomyocyte mortality by inducing cardiomyocyte senescence. Further experiments demonstrate that DOX significantly induces the expression of thioredoxin-interacting protein (TXNIP) and NLRP3 inflammasome and its downstream-mediated cellular pyroptosis proteins, such as casapse-1 and IL-1β, in cardiomyocytes. This demonstrates that DOX can also lead to cardiomyocyte death by inducing cardiomyocyte pyroptosis. Honokiol’s intervention significantly ameliorates DOX-induced cellular senescence and NLRP3 inflammasome-mediated cellular pyroptosis. Further experiment overexpressing TXNIP shows that the function of honokiol disappears. This demonstrates that the function of honokiol is closely related to TXNIP and that honokiol attenuates DOX-induced cellular senescence and NLRP3 inflammasome-mediated cellular pyroptosis by inhibiting TXNIP. However, it is noteworthy that this study attributes DOX-induced cardiomyocyte senescence to NLRP3 inflammasome-mediated cellular pyroptosis. We think this is inappropriate. Because the study does not modulate NLRP3 inflammasome to detect cardiomyocyte senescence, it is impossible to determine whether NLRP3 inflammasome-mediated cellular pyroptosis is upstream of cellular senescence.
Amentoflavone is a polyphenol compound derived from Selaginella tamariscina (Ma et al., 2024). Available studies have demonstrated that amentoflavone has significant anti-inflammatory and anti-apoptotic properties and is essential in ameliorating several cardiovascular diseases (Qin et al., 2018; Li W. W. et al., 2021). A study has demonstrated that amentoflavone ameliorates DOX-induced cardiotoxicity by attenuating NLRP3 inflammasome-mediated cellular pyroptosis (Fang et al., 2023). This study demonstrates that DOX significantly affects the pumping function and hemodynamics of the heart, as well as significantly inducing cardiac hypertrophy and myocardial fibrosis, with damaging effects on the structure of myocardial tissue. The study also shows that DOX significantly increases blood levels of the markers of myocardial damage, creatine kinase-myoglobin (CK-MB) and LDH. The emergence of these pathologies may be closely associated with increased NLRP3 inflammasome and their downstream-mediated cellular pyroptosis proteins such as caspase-1 and GSDMD, IL-1β and IL-18. This experiment further shows that DOX promotes stimulator of interferon genes (STING) expression. Interestingly, amentoflavone intervention significantly reverses various myocardial pathologic injuries caused by DOX and significantly reduces NLRP3 inflammasome-mediated cellular pyroptosis-related proteins and STING. This suggests that the mechanism of amentoflavone in ameliorating DOX-induced cardiotoxicity may be closely related to its inhibition of NLRP3 inflammasome and its downstream-mediated cardiomyocyte pyroptosis. Further experiments overexpressing STING reveal that the effect of amentoflavone disappears and NLRP3 expression is significantly elevated. This suggests that amentoflavone ameliorates DOX-induced cardiotoxicity by inhibiting STING and, thus, NLRP3 inflammasome, inhibiting cardiomyocyte pyroptosis.
Flavonoids are a category of vital phytochemicals characterized by polyphenolic structures, frequently found in a variety of plants, fruits, and vegetables as secondary metabolites (Martínez-Coria et al., 2023; Yi, 2023). In recent years, reports on flavonoid phytochemicals ameliorating DOX-induced cardiotoxicity have gradually increased. In the following section, we will summarize six different flavonoid phytochemicals ameliorating DOX-induced cardiotoxicity by inhibiting NLRP3 inflammasome-mediated cardiomyocyte pyroptosis.
Myricetin, a flavonoid phytochemical, is frequently present in various natural plants, especially prunes (Song et al., 2021). According to numerous preclinical investigations, myricetin serves multiple biological purposes and is essential in the amelioration of cardiovascular-related diseases (Zhang et al., 2018; Zhu J. et al., 2024). A study has shown that myricetin can ameliorate DOX-induced cardiotoxicity by modulating NLRP3 inflammasome-mediated cardiomyocyte cellular pyroptosis (Li et al., 2024). This study describes in detail that DOX significantly increases the release of myocardial injury markers such as cTnI, AST, brain natriuretic peptide (BNP) and LDH in the blood and increases the levels of cholesterol (TC) and triglycerides (TG) in the blood. Pathological staining experiments prove that DOX could significantly damage the structure of myocardial tissue and induce the development of myocardial fibrosis. Further experiments demonstrate that DOX significantly induces NLRP3 inflammasome-mediated cellular pyroptosis in myocardial tissues (derived from increased NLRP3, ASC and caspase-1). However, the above pathology is significantly reversed, and the expression of NLRP3 inflammasome and their mediated cellular pyroptosis proteins in cardiomyocytes are significantly reduced after the intervention of low, medium and high doses of myricetin. Therefore, this study implies that myricetin’s effect in mitigating DOX-induced cardiotoxicity is likely associated with its inhibition of NLRP3 inflammasome and cardiomyocyte pyroptosis. It is crucial to acknowledge that there are no clinical cases concerning the amelioration of DOX-induced cardiotoxicity by myricetin, and we believe that based on the broad pharmacological effects of myricetin, future studies should gradually validate their association in clinical studies.
Pinocembrin is a flavonoid phytochemical found mainly in Euphorbia, Sparattosperma leucanthum and Pinus heartwood (Rasul et al., 2013). Its widespread pharmacological activity in the anti-cardiovascular disease has been well studied (Li C. et al., 2021). In a study of DOX-induced cardiotoxicity, Gu et al. (2021) find that DOX could significantly affect the pumping function of the heart and can lead to the development of fibrosis and myocardial tissue damage. The study also shows that DOX can increase the release of CK-MB and LDH, markers of myocardial damage, in the blood. Further experiments demonstrate that DOX could inhibit the nuclear translocation of nuclear factor erythroid-2-related factor 2 (Nrf2) and activate the downstream sirtuin 3 (SIRT3)/NLPR3 signaling pathway, which in turn can induce the expression of cellular pyroptosis proteins, such as caspase-1 and GSDMD-N, and ultimately lead to myocardial pyroptosis. In contrast, pinocembrin significantly reverses DOX-inhibited Nrf2 nuclear translocation, thereby inhibiting the SIRT3/NLPR3 signaling pathway and its downstream-mediated cellular pyroptosis proteins, and thus ameliorating DOX-induced cardiotoxicity. Nevertheless, it is pertinent to acknowledge that the relationship between SIRT3 and NLRP3 inflammasome is not demonstrated in this study, and we are aware that many studies have demonstrated that SIRT3 is located upstream of NLRP3 inflammasome. However, it is illogical to conclude the above results directly in this study because SIRT3 is not modulated to detect the expression level of NLRP3 inflammasome.
Dihydromyricetin is a flavonoid phytochemical widely found in Ampelopsis grossedentata (Wang et al., 2023). One recent study suggests it serves a crucial function in attenuating DOX-induced cardiotoxicity (Sun et al., 2020). As this research demonstrates, DOX can significantly induce myocardial damage, myocardial fibrosis and pathological cardiac hypertrophy. In the meanwhile, the pumping function of the heart is also significantly affected. Additional experimental evidence suggests that myocardial injury may be associated with the induction of NLRP3 inflammasome and cellular pyroptosis. The intervention of dihydromyricetin significantly reverses the above pathology and inhibits the induction of NLRP3 inflammasome and cellular pyroptosis. Interestingly, the study further explores how dihydromyricetin can affect the expression of NLRP3 inflammasome in cardiomyocytes. This study finds that DOX can significantly inhibit the expression of SIRT1, while the expression of SIRT1 is significantly elevated after dihydromyricetin intervention, and the effect of dihydromyricetin is found to disappear after silencing SIRT1. This demonstrates that dihydromyricetin can inhibit the induction of NLRP3 inflammasome by activating the expression of SIRT1 in cardiomyocytes, thereby inhibiting cardiomyocyte pyroptosis and ultimately attenuating DOX-induced cardiotoxicity. Notably, it has been found that dihydromyricetin has a low oral utilization and short half-life, which significantly affects its use in the clinic (Wang et al., 2023).
Hyperoside is a flavonoid phytochemical found in various plants such as Hypericum monogynum (Hypericaceae), Crataegus pinnatifida (Rosaceae) and Polygonum aviculare (Polygonaceae) (Xu et al., 2022). One available evidence has demonstrated its crucial role in interfering with DOX-induced cardiotoxicity (Wei et al., 2023). This study suggests that DOX significantly promotes cardiac hypertrophy in mice and facilitates the release of myocardial injury biomarkers, such as cardiac troponin T (cTnT), CK, CK-MB and LDH, in the blood, as well as affecting the pumping function of the heart. Subsequent investigations indicate that the cause of myocardial injury may be closely related to the activation of NLRP3 inflammasome and its downstream-mediated cellular pyroptosis. Intervention with hyperoside significantly reverses these pathologies and simultaneously reduces the expression of NLRP3 inflammasome and its downstream-mediated cellular pyroptosis in cardiomyocytes. Therefore, this study concludes that the mechanism of hyperoside intervention in DOX-induced cardiotoxicity may be intricately linked to its suppression of NLRP3 inflammasome and its downstream-mediated cellular pyroptosis. It is worth noting that in this study, hyperoside is administered to mice by gavage. Yuan et al. have examined the impact of hyperoside of different modes of administration on rats, and they have found that the bioavailability of hyperoside in rats is significantly low following gavage administration and that intraperitoneal injection (IP) and intravenous administration (IV) are the best ways to promote the absorption and utilization of hyperoside in the body (Yuan et al., 2021).
Calycosin, a flavonoid phytochemical, is derived mainly from the root of Astragalus membranaceus and a typical phytoestrogen (Han et al., 2018; Deng et al., 2021). Current studies have shown that calycosin has multiple biological functions and is essential in ameliorating several cardiovascular diseases (Pan et al., 2020). A recent study shows that calycosin could ameliorate DOX-induced cardiotoxicity through the inhibition of NLRP3 inflammasome-mediated cardiomyocyte pyroptosis (Zhang et al., 2022). This study shows that DOX significantly damages the pumping function of the heart and induces cardiac fibrosis. Further experiments demonstrate that DOX increases NLRP3 inflammasome and their downstream-mediated cardiomyocyte pyroptosis. Intervention with calycosin reverses these pathologies and reduces NLRP3 inflammasome-mediated pyroptosis in cardiomyocytes. Therefore, this study concludes that the amelioration of DOX-induced cardiotoxicity by calycosin may be closely related to the inhibition of NLRP3 inflammasome in cardiomyocytes. Another study further explores the association between calycosin and NLRP3 inflammasome in cardiomyocytes (Zhai et al., 2020). They demonstrate that the SIRT1 inhibitor significantly promotes the expression of NLRP3 inflammasome in cardiomyocytes, whereas calycosin significantly promotes SIRT1 expression in cardiomyocytes. Therefore, the study suggests that calycosin may inhibit the expression of NLRP3 inflammasome in cardiomyocytes by promoting the expression of SIRT1, thereby inhibiting cardiomyocyte pyroptosis and ameliorating DOX-induced cardiotoxicity. Unfortunately, there are fewer pharmacokinetic studies on calycosin, and most of the current studies are on the co-metabolism of calycosin in combination with other drugs, so the metabolic process for calycosin can only be derived by speculating on its composition (Deng et al., 2021).
Cynaroside is a flavonoid phytochemical distributed in the honeysuckle plant, and it has potential biological properties such as anti-inflammatory, anti-oxidant and anti-pyroptosis (Zou et al., 2024). A study demonstrates that cynaroside could inhibit DOX-induced cardiomyocyte death by affecting the expression of the NLRP3 inflammasome (Zou et al., 2024). This study shows that DOX can significantly induce structural damage in mouse myocardial tissue and promote the release of myocardial damage markers alkaline phosphatase (ALP), AST, LDH and CK-MB in the blood. Further experiments demonstrate that the myocardial damage caused by DOX is partly due to the activation of NLRP3 inflammasome in cardiomyocytes and its downstream-mediated cardiomyocyte pyroptosis. The intervention of cynaroside significantly reverses the above pathologic effects, and this reversal may result from the inhibition of NLRP3 inflammasome and their downstream-mediated pyroptosis in cardiomyocytes.
Terpenoids are the biggest class of compounds in natural products, predominantly derived from plants (Huang et al., 2012). The biological functions of terpenoids are well documented. Here, we will summarize four different terpenoids that ameliorate DOX-induced cardiomyocyte pyroptosis, thus improving myocardial injury by affecting the expression of NLRP3 inflammasome in cardiomyocytes.
Carnosic acid, a phenolic diterpenoid, is found in Lamiaceae plants, including Rosemary (Rosmarinus officinalis L.) (Chen X. et al., 2024). Available evidence suggests its crucial role in intervening in cardiovascular-related diseases (Sahu et al., 2014; Zhang et al., 2019). One study demonstrates that carnosic acid could mitigate DOX-induced cardiomyocyte death by suppressing NLRP3 inflammasome in cardiomyocytes (Hu et al., 2023). The study shows that DOX significantly induces cardiac pumping dysfunction, disrupts normal myocardial structure and induces myocardial fibrosis in mice. It also promotes the release of biomarkers that indicate damage to the myocardium, such as CK-MB, LDH, and cTnT, in the blood. Subsequent research has shown that DOX-induced cardiotoxicity may be intricately linked to the activation of the NLRP3 inflammasome and its facilitation of cardiomyocyte pyroptosis proteins, including caspase-1, IL-1β, and IL-18. This study also shows that DOX significantly inhibits the expression of Nrf2 and its downstream anti-oxidative stress-related proteins, heme oxygenase-1 (HO-1). Interestingly, intervention with carnosic acid significantly reverses the above pathology, in which the suppression of NLRP3 inflammasome and cellular pyroptosis may have an indispensable role. To explore how carnosic acid could suppress NLRP3 inflammasome, the study silences Nrf2 and finds that the therapeutic effect of carnosic acid disappears after silencing Nrf2. This suggests that carnosic acid ameliorates DOX-induced cardiotoxicity by activating Nrf2 expression in cardiomyocytes, thereby inhibiting the expression of NLRP3 inflammasome and their downstream cellular pyroptosis. It is crucial to recognize that although many natural compounds possess significant pharmacological capabilities, they may also elicit severe side effects or hazardous responses under certain circumstances, with carnosic acid serving as a pertinent example. Several evidences have been targeted to explore the toxic effects of carnosic acid and have demonstrated the presence of acute, chronic, hepatic and renal toxicity of carnosic acid (Dickmann et al., 2012; Wang et al., 2012; Liu et al., 2017). Therefore, the cytotoxicity of carnosic acid must be taken into account when using.
As a monocyclic sesquiterpene alcohol, α-Bisabolol is the primary source of Matricaria chamomilla, and available evidence has suggested that it is present in large quantities of medicinal plants’ essential oils (McKay and Blumberg, 2006; Eddin et al., 2022). The biological functions of α-Bisabolol have been validated, and many studies have demonstrated its ameliorative and palliative effects in various cardiovascular diseases (Meeran et al., 2018; Nagoor Meeran et al., 2019). One study has demonstrated that α-Bisabolol can ameliorate DOX-induced cardiomyocyte death by modulating the expression of NLRP3 inflammasome in cardiomyocytes (Nagoor Meeran et al., 2023). This study shows that DOX significantly increases the blood levels of myocardial injury markers such as CK and LDH and significantly affects the cardiac hemodynamics in rats. Further experiments demonstrate that DOX significantly increases the expression of NLRP3 inflammasome and its downstream-mediated cell pyroptosis-associated proteins, such as caspase-1 and IL-18, in cardiomyocytes. This suggests that the cause of the above myocardial injury by DOX may be closely related to the activation of NLRP3 inflammasome in the myocardium. Intervention of α-Bisabolol can significantly reverse the above effects. This suggests that the mechanism of α-Bisabolol in ameliorating DOX-induced cardiotoxicity is partly related to the inhibition of NLRP3 inflammasome-mediated cellular pyroptosis. Like carnosic acid, α-Bisabolol has toxic effects. Toxic reactions such as sedation, ataxia, dyspnea and affective blunting have been demonstrated after oral α-Bisabolol overdose (Api et al., 2020; Eddin et al., 2022). Therefore, the intervention dosage of α-Bisabolol is an issue that must be focused on in future clinical studies.
Andrographolide, a labdane diterpene, is mostly sourced from Andrographis paniculata, exhibiting a diverse array of pharmacological effects (Burgos et al., 2020). One available evidence suggests that andrographolide can mitigate DOX-induced cardiotoxicity (Liu et al., 2024). The study claims that DOX induces cardiomyocyte death and promotes the expression of markers of myocardial damage, CK-MB and LDH, in the blood of mice, as well as affecting the pumping function of the heart. Further experiments demonstrate that myocardial injury caused by DOX is closely related to the activation of NLRP3 inflammasome in cardiomyocytes. Andrographolide significantly reverses these pathologies, inhibiting NLRP3 inflammasome and their downstream-mediated cellular pyroptosis in cardiomyocytes. This, therefore, suggests that the mechanism of andrographolide in ameliorating DOX-induced cardiotoxicity has a profound connection with the suppression of NLRP3 inflammasome and their downstream-mediated cellular pyroptosis in cardiomyocytes.
Nerolidol, a sesquiterpene alcohol, is naturally present in the essential oil of a variety of plants (Lapczynski et al., 2008; Ferreira et al., 2012; Chan et al., 2016). One available evidence suggests that nerolidol has an effect that interferes with DOX-induced cardiotoxicity (Meeran et al., 2021). This study demonstrates that DOX could induce the release of myocardial damage markers CK, LDH and Troponin-T/I in the blood while inducing cardiac fibrosis in mice. Further experiments show that these pathologic effects are closely linked to the activation of NLRP3 inflammasome and their mediated cellular pyroptosis. This study demonstrates that nerolidol can effectively reverse the pathology caused by DOX, and this effect may be intimately connected to its capacity to diminish the expression of the NLRP3 inflammasome and cellular pyroptosis in cardiomyocytes. It is encouraging that the toxic effects of nerolidol are low and that none of the doses used are sufficient to cause severe toxic effects (Chan et al., 2016). Therefore, many translational clinical studies should be on the agenda based on the doses used.
Polysaccharides are an important class of biomolecules, consisting of more than 10 monosaccharide molecules condensed by dehydration, and are widely distributed in nature (Zhou and Huang, 2021). Polysaccharides have extensive array of biological characteristics, such as anti-oxidant, anti-viral and anti-inflammatory, and available evidence also suggests that they also play a critical role in ameliorating DOX-induced cardiotoxicity (Chen F. and Huang G., 2018; Chen L. and Huang G., 2018; Liu et al., 2018; Wang et al., 2022). Here, we will summarize two different polysaccharides based on the existing studies that affect the NLRP3 inflammasome, thereby ameliorating DOX-induced cardiomyocyte pyroptosis and consequently ameliorating myocardial injury.
Polyguluronic acid, a polysaccharide from alginate, has several biological effects, such as anti-inflammatory and anti-oxidants (Dun et al., 2015; Bakhtiari et al., 2019). The evidence suggests its crucial role in intervening in DOX-induced cardiotoxicity (Zhang et al., 2023). This study shows that DOX can induce cardiomyocyte death, significantly affect the normal structure of myocardial tissue in mice and promote the release of LDH, a marker of myocardial injury, in the blood. This phenomenon may be closely related to the activation of NLRP3 inflammasome and its downstream-mediated casapse-1 and GSDMD proteins. Polyguluronic acid could reverse the above pathologic effects and significantly reduce the expression of NLRP3 inflammasome and cardiomyocyte pyroptosis. To investigate how polyguluronic acid reverses the expression of NLRP3 inflammasome, the study finds that DOX significantly activates the expression of Pellino1 (Peli1), while polyguluronic acid significantly inhibits its expression. However, the pathology of cardiomyocytes is found to be significantly reduced after Peli1 inhibition. This suggests that polyguluronic acid may ameliorate cardiomyocyte injury by inhibiting the expression of Peli1, which in turn inhibits the expression of NLPR3 inflammasome, which in turn inhibits cardiomyocyte pyroptosis.
Fuzi polysaccharide is a water-soluble polysaccharide compound derived from Fuzi and has a variety of biological effects (Yan et al., 2010). Available studies have shown its significant contribution to the treatment of cardiovascular diseases (Liao et al., 2013). A report of DOX-induced cardiotoxicity has demonstrated that fuzi polysaccharide could ameliorate DOX-induced cardiomyocyte death by modulating the expression of NLRP3 inflammasome in cardiomyocytes (Xiong et al., 2024). This study shows that DOX can significantly reduce cardiac contractile function and induce the expression of myocardial damage biomarkers, including CK-MB and LDH, in the blood of mice. The study also implies that the activation of the NLRP3 inflammasome in cardiomyocytes may be strongly connected with the cause of myocardial injury and intervention with fuzi polysaccharide significantly reverses the above pathologic effects and reduces the expression of NLRP3 inflammasome and their mediated pyroptosis in the myocardium. Interestingly, the study further reveals that DOX can significantly stimulate the expression of IL-6 while suppressing the expression of signal transducer and activator of transcription 3 (STAT3). IL-6/STAT3 signaling pathway-mediated apoptosis has been demonstrated (Zhou et al., 2021). Therefore, this study suggests that the cause of myocardial injury by DOX may be through activation of NLRP3 inflammasome and, thus, activation of cardiomyocyte pyroptosis, which, in turn, activates the IL-6/STAT3 signaling pathway and thus causes apoptosis. Fuzi polysaccharide ameliorates DOX-induced cardiotoxicity by blocking the above pathway. However, we think that this study does not detect the association between cellular pyroptosis and the IL-6/STAT3 signaling pathway, and it is inappropriate to connect the two directly. Subsequent experiments should detect the expression of the IL-6/STAT3 signaling pathway after cellular pyroptosis has been regulated and then make a corresponding judgment.
Saponins, amphiphilic compounds, are composed of carbohydrates and either triterpenoid or steroid aglycone moieties, frequently discovered in herbal and conventional medicines (Juang and Liang, 2020). The biological functions of saponins have been well studied, and saponins have been found to have a very important potential in intervening in cardiovascular-related diseases (Liu et al., 2014; Sun et al., 2016; Yao et al., 2022). Astragaloside IV, a saponin extracted from A. membranaceus Bunge, is rich in biological activities (Zhou et al., 2023). Two available evidences suggest that astragaloside IV carries a significant amount of weight in attenuating DOX-induced cardiotoxicity (Chen et al., 2023; Tian et al., 2024). One study shows that DOX can significantly induce pump dysfunction and promote fibrosis of myocardial tissue in mice. It also could induce the release of biomarkers that indicate injury to the myocardium, such as CK-MB, LDH and cTnI in the blood. Further experiments show that DOX can also induce the expression of NLRP3 inflammasome and cardiomyocyte pyroptosis. Intervention with astragaloside IV significantly reverses these pathologies and significantly reduces the expression of NLRP3 inflammasome and their mediated cell pyroptosis-associated proteins in cardiomyocytes. Notably, the study further explores the association between astragaloside IV and NLRP3 inflammasome in cardiomyocytes and finds that DOX can significantly inhibit the expression of Nrf2 and its downstream anti-oxidant-associated protein Ho-1, which is significantly reversed by astragaloside IV intervention. Therefore, this study concludes that astragaloside IV could inhibit ROS production in cardiomyocytes by activating Nrf2, thereby attenuating the expression of NLRP3 inflammasome and preventing cardiomyocyte pyroptosis. However, this study does not experimentally validate the relationship between Nrf2 and NLRP3, which is a shortcoming. Of course, another report on astragaloside IV further explores how astragaloside IV inhibits the expression of NLRP3 inflammasome in cardiomyocytes (Tian et al., 2024). This study shows that DOX can significantly inhibit SIRT1 expression, which is significantly elevated after astragaloside IV intervention. However, the pharmacologic effect of astragaloside IV is found to be lost after SIRT1 is inhibited. This suggests that astragaloside IV works by activating SIRT1, which in turn inhibits NLRP3 inflammasome, thus inhibiting cardiomyocyte pyroptosis. Interestingly, the study also examines the expression of AMP-activated protein kinase (AMPK) and AKT in cardiomyocytes and demonstrates that both AMPK and AKT are located downstream of SIRT1 and that astragaloside IV inhibits cardiomyocyte pyroptosis by activating SIRT1, which in turn activates either AMPK or AKT, which in turn inhibits the expression of the NLRP3 inflammasome in cardiomyocytes, and thereby inhibits cardiomyocyte pyroptosis, which in turn ameliorate DOX-induced cardiotoxicity. However, since neither AMPK nor AKT are modulated in this study to assess their effect on SIRT1 or NLRP3, this conclusion may be premature.
In addition to polyphenols, flavonoids, terpenoids, polysaccharides and saponins phytochemicals, several small molecule compounds do not fall into the above categories that can also ameliorate DOX-induced cardiotoxicity by affecting the NLRP3 inflammasome in cardiomyocytes. We will elaborate here. First, fraxetin. Fraxetin is a coumarin phytochemical with a variety of biological functions (Robe et al., 2021). In a study of DOX-induced cardiotoxicity, fraxetin is reported to ameliorate DOX-induced cardiomyocyte pyroptosis by affecting the expression of NLRP3 inflammasome in cardiomyocytes (Kabel et al., 2021). The study reports that DOX significantly increases the levels of myocardial injury markers such as CK-MB, LDH, cTnI and NT-pro BNP in the blood in rats, while significantly affecting the pumping function of the heart and inducing myocardial fibrosis. Further experiments demonstrate that DOX significantly activates the expression of NLRP3 inflammasome in cardiomyocytes. The above pathological effects are significantly reversed by high and low doses of fraxetin, which also reduces the expression of NLRP3 inflammasome in cardiomyocytes. This suggests that the impact of fraxetin in mitigating DOX-induced cardiotoxicity may be closely related to its inhibition of NLRP3 inflammasome-mediated cell pyroptosis. Another report is about betaine. There is a report that betaine, as a type of alkaloid, can also ameliorate DOX-induced cardiomyocyte pyroptosis by affecting NLRP3 inflammasome in cardiomyocytes (Mohammadpour et al., 2024). This study shows that DOX significantly induces the release of markers of myocardial injury, such as CK-MB, LDH and Troponin I, in the blood while affecting the structure of myocardial tissue. Further experiments show that these pathological effects are intimately connected to the activation of the NLRP3 inflammasome in cardiomyocytes, which are significantly reversed by the intervention of betaine, which is intricately associated with the suppression of the NLRP3 inflammasome in cardiomyocytes.
This review focuses on NLRP3 inflammasome-mediated pyroptosis in myocardial cells and details the amelioration of DOX-induced cardiotoxicity by various phytochemicals. This work comprehensively delineates the several pathological pathways behind DOX-induced cardiotoxicity, including oxidative stress, programmed cell death, inflammation and endoplasmic reticulum stress, as informed by recent studies. Subsequently, we provide a comprehensive overview of the NLRP3 inflammasome. Finally, based on the existing findings, we summarize in detail that a variety of phytochemicals ameliorate DOX-induced cardiotoxicity by affecting NLRP3 inflammasome-mediated cardiomyocyte pyroptosis.
However, combined with existing research, they have several shortcomings. Primarily, the majority of current research on phytochemicals to mitigate DOX-induced cardiotoxicity has been confined to preclinical investigations and has not progressed to clinical trials. However, combined with our research in the field, the necessary clinical translation is worth pursuing. Second, during phytochemical interventions, different doses may play different roles. In many of the studies, only an experimental dose is set up, which will seriously undermine an in-depth exploration of the biological functions of the phytochemical. Third, according to the existing reports, we find that some studies claim that phytochemicals such as curcumin and resveratrol belong to pan-assay interference compounds (PAINS). In other words, these phytochemicals can interfere with various reactions (not based on a specific interaction between compound molecules and proteins) in the experiment to achieve a “positive” result. These phytochemicals can seriously affect the output of the experimental results. Therefore, experiments with these phytochemicals should be repeated several times to prevent “false-positive” results.
Regarding the above, we call for the collaboration of additional pertinent researchers to implement systematic experimental design and analysis, as well as to develop an effective evaluation system, thereby enhancing the utilization of phytochemicals for the benefit of patients afflicted by DOX-induced cardiotoxicity.
X-PZ: Writing – original draft. LD: Visualization, Writing – review and editing. Q-RZ: Visualization, Writing – review and editing. XL: Investigation, Writing – review and editing. N-YT: Investigation, Writing – review and editing. S-LY: Conceptualization, Supervision, Writing – review and editing. KD: Conceptualization, Supervision, Writing – review and editing.
The author(s) declare that financial support was received for the research and/or publication of this article. This work was supported by Ministry of Education Humanities and Social Sciences Research Program - Planning Fund Project (Grant numbers 24YJA890007) and Ministry of Education Humanities and Social Sciences Research Program - General Program (Grant numbers 23YJC890010).
Figures were created with Servier Medical Art (smart.servier.com).
The authors declare that the research was conducted in the absence of any commercial or financial relationships that could be construed as a potential conflict of interest.
The author(s) declare that no Generative AI was used in the creation of this manuscript.
All claims expressed in this article are solely those of the authors and do not necessarily represent those of their affiliated organizations, or those of the publisher, the editors and the reviewers. Any product that may be evaluated in this article, or claim that may be made by its manufacturer, is not guaranteed or endorsed by the publisher.
Abbate, A., Toldo, S., Marchetti, C., Kron, J., Van Tassell, B. W., and Dinarello, C. A. (2020). Interleukin-1 and the inflammasome as therapeutic targets in cardiovascular disease. Circ. Res. 126 (9), 1260–1280. doi:10.1161/circresaha.120.315937
Abushouk, A. I., Ismail, A., Salem, A. M. A., Afifi, A. M., and Abdel-Daim, M. M. (2017). Cardioprotective mechanisms of phytochemicals against doxorubicin-induced cardiotoxicity. Biomed. Pharmacother. 90, 935–946. doi:10.1016/j.biopha.2017.04.033
Agudelo, D., Bourassa, P., Bérubé, G., and Tajmir-Riahi, H. A. (2014). Intercalation of antitumor drug doxorubicin and its analogue by DNA duplex: structural features and biological implications. Int. J. Biol. Macromol. 66, 144–150. doi:10.1016/j.ijbiomac.2014.02.028
Ai, Y., Yan, B., and Wang, X. (2021). The oxidoreductases POR and CYB5R1 catalyze lipid peroxidation to execute ferroptosis. Mol. Cell Oncol. 8 (2), 1881393. doi:10.1080/23723556.2021.1881393
Andreeva, L., David, L., Rawson, S., Shen, C., Pasricha, T., Pelegrin, P., et al. (2021). NLRP3 cages revealed by full-length mouse NLRP3 structure control pathway activation. Cell 184 (26), 6299–6312.e22. doi:10.1016/j.cell.2021.11.011
Api, A. M., Belsito, D., Biserta, S., Botelho, D., Bruze, M., Burton, G. A., et al. (2020). RIFM fragrance ingredient safety assessment, α-bisabolol, CAS registry number 515-69-5. Food Chem. Toxicol. 141 (Suppl. 1), 111238. doi:10.1016/j.fct.2020.111238
Arterbery, A. S., Yao, J., Ling, A., Avitzur, Y., Martinez, M., Lobritto, S., et al. (2018). Inflammasome priming mediated via toll-like receptors 2 and 4, induces Th1-like regulatory T cells in de novo autoimmune hepatitis. Front. Immunol. 9, 1612. doi:10.3389/fimmu.2018.01612
Bakhtiari, T., Azarian, S., Ghaderi, A., Ahmadzadeh, A., and Mirshafiey, A. (2019). Effect of guluronic acid (G2013), as a new anti-inflammatory drug on gene expression of pro-inflammatory and anti-inflammatory cytokines and their transcription factors in rheumatoid arthritis patients. Iran. J. Allergy Asthma Immunol. 18 (6), 639–648. doi:10.18502/ijaai.v18i6.2176
Burgos, R. A., Alarcón, P., Quiroga, J., Manosalva, C., and Hancke, J. (2020). Andrographolide, an anti-inflammatory multitarget drug: all roads lead to cellular metabolism. Molecules 26 (1), 5. doi:10.3390/molecules26010005
Carvalho, C., Santos, R. X., Cardoso, S., Correia, S., Oliveira, P. J., Santos, M. S., et al. (2009). Doxorubicin: the good, the bad and the ugly effect. Curr. Med. Chem. 16 (25), 3267–3285. doi:10.2174/092986709788803312
Chan, W. K., Tan, L. T., Chan, K. G., Lee, L. H., and Goh, B. H. (2016). Nerolidol: a sesquiterpene alcohol with multi-faceted pharmacological and biological activities. Molecules 21 (5), 529. doi:10.3390/molecules21050529
Chen, F., and Huang, G. (2018a). Preparation and immunological activity of polysaccharides and their derivatives. Int. J. Biol. Macromol. 112, 211–216. doi:10.1016/j.ijbiomac.2018.01.169
Chen, F. P., Li, B. S., and Tang, C. H. (2015). Nanocomplexation of soy protein isolate with curcumin: influence of ultrasonic treatment. Food Res. Int. 75, 157–165. doi:10.1016/j.foodres.2015.06.009
Chen, L., and Huang, G. (2018b). The antiviral activity of polysaccharides and their derivatives. Int. J. Biol. Macromol. 115, 77–82. doi:10.1016/j.ijbiomac.2018.04.056
Chen, L., Sun, X., Wang, Z., Chen, M., He, Y., Zhang, H., et al. (2024a). Resveratrol protects against doxorubicin-induced cardiotoxicity by attenuating ferroptosis through modulating the MAPK signaling pathway. Toxicol. Appl. Pharmacol. 482, 116794. doi:10.1016/j.taap.2023.116794
Chen, R. C., Sun, G. B., Ye, J. X., Wang, J., Zhang, M. D., and Sun, X. B. (2017). Salvianolic acid B attenuates doxorubicin-induced ER stress by inhibiting TRPC3 and TRPC6 mediated Ca(2+) overload in rat cardiomyocytes. Toxicol. Lett. 276, 21–30. doi:10.1016/j.toxlet.2017.04.010
Chen, X., Tian, C., Zhang, Z., Qin, Y., Meng, R., Dai, X., et al. (2023). Astragaloside IV inhibits NLRP3 inflammasome-mediated pyroptosis via activation of nrf-2/HO-1 signaling pathway and protects against doxorubicin-induced cardiac dysfunction. Front. Biosci. (Landmark Ed) 28 (3), 45. doi:10.31083/j.fbl2803045
Chen, X., Wei, C., Zhao, J., Zhou, D., Wang, Y., Zhang, S., et al. (2024b). Carnosic acid: an effective phenolic diterpenoid for prevention and management of cancers via targeting multiple signaling pathways. Pharmacol. Res. 206, 107288. doi:10.1016/j.phrs.2024.107288
Christidi, E., and Brunham, L. R. (2021). Regulated cell death pathways in doxorubicin-induced cardiotoxicity. Cell Death Dis. 12 (4), 339. doi:10.1038/s41419-021-03614-x
Curigliano, G., Cardinale, D., Dent, S., Criscitiello, C., Aseyev, O., Lenihan, D., et al. (2016). Cardiotoxicity of anticancer treatments: epidemiology, detection, and management. CA Cancer J. Clin. 66 (4), 309–325. doi:10.3322/caac.21341
D'Andurain, J., López, V., Arazo-Rusindo, M., Tiscornia, C., Aicardi, V., Simón, L., et al. (2023). Effect of curcumin consumption on inflammation and oxidative stress in patients on hemodialysis: a literature review. Nutrients 15 (10), 2239. doi:10.3390/nu15102239
Dantas, D., Pereira, A. G., Fujimori, A. S. S., Ribeiro, A. P. D., de Almeida Silva, C. C. V., Monte, M. G., et al. (2022). Doxycycline attenuates doxorubicin-induced cardiotoxicity by improving myocardial energy metabolism in rats. J. Cardiovasc Dev. Dis. 9 (8), 254. doi:10.3390/jcdd9080254
Deng, M., Chen, H., Long, J., Song, J., Xie, L., and Li, X. (2021). Calycosin: a review of its pharmacological effects and application prospects. Expert Rev. Anti Infect. Ther. 19 (7), 911–925. doi:10.1080/14787210.2021.1863145
Dickmann, L. J., VandenBrink, B. M., and Lin, Y. S. (2012). In vitro hepatotoxicity and cytochrome P450 induction and inhibition characteristics of carnosic acid, a dietary supplement with antiadipogenic properties. Drug Metab. Dispos. 40 (7), 1263–1267. doi:10.1124/dmd.112.044909
Ditano-Vázquez, P., Torres-Peña, J. D., Galeano-Valle, F., Pérez-Caballero, A. I., Demelo-Rodríguez, P., Lopez-Miranda, J., et al. (2019). The fluid aspect of the mediterranean diet in the prevention and management of cardiovascular disease and diabetes: the role of polyphenol content in moderate consumption of wine and olive oil. Nutrients 11 (11), 2833. doi:10.3390/nu11112833
Dun, Y. L., Zhou, X. L., Guan, H. S., Yu, G. L., Li, C. X., Hu, T., et al. (2015). Low molecular weight guluronate prevents TNF-α-induced oxidative damage and mitochondrial dysfunction in C2C12 skeletal muscle cells. Food Funct. 6 (9), 3056–3064. doi:10.1039/c5fo00533g
Eddin, L. B., Jha, N. K., Goyal, S. N., Agrawal, Y. O., Subramanya, S. B., Bastaki, S. M. A., et al. (2022). Health benefits, pharmacological effects, molecular mechanisms, and therapeutic potential of α-bisabolol. Nutrients 14 (7), 1370. doi:10.3390/nu14071370
El-Agamy, D. S., El-Harbi, K. M., Khoshhal, S., Ahmed, N., Elkablawy, M. A., Shaaban, A. A., et al. (2019). Pristimerin protects against doxorubicin-induced cardiotoxicity and fibrosis through modulation of Nrf2 and MAPK/NF-kB signaling pathways. Cancer Manag. Res. 11, 47–61. doi:10.2147/cmar.S186696
Fang, G., Li, X., Yang, F., Huang, T., Qiu, C., Peng, K., et al. (2023). Amentoflavone mitigates doxorubicin-induced cardiotoxicity by suppressing cardiomyocyte pyroptosis and inflammation through inhibition of the STING/NLRP3 signalling pathway. Phytomedicine 117, 154922. doi:10.1016/j.phymed.2023.154922
Ferreira, F. M., Palmeira, C. M., Oliveira, M. M., Santos, D., Simões, A. M., Rocha, S. M., et al. (2012). Nerolidol effects on mitochondrial and cellular energetics. Toxicol Vitro 26 (2), 189–196. doi:10.1016/j.tiv.2011.11.009
Font-Belmonte, E., González-Rodríguez, P., and Fernández-López, A. (2020). Necroptosis in global cerebral ischemia: a role for endoplasmic reticulum stress. Neural Regen. Res. 15 (3), 455–456. doi:10.4103/1673-5374.266054
Francioso, A., Mastromarino, P., Masci, A., d'Erme, M., and Mosca, L. (2014). Chemistry, stability and bioavailability of resveratrol. Med. Chem. 10 (3), 237–245. doi:10.2174/15734064113096660053
Gianni, L., Herman, E. H., Lipshultz, S. E., Minotti, G., Sarvazyan, N., and Sawyer, D. B. (2008). Anthracycline cardiotoxicity: from bench to bedside. J. Clin. Oncol. 26 (22), 3777–3784. doi:10.1200/jco.2007.14.9401
Gu, J., Hu, W., and Zhang, D. D. (2015). Resveratrol, a polyphenol phytoalexin, protects against doxorubicin-induced cardiotoxicity. J. Cell Mol. Med. 19 (10), 2324–2328. doi:10.1111/jcmm.12633
Gu, J., Huang, H., Liu, C., Jiang, B., Li, M., Liu, L., et al. (2021). Pinocembrin inhibited cardiomyocyte pyroptosis against doxorubicin-induced cardiac dysfunction via regulating Nrf2/Sirt3 signaling pathway. Int. Immunopharmacol. 95, 107533. doi:10.1016/j.intimp.2021.107533
Guillermo, R. F., Chilampalli, C., Zhang, X., Zeman, D., Fahmy, H., and Dwivedi, C. (2012). Time and dose-response effects of honokiol on UVB-induced skin cancer development. Drug Discov. Ther. 6 (3), 140–146. doi:10.5582/ddt.2012.v6.3.140
Gupta, S. K., Garg, A., Avramopoulos, P., Engelhardt, S., Streckfuss-Bömeke, K., Batkai, S., et al. (2019). miR-212/132 cluster modulation prevents doxorubicin-mediated atrophy and cardiotoxicity. Mol. Ther. 27 (1), 17–28. doi:10.1016/j.ymthe.2018.11.004
Hacioglu, C. (2024). Long-term exposure of sucralose induces neuroinflammation and ferroptosis in human microglia cells via SIRT1/NLRP3/IL-1β/GPx4 signaling pathways. Food Sci. Nutr. 12 (11), 9094–9107. doi:10.1002/fsn3.4488
Han, F., Li, K., Pan, R., Xu, W., Han, X., Hou, N., et al. (2018). Calycosin directly improves perivascular adipose tissue dysfunction by upregulating the adiponectin/AMPK/eNOS pathway in obese mice. Food Funct. 9 (4), 2409–2415. doi:10.1039/c8fo00328a
Harding, D., Chong, M. H. A., Lahoti, N., Bigogno, C. M., Prema, R., Mohiddin, S. A., et al. (2023). Dilated cardiomyopathy and chronic cardiac inflammation: pathogenesis, diagnosis and therapy. J. Intern Med. 293 (1), 23–47. doi:10.1111/joim.13556
Hausmann, A., Böck, D., Geiser, P., Berthold, D. L., Fattinger, S. A., Furter, M., et al. (2020). Intestinal epithelial NAIP/NLRC4 restricts systemic dissemination of the adapted pathogen Salmonella Typhimurium due to site-specific bacterial PAMP expression. Mucosal Immunol. 13 (3), 530–544. doi:10.1038/s41385-019-0247-0
He, H., Wang, L., Qiao, Y., Yang, B., Yin, D., and He, M. (2021). Epigallocatechin-3-gallate pretreatment alleviates doxorubicin-induced ferroptosis and cardiotoxicity by upregulating AMPKα2 and activating adaptive autophagy. Redox Biol. 48, 102185. doi:10.1016/j.redox.2021.102185
Hu, S., Liu, B., Yang, M., Mao, S., Ju, H., Liu, Z., et al. (2023). Carnosic acid protects against doxorubicin-induced cardiotoxicity through enhancing the Nrf2/HO-1 pathway. Food Funct. 14 (8), 3849–3862. doi:10.1039/d2fo03904d
Huang, M., Lu, J. J., Huang, M. Q., Bao, J. L., Chen, X. P., and Wang, Y. T. (2012). Terpenoids: natural products for cancer therapy. Expert Opin. Investig. Drugs 21 (12), 1801–1818. doi:10.1517/13543784.2012.727395
Huang, P. P., Fu, J., Liu, L. H., Wu, K. F., Liu, H. X., Qi, B. M., et al. (2020). Honokiol antagonizes doxorubicin-induced cardiomyocyte senescence by inhibiting TXNIP-mediated NLRP3 inflammasome activation. Int. J. Mol. Med. 45 (1), 186–194. doi:10.3892/ijmm.2019.4393
Ibrahim Fouad, G., and Ahmed, K. A. (2022). Curcumin ameliorates doxorubicin-induced cardiotoxicity and hepatotoxicity via suppressing oxidative stress and modulating iNOS, NF-κB, and TNF-α in rats. Cardiovasc Toxicol. 22 (2), 152–166. doi:10.1007/s12012-021-09710-w
Juang, Y. P., and Liang, P. H. (2020). Biological and pharmacological effects of synthetic saponins. Molecules 25 (21), 4974. doi:10.3390/molecules25214974
Kabel, A. M., Salama, S. A., Adwas, A. A., and Estfanous, R. S. (2021). Targeting oxidative stress, NLRP3 inflammasome, and autophagy by fraxetin to combat doxorubicin-induced cardiotoxicity. Pharm. (Basel) 14 (11), 1188. doi:10.3390/ph14111188
Kaźmierczak-Barańska, J., Boguszewska, K., Adamus-Grabicka, A., and Karwowski, B. T. (2020). Two faces of vitamin C-antioxidative and pro-oxidative agent. Nutrients 12 (5), 1501. doi:10.3390/nu12051501
Kitazumi, I., and Tsukahara, M. (2011). Regulation of DNA fragmentation: the role of caspases and phosphorylation. Febs j 278 (3), 427–441. doi:10.1111/j.1742-4658.2010.07975.x
Komada, T., Chung, H., Lau, A., Platnich, J. M., Beck, P. L., Benediktsson, H., et al. (2018). Macrophage uptake of necrotic cell DNA activates the AIM2 inflammasome to regulate a proinflammatory phenotype in CKD. J. Am. Soc. Nephrol. 29 (4), 1165–1181. doi:10.1681/asn.2017080863
Koppenol, W. H. (2001). The Haber-Weiss cycle--70 years later. Redox Rep. 6 (4), 229–234. doi:10.1179/135100001101536373
Kumar, H., Kawai, T., and Akira, S. (2009). Toll-like receptors and innate immunity. Biochem. Biophys. Res. Commun. 388 (4), 621–625. doi:10.1016/j.bbrc.2009.08.062
Lapczynski, A., Bhatia, S. P., Letizia, C. S., and Api, A. M. (2008). Fragrance material review on nerolidol (isomer unspecified). Food Chem. Toxicol. 46 (Suppl. 11), S247–S250. doi:10.1016/j.fct.2008.06.063
Latz, E., Xiao, T. S., and Stutz, A. (2013). Activation and regulation of the inflammasomes. Nat. Rev. Immunol. 13 (6), 397–411. doi:10.1038/nri3452
Li, C., Wan, W., Ye, T., Sun, Y., Chen, X., Liu, X., et al. (2021a). Pinocembrin alleviates lipopolysaccharide-induced myocardial injury and cardiac dysfunction in rats by inhibiting p38/JNK MAPK pathway. Life Sci. 277, 119418. doi:10.1016/j.lfs.2021.119418
Li, J., Luo, T., Zhao, Y., Wang, D., Jin, Y., Wu, Z., et al. (2024). Cardioprotective potentials of myricetin on doxorubicin-induced cardiotoxicity based on biochemical and transcriptomic analysis. Biomed. Pharmacother. 175, 116748. doi:10.1016/j.biopha.2024.116748
Li, Q., Tian, Z., Wang, M., Kou, J., Wang, C., Rong, X., et al. (2019). Luteoloside attenuates neuroinflammation in focal cerebral ischemia in rats via regulation of the PPARγ/Nrf2/NF-κB signaling pathway. Int. Immunopharmacol. 66, 309–316. doi:10.1016/j.intimp.2018.11.044
Li, S., Sun, Y., Song, M., Song, Y., Fang, Y., Zhang, Q., et al. (2021b). NLRP3/caspase-1/GSDMD-mediated pyroptosis exerts a crucial role in astrocyte pathological injury in mouse model of depression. JCI Insight 6 (23), e146852. doi:10.1172/jci.insight.146852
Li, W. W., Li, D., Qin, Y., Sun, C. X., Wang, Y. L., Gao, L., et al. (2021c). Cardioprotective effects of Amentoflavone by suppression of apoptosis and inflammation on an in vitro and vivo model of myocardial ischemia-reperfusion injury. Int. Immunopharmacol. 101 (Pt B), 108296. doi:10.1016/j.intimp.2021.108296
Li, Y., Fu, T. M., Lu, A., Witt, K., Ruan, J., Shen, C., et al. (2018). Cryo-EM structures of ASC and NLRC4 CARD filaments reveal a unified mechanism of nucleation and activation of caspase-1. Proc. Natl. Acad. Sci. U S A. 115 (43), 10845–10852. doi:10.1073/pnas.1810524115
Liao, L. Z., Chen, Y. L., Lu, L. H., Zhao, Y. H., Guo, H. L., and Wu, W. K. (2013). Polysaccharide from Fuzi likely protects against starvation-induced cytotoxicity in H9c2 cells by increasing autophagy through activation of the AMPK/mTOR pathway. Am. J. Chin. Med. 41 (2), 353–367. doi:10.1142/s0192415x13500262
Lin, Z., and Wang, J. (2023). Taxifolin protects against doxorubicin-induced cardiotoxicity and ferroptosis by adjusting microRNA-200a-mediated Nrf2 signaling pathway. Heliyon 9 (11), e22011. doi:10.1016/j.heliyon.2023.e22011
Lipshultz, S. E., Lipsitz, S. R., Sallan, S. E., Simbre, V. C., Shaikh, S. L., Mone, S. M., et al. (2002). Long-term enalapril therapy for left ventricular dysfunction in doxorubicin-treated survivors of childhood cancer. J. Clin. Oncol. 20 (23), 4517–4522. doi:10.1200/jco.2002.12.102
Liu, A., Xun, S., Zhou, G., Zhang, Y., and Lin, L. (2023). Honokiol alleviates sepsis-associated cardiac dysfunction via attenuating inflammation, apoptosis and oxidative stress. J. Pharm. Pharmacol. 75 (3), 397–406. doi:10.1093/jpp/rgac102
Liu, H., Ren, C., Liu, W., Jiang, X., Wang, L., Zhu, B., et al. (2017). Embryotoxicity estimation of commonly used compounds with embryonic stem cell test. Mol. Med. Rep. 16 (1), 263–271. doi:10.3892/mmr.2017.6552
Liu, J., Wang, Y., Qiu, L., Yu, Y., and Wang, C. (2014). Saponins of Panax notoginseng: chemistry, cellular targets and therapeutic opportunities in cardiovascular diseases. Expert Opin. Investig. Drugs 23 (4), 523–539. doi:10.1517/13543784.2014.892582
Liu, S., Liu, J., Su, N., Wei, S., Xie, N., Li, X., et al. (2024). An integrated network pharmacology and RNA-seq approach for exploring the protective effect of andrographolide in doxorubicin-induced cardiotoxicity. Cardiovasc Drugs Ther. doi:10.1007/s10557-024-07555-3
Liu, X., Zhang, Z., Ruan, J., Pan, Y., Magupalli, V. G., Wu, H., et al. (2016). Inflammasome-activated gasdermin D causes pyroptosis by forming membrane pores. Nature 535 (7610), 153–158. doi:10.1038/nature18629
Liu, Y., Sun, Y., and Huang, G. (2018). Preparation and antioxidant activities of important traditional plant polysaccharides. Int. J. Biol. Macromol. 111, 780–786. doi:10.1016/j.ijbiomac.2018.01.086
Lu, A., Magupalli, V. G., Ruan, J., Yin, Q., Atianand, M. K., Vos, M. R., et al. (2014). Unified polymerization mechanism for the assembly of ASC-dependent inflammasomes. Cell 156 (6), 1193–1206. doi:10.1016/j.cell.2014.02.008
Ludlow, L. E., Johnstone, R. W., and Clarke, C. J. (2005). The HIN-200 family: more than interferon-inducible genes? Exp. Cell Res. 308 (1), 1–17. doi:10.1016/j.yexcr.2005.03.032
Ma, Y., Lin, H., Li, Y., and An, Z. (2024). Amentoflavone induces ferroptosis to alleviate proliferation, migration, invasion and inflammation in rheumatoid arthritis fibroblast-like synoviocytes by inhibiting PIN1. Cell Biochem. Biophys. 83, 1299–1312. doi:10.1007/s12013-024-01563-8
Maayah, Z. H., Alam, A. S., Takahara, S., Soni, S., Ferdaoussi, M., Matsumura, N., et al. (2021). Resveratrol reduces cardiac NLRP3-inflammasome activation and systemic inflammation to lessen doxorubicin-induced cardiotoxicity in juvenile mice. FEBS Lett. 595 (12), 1681–1695. doi:10.1002/1873-3468.14091
Maiti, K., Mukherjee, K., Gantait, A., Saha, B. P., and Mukherjee, P. K. (2007). Curcumin-phospholipid complex: preparation, therapeutic evaluation and pharmacokinetic study in rats. Int. J. Pharm. 330 (1-2), 155–163. doi:10.1016/j.ijpharm.2006.09.025
Martínez-Coria, H., Arrieta-Cruz, I., Gutiérrez-Juárez, R., and López-Valdés, H. E. (2023). Anti-inflammatory effects of flavonoids in common neurological disorders associated with aging. Int. J. Mol. Sci. 24 (5), 4297. doi:10.3390/ijms24054297
McKay, D. L., and Blumberg, J. B. (2006). A review of the bioactivity and potential health benefits of chamomile tea (Matricaria recutita L.). Phytother. Res. 20 (7), 519–530. doi:10.1002/ptr.1900
Meeran, M. F. N., Azimullah, S., Mamoudh, H. H., Sharma, C., Kumar, S., Goyal, S. N., et al. (2021). Nerolidol, a sesquiterpene from the essential oils of aromatic plants, attenuates doxorubicin-induced chronic cardiotoxicity in rats. J. Agric. Food Chem. 69 (26), 7334–7343. doi:10.1021/acs.jafc.0c05667
Meeran, M. F. N., Laham, F., Al-Taee, H., Azimullah, S., and Ojha, S. (2018). Protective effects of α-bisabolol on altered hemodynamics, lipid peroxidation, and nonenzymatic antioxidants in isoproterenol-induced myocardial infarction: in vivo and in vitro evidences. J. Biochem. Mol. Toxicol. 32 (10), e22200. doi:10.1002/jbt.22200
Mezzaroma, E., Abbate, A., and Toldo, S. (2021). The inflammasome in heart failure. Curr. Opin. Physiol. 19, 105–112. doi:10.1016/j.cophys.2020.09.013
Milano, G., Raucci, A., Scopece, A., Daniele, R., Guerrini, U., Sironi, L., et al. (2014). Doxorubicin and trastuzumab regimen induces biventricular failure in mice. J. Am. Soc. Echocardiogr. 27 (5), 568–579. doi:10.1016/j.echo.2014.01.014
Mohammadpour, Y. H., Khodayar, M. J., Khorsandi, L., and Kalantar, H. (2024). Betaine alleviates doxorubicin-related cardiotoxicity via suppressing oxidative stress and inflammation via the NLRP3/SIRT1 pathway. Naunyn Schmiedeb. Arch. Pharmacol. 397 (12), 9981–9990. doi:10.1007/s00210-024-03261-x
Motta, V., Soares, F., Sun, T., and Philpott, D. J. (2015). NOD-like receptors: versatile cytosolic sentinels. Physiol. Rev. 95 (1), 149–178. doi:10.1152/physrev.00009.2014
Ms, S. A. B., Waldman Ph, D. H., Krings Ph, D. B., Lamberth Ph, D. J., Smith Ph, D. J., and McAllister Ph, D. M. (2020). Effect of curcumin supplementation on exercise-induced oxidative stress, inflammation, muscle damage, and muscle soreness. J. Diet. Suppl. 17 (4), 401–414. doi:10.1080/19390211.2019.1604604
Munroe, M. E., Businga, T. R., Kline, J. N., and Bishop, G. A. (2010). Anti-inflammatory effects of the neurotransmitter agonist Honokiol in a mouse model of allergic asthma. J. Immunol. 185 (9), 5586–5597. doi:10.4049/jimmunol.1000630
Murphy, A. J., Kraakman, M. J., Kammoun, H. L., Dragoljevic, D., Lee, M. K., Lawlor, K. E., et al. (2016). IL-18 production from the NLRP1 inflammasome prevents obesity and metabolic syndrome. Cell Metab. 23 (1), 155–164. doi:10.1016/j.cmet.2015.09.024
Nagoor Meeran, M. F., Arunachalam, S., Azimullah, S., Saraswathiamma, D., Albawardi, A., Almarzooqi, S., et al. (2023). α-Bisabolol, a dietary sesquiterpene, attenuates doxorubicin-induced acute cardiotoxicity in rats by inhibiting cellular signaling pathways, nrf2/keap-1/HO-1, akt/mTOR/GSK-3β, NF-κB/p38/MAPK, and NLRP3 inflammasomes regulating oxidative stress and inflammatory cascades. Int. J. Mol. Sci. 24 (18), 14013. doi:10.3390/ijms241814013
Nagoor Meeran, M. F., Laham, F., Azimullah, S., Tariq, S., and Ojha, S. (2019). α-Bisabolol abrogates isoproterenol-induced myocardial infarction by inhibiting mitochondrial dysfunction and intrinsic pathway of apoptosis in rats. Mol. Cell Biochem. 453 (1-2), 89–102. doi:10.1007/s11010-018-3434-5
Octavia, Y., Tocchetti, C. G., Gabrielson, K. L., Janssens, S., Crijns, H. J., and Moens, A. L. (2012). Doxorubicin-induced cardiomyopathy: from molecular mechanisms to therapeutic strategies. J. Mol. Cell Cardiol. 52 (6), 1213–1225. doi:10.1016/j.yjmcc.2012.03.006
Pan, L., Zhang, X. F., Wei, W. S., Zhang, J., and Li, Z. Z. (2020). The cardiovascular protective effect and mechanism of calycosin and its derivatives. Chin. J. Nat. Med. 18 (12), 907–915. doi:10.1016/s1875-5364(20)60034-6
Pavillard, L. E., Cañadas-Lozano, D., Alcocer-Gómez, E., Marín-Aguilar, F., Pereira, S., Robertson, A. A. B., et al. (2017). NLRP3-inflammasome inhibition prevents high fat and high sugar diets-induced heart damage through autophagy induction. Oncotarget 8 (59), 99740–99756. doi:10.18632/oncotarget.20763
Petrovski, G., Gurusamy, N., and Das, D. K. (2011). Resveratrol in cardiovascular health and disease. Ann. N. Y. Acad. Sci. 1215, 22–33. doi:10.1111/j.1749-6632.2010.05843.x
Pillai, V. B., Kanwal, A., Fang, Y. H., Sharp, W. W., Samant, S., Arbiser, J., et al. (2017). Honokiol, an activator of Sirtuin-3 (SIRT3) preserves mitochondria and protects the heart from doxorubicin-induced cardiomyopathy in mice. Oncotarget 8 (21), 34082–34098. doi:10.18632/oncotarget.16133
Platnich, J. M., and Muruve, D. A. (2019). NOD-like receptors and inflammasomes: a review of their canonical and non-canonical signaling pathways. Arch. Biochem. Biophys. 670, 4–14. doi:10.1016/j.abb.2019.02.008
Priya, L. B., Baskaran, R., Huang, C. Y., and Padma, V. V. (2017). Neferine ameliorates cardiomyoblast apoptosis induced by doxorubicin: possible role in modulating NADPH oxidase/ROS-mediated NFκB redox signaling cascade. Sci. Rep. 7 (1), 12283. doi:10.1038/s41598-017-12060-9
Qin, L., Zhao, Y., Zhang, B., and Li, Y. (2018). Amentoflavone improves cardiovascular dysfunction and metabolic abnormalities in high fructose and fat diet-fed rats. Food Funct. 9 (1), 243–252. doi:10.1039/c7fo01095h
Qu, Y., Gao, R., Wei, X., Sun, X., Yang, K., Shi, H., et al. (2022). Gasdermin D mediates endoplasmic reticulum stress via FAM134B to regulate cardiomyocyte autophagy and apoptosis in doxorubicin-induced cardiotoxicity. Cell Death Dis. 13 (10), 901. doi:10.1038/s41419-022-05333-3
Rasul, A., Millimouno, F. M., Ali Eltayb, W., Ali, M., Li, J., and Li, X. (2013). Pinocembrin: a novel natural compound with versatile pharmacological and biological activities. Biomed. Res. Int. 2013, 379850. doi:10.1155/2013/379850
Rathinam, V. A., Vanaja, S. K., and Fitzgerald, K. A. (2012). Regulation of inflammasome signaling. Nat. Immunol. 13 (4), 333–342. doi:10.1038/ni.2237
Rawat, P. S., Jaiswal, A., Khurana, A., Bhatti, J. S., and Navik, U. (2021). Doxorubicin-induced cardiotoxicity: an update on the molecular mechanism and novel therapeutic strategies for effective management. Biomed. Pharmacother. 139, 111708. doi:10.1016/j.biopha.2021.111708
Robe, K., Conejero, G., Gao, F., Lefebvre-Legendre, L., Sylvestre-Gonon, E., Rofidal, V., et al. (2021). Coumarin accumulation and trafficking in Arabidopsis thaliana: a complex and dynamic process. New Phytol. 229 (4), 2062–2079. doi:10.1111/nph.17090
Robinson, K., Mock, C., and Liang, D. (2015). Pre-formulation studies of resveratrol. Drug Dev. Ind. Pharm. 41 (9), 1464–1469. doi:10.3109/03639045.2014.958753
Sahu, B. D., Putcha, U. K., Kuncha, M., Rachamalla, S. S., and Sistla, R. (2014). Carnosic acid promotes myocardial antioxidant response and prevents isoproterenol-induced myocardial oxidative stress and apoptosis in mice. Mol. Cell Biochem. 394 (1-2), 163–176. doi:10.1007/s11010-014-2092-5
Salucci, S., Burattini, S., Giordano, F. M., Lucarini, S., Diamantini, G., and Falcieri, E. (2017). Further highlighting on the prevention of oxidative damage by polyphenol-rich wine extracts. J. Med. Food 20 (4), 410–419. doi:10.1089/jmf.2016.0153
Sangweni, N. F., Gabuza, K., Huisamen, B., Mabasa, L., van Vuuren, D., and Johnson, R. (2022). Molecular insights into the pathophysiology of doxorubicin-induced cardiotoxicity: a graphical representation. Arch. Toxicol. 96 (6), 1541–1550. doi:10.1007/s00204-022-03262-w
Shabalala, S., Muller, C. J. F., Louw, J., and Johnson, R. (2017). Polyphenols, autophagy and doxorubicin-induced cardiotoxicity. Life Sci. 180, 160–170. doi:10.1016/j.lfs.2017.05.003
Shao, W., Yeretssian, G., Doiron, K., Hussain, S. N., and Saleh, M. (2007). The caspase-1 digestome identifies the glycolysis pathway as a target during infection and septic shock. J. Biol. Chem. 282 (50), 36321–36329. doi:10.1074/jbc.M708182200
Sharma, B. R., and Kanneganti, T. D. (2021). NLRP3 inflammasome in cancer and metabolic diseases. Nat. Immunol. 22 (5), 550–559. doi:10.1038/s41590-021-00886-5
Singh, S., and Jha, S. (2018). NLRs as helpline in the brain: mechanisms and therapeutic implications. Mol. Neurobiol. 55 (10), 8154–8178. doi:10.1007/s12035-018-0957-4
Song, X., Tan, L., Wang, M., Ren, C., Guo, C., Yang, B., et al. (2021). Myricetin: a review of the most recent research. Biomed. Pharmacother. 134, 111017. doi:10.1016/j.biopha.2020.111017
Songbo, M., Lang, H., Xinyong, C., Bin, X., Ping, Z., and Liang, S. (2019). Oxidative stress injury in doxorubicin-induced cardiotoxicity. Toxicol. Lett. 307, 41–48. doi:10.1016/j.toxlet.2019.02.013
Sun, M., Zhang, X., Tan, B., Zhang, Q., Zhao, X., and Dong, D. (2024). Potential role of endoplasmic reticulum stress in doxorubicin-induced cardiotoxicity-an update. Front. Pharmacol. 15, 1415108. doi:10.3389/fphar.2024.1415108
Sun, Y., Liu, Y., and Chen, K. (2016). Roles and mechanisms of ginsenoside in cardiovascular diseases: progress and perspectives. Sci. China Life Sci. 59 (3), 292–298. doi:10.1007/s11427-016-5007-8
Sun, Z., Lu, W., Lin, N., Lin, H., Zhang, J., Ni, T., et al. (2020). Dihydromyricetin alleviates doxorubicin-induced cardiotoxicity by inhibiting NLRP3 inflammasome through activation of SIRT1. Biochem. Pharmacol. 175, 113888. doi:10.1016/j.bcp.2020.113888
Tai, P., Chen, X., Jia, G., Chen, G., Gong, L., Cheng, Y., et al. (2023). WGX50 mitigates doxorubicin-induced cardiotoxicity through inhibition of mitochondrial ROS and ferroptosis. J. Transl. Med. 21 (1), 823. doi:10.1186/s12967-023-04715-1
Tan, L., Bogush, N., Naqvi, E., Calvert, J. W., Graham, R. M., Taylor, W. R., et al. (2021). Thyroid hormone plus dual-specificity phosphatase-5 siRNA increases the number of cardiac muscle cells and improves left ventricular contractile function in chronic doxorubicin-injured hearts. Theranostics 11 (10), 4790–4808. doi:10.7150/thno.57456
Tattoli, I., Travassos, L. H., Carneiro, L. A., Magalhaes, J. G., and Girardin, S. E. (2007). The Nodosome: nod1 and Nod2 control bacterial infections and inflammation. Semin. Immunopathol. 29 (3), 289–301. doi:10.1007/s00281-007-0083-2
Tian, W., Zhang, P., Yang, L., Song, P., Zhao, J., Wang, H., et al. (2024). Astragaloside IV alleviates doxorubicin-induced cardiotoxicity by inhibiting cardiomyocyte pyroptosis through the SIRT1/NLRP3 pathway. Am. J. Chin. Med. 52 (2), 453–469. doi:10.1142/s0192415x24500198
Tian, Z., Zhang, X., and Sun, M. (2021). Phytochemicals mediate autophagy against osteoarthritis by maintaining cartilage homeostasis. Front. Pharmacol. 12, 795058. doi:10.3389/fphar.2021.795058
Toldo, S., and Abbate, A. (2018). The NLRP3 inflammasome in acute myocardial infarction. Nat. Rev. Cardiol. 15 (4), 203–214. doi:10.1038/nrcardio.2017.161
Toldo, S., Mezzaroma, E., Buckley, L. F., Potere, N., Di Nisio, M., Biondi-Zoccai, G., et al. (2022). Targeting the NLRP3 inflammasome in cardiovascular diseases. Pharmacol. Ther. 236, 108053. doi:10.1016/j.pharmthera.2021.108053
Toldo, S., Mezzaroma, E., Mauro, A. G., Salloum, F., Van Tassell, B. W., and Abbate, A. (2015). The inflammasome in myocardial injury and cardiac remodeling. Antioxid. Redox Signal 22 (13), 1146–1161. doi:10.1089/ars.2014.5989
Vitale, R., Marzocco, S., and Popolo, A. (2024). Role of oxidative stress and inflammation in doxorubicin-induced cardiotoxicity: a brief account. Int. J. Mol. Sci. 25 (13), 7477. doi:10.3390/ijms25137477
Volkova, M., and Russell, R. (2011). Anthracycline cardiotoxicity: prevalence, pathogenesis and treatment. Curr. Cardiol. Rev. 7 (4), 214–220. doi:10.2174/157340311799960645
Vollono, L., Falconi, M., Gaziano, R., Iacovelli, F., Dika, E., Terracciano, C., et al. (2019). Potential of curcumin in skin disorders. Nutrients 11 (9), 2169. doi:10.3390/nu11092169
Wang, Q. L., Li, H., Li, X. X., Cui, C. Y., Wang, R., Yu, N. X., et al. (2012). Acute and 30-day oral toxicity studies of administered carnosic acid. Food Chem. Toxicol. 50 (12), 4348–4355. doi:10.1016/j.fct.2012.08.057
Wang, T. J., Liu, H. T., Lai, Y. H., Jan, T. R., Nomura, N., Chang, H. W., et al. (2018). Honokiol, a polyphenol natural compound, attenuates cisplatin-induced acute cytotoxicity in renal epithelial cells through cellular oxidative stress and cytoskeleton modulations. Front. Pharmacol. 9, 357. doi:10.3389/fphar.2018.00357
Wang, X., Ji, Y., Jin, D., Qi, J., Hou, X., Zhao, W., et al. (2022). Natural polysaccharide β-glucan protects against doxorubicin-induced cardiotoxicity by suppressing oxidative stress. Nutrients 14 (4), 906. doi:10.3390/nu14040906
Wang, Z., Cao, Z., Yue, Z., and Yang, Z. (2023). Research progress of dihydromyricetin in the treatment of diabetes mellitus. Front. Endocrinol. (Lausanne) 14, 1216907. doi:10.3389/fendo.2023.1216907
Wei, S., Ma, W., Jiang, C., Liu, J., Liu, J., Zhang, B., et al. (2023). Hyperoside prevents doxorubicin-induced cardiotoxicity by inhibiting NOXs/ROS/NLRP3 inflammasome signaling pathway. Phytother. Res. 37 (9), 4196–4209. doi:10.1002/ptr.7900
Wen, J., Li, L., Yang, Y., Ou, D., Yang, J., Xie, J., et al. (2024). Phytochemicals targeting ferroptosis in cardiovascular diseases: recent advances and therapeutic perspectives. Phytother. Res. 38 (9), 4386–4405. doi:10.1002/ptr.8278
Wicherska-Pawłowska, K., Wróbel, T., and Rybka, J. (2021). Toll-like receptors (TLRs), NOD-like receptors (NLRs), and RIG-I-like receptors (RLRs) in innate immunity. TLRs, NLRs, and RLRs ligands as immunotherapeutic agents for hematopoietic diseases. Int. J. Mol. Sci. 22 (24), 13397. doi:10.3390/ijms222413397
Wu, B. B., Leung, K. T., and Poon, E. N. (2022). Mitochondrial-targeted therapy for doxorubicin-induced cardiotoxicity. Int. J. Mol. Sci. 23 (3), 1912. doi:10.3390/ijms23031912
Xia, C. Y., Guo, Y. X., Lian, W. W., Yan, Y., Ma, B. Z., Cheng, Y. C., et al. (2023). The NLRP3 inflammasome in depression: potential mechanisms and therapies. Pharmacol. Res. 187, 106625. doi:10.1016/j.phrs.2022.106625
Xiong, F., Pu, L., Wang, D., Peng, F., Sun, L., Yu, Q., et al. (2024). Investigation of polysaccharide from Radix Aconiti Lateralis Preparata (Fuzi) cardio protective effect on doxorubicin-induced chronic cardiotoxicity. J. Pharm. Pharmacol. 76 (4), 391–404. doi:10.1093/jpp/rgae001
Xu, J., Zhang, Z., Hu, H., Yang, Y., Xiao, C., Xi, L., et al. (2024). Synergistic antitumor effects of Peiminine and Doxorubicin on breast cancer through enhancing DNA damage via ZEB1. Biomed. Pharmacother. 173, 116353. doi:10.1016/j.biopha.2024.116353
Xu, S., Chen, S., Xia, W., Sui, H., and Fu, X. (2022). Hyperoside: a review of its structure, synthesis, pharmacology, pharmacokinetics and toxicity. Molecules 27 (9), 3009. doi:10.3390/molecules27093009
Yan, H. C., Qu, H. D., Sun, L. R., Li, S. J., Cao, X., Fang, Y. Y., et al. (2010). Fuzi polysaccharide-1 produces antidepressant-like effects in mice. Int. J. Neuropsychopharmacol. 13 (5), 623–633. doi:10.1017/s1461145709990733
Yang, H. B., Lu, Z. Y., Yuan, W., Li, W. D., and Mao, S. (2022). Selenium attenuates doxorubicin-induced cardiotoxicity through nrf2-NLRP3 pathway. Biol. Trace Elem. Res. 200 (6), 2848–2856. doi:10.1007/s12011-021-02891-z
Yang, Y., Lv, J., Jiang, S., Ma, Z., Wang, D., Hu, W., et al. (2016). The emerging role of Toll-like receptor 4 in myocardial inflammation. Cell Death Dis. 7 (5), e2234. doi:10.1038/cddis.2016.140
Yao, H., He, Q., Huang, C., Wei, S., Gong, Y., Li, X., et al. (2022). Panaxatriol saponin ameliorates myocardial infarction-induced cardiac fibrosis by targeting Keap1/Nrf2 to regulate oxidative stress and inhibit cardiac-fibroblast activation and proliferation. Free Radic. Biol. Med. 190, 264–275. doi:10.1016/j.freeradbiomed.2022.08.016
Yi, X., Wang, Q., Zhang, M., Shu, Q., and Zhu, J. (2024). Ferroptosis: a novel therapeutic target of natural products against doxorubicin-induced cardiotoxicity. Biomed. Pharmacother. 178, 117217. doi:10.1016/j.biopha.2024.117217
Yi, Y. S. (2020). Functional crosstalk between non-canonical caspase-11 and canonical NLRP3 inflammasomes during infection-mediated inflammation. Immunology 159 (2), 142–155. doi:10.1111/imm.13134
Yi, Y. S. (2023). Regulatory roles of flavonoids in caspase-11 non-canonical inflammasome-mediated inflammatory responses and diseases. Int. J. Mol. Sci. 24 (12), 10402. doi:10.3390/ijms241210402
Yin, Q., Fu, T. M., Li, J., and Wu, H. (2015). Structural biology of innate immunity. Annu. Rev. Immunol. 33, 393–416. doi:10.1146/annurev-immunol-032414-112258
Yu, W., Qin, X., Zhang, Y., Qiu, P., Wang, L., Zha, W., et al. (2020). Curcumin suppresses doxorubicin-induced cardiomyocyte pyroptosis via a PI3K/Akt/mTOR-dependent manner. Cardiovasc Diagn Ther. 10 (4), 752–769. doi:10.21037/cdt-19-707
Yuan, C., Liu, L., Zhao, Y., and Wang, K. (2024). Puerarin inhibits Staphylococcus aureus-induced endometritis through attenuating inflammation and ferroptosis via regulating the P2X7/NLRP3 signalling pathway. J. Cell Mol. Med. 28 (14), e18550. doi:10.1111/jcmm.18550
Yuan, W., Wang, J., An, X., Dai, M., Jiang, Z., Zhang, L., et al. (2021). UPLC-MS/MS method for the determination of hyperoside and application to pharmacokinetics study in rat after different administration routes. Chromatographia 84 (3), 249–256. doi:10.1007/s10337-020-04002-x
Yun, W., Qian, L., Yuan, R., and Xu, H. (2021). Periplocymarin alleviates doxorubicin-induced heart failure and excessive accumulation of ceramides. Front. Cardiovasc Med. 8, 732554. doi:10.3389/fcvm.2021.732554
Zhai, J., Tao, L., Zhang, S., Gao, H., Zhang, Y., Sun, J., et al. (2020). Calycosin ameliorates doxorubicin-induced cardiotoxicity by suppressing oxidative stress and inflammation via the sirtuin 1-NOD-like receptor protein 3 pathway. Phytother. Res. 34 (3), 649–659. doi:10.1002/ptr.6557
Zhang, E., Shang, C., Ma, M., Zhang, X., Liu, Y., Song, S., et al. (2023). Polyguluronic acid alleviates doxorubicin-induced cardiotoxicity by suppressing Peli1-NLRP3 inflammasome-mediated pyroptosis. Carbohydr. Polym. 321, 121334. doi:10.1016/j.carbpol.2023.121334
Zhang, L., Fan, C., Jiao, H. C., Zhang, Q., Jiang, Y. H., Cui, J., et al. (2022). Calycosin alleviates doxorubicin-induced cardiotoxicity and pyroptosis by inhibiting NLRP3 inflammasome activation. Oxid. Med. Cell Longev. 2022, 1733834. doi:10.1155/2022/1733834
Zhang, L. X., Li, C. X., Kakar, M. U., Khan, M. S., Wu, P. F., Amir, R. M., et al. (2021). Resveratrol (RV): a pharmacological review and call for further research. Biomed. Pharmacother. 143, 112164. doi:10.1016/j.biopha.2021.112164
Zhang, N., Feng, H., Liao, H. H., Chen, S., Yang, Z., Deng, W., et al. (2018). Myricetin attenuated LPS induced cardiac injury in vivo and in vitro. Phytother. Res. 32 (3), 459–470. doi:10.1002/ptr.5989
Zhang, Q. L., Yang, J. J., and Zhang, H. S. (2019). Carvedilol (CAR) combined with carnosic acid (CAA) attenuates doxorubicin-induced cardiotoxicity by suppressing excessive oxidative stress, inflammation, apoptosis and autophagy. Biomed. Pharmacother. 109, 71–83. doi:10.1016/j.biopha.2018.07.037
Zhang, Z., He, Y., Liu, H., Liu, Y., Wu, T., Li, R., et al. (2024). NLRP3 regulates ferroptosis via the JAK2/STAT3 pathway in asthma inflammation: insights from in vivo and in vitro studies. Int. Immunopharmacol. 143 (Pt 2), 113416. doi:10.1016/j.intimp.2024.113416
Zhao, X., Tian, Z., Sun, M., and Dong, D. (2023). Nrf2: a dark horse in doxorubicin-induced cardiotoxicity. Cell Death Discov. 9 (1), 261. doi:10.1038/s41420-023-01565-0
Zhou, L., Li, M., Chai, Z., Zhang, J., Cao, K., Deng, L., et al. (2023). Anticancer effects and mechanisms of astragaloside‑IV (Review). Oncol. Rep. 49 (1), 5. (Review). doi:10.3892/or.2022.8442
Zhou, S., and Huang, G. (2021). Preparation, structure and activity of polysaccharide phosphate esters. Biomed. Pharmacother. 144, 112332. doi:10.1016/j.biopha.2021.112332
Zhou, S., Zhong, Z., Huang, P., Xiang, B., Li, X., Dong, H., et al. (2021). IL-6/STAT3 induced neuron apoptosis in hypoxia by downregulating ATF6 expression. Front. Physiol. 12, 729925. doi:10.3389/fphys.2021.729925
Zhu, J., Bao, Z., Hu, Z., Wu, S., Tian, C., Zhou, Y., et al. (2024a). Myricetin alleviates diabetic cardiomyopathy by regulating gut microbiota and their metabolites. Nutr. Diabetes 14 (1), 10. doi:10.1038/s41387-024-00268-4
Zhu, M., Chen, Y., Cheng, L., Li, X., Shen, Y., Guo, G., et al. (2024b). Calsyntenin-1 promotes doxorubicin-induced dilated cardiomyopathy in rats. Cardiovasc Drugs Ther. 38 (2), 237–252. doi:10.1007/s10557-022-07389-x
Zilinyi, R., Czompa, A., Czegledi, A., Gajtko, A., Pituk, D., Lekli, I., et al. (2018). The cardioprotective effect of metformin in doxorubicin-induced cardiotoxicity: the role of autophagy. Molecules 23 (5), 1184. doi:10.3390/molecules23051184
Keywords: phytochemicals, NLRP3 inflammasome, pyroptosis, DOX-induced cardiotoxicity, myocardial injury
Citation: Zhao X-P, Duan L, Zhao Q-R, Lv X, Tian N-Y, Yang S-L and Dong K (2025) NLRP3 inflammasome as a therapeutic target in doxorubicin-induced cardiotoxicity: role of phytochemicals. Front. Pharmacol. 16:1567312. doi: 10.3389/fphar.2025.1567312
Received: 27 January 2025; Accepted: 07 April 2025;
Published: 17 April 2025.
Edited by:
Antonio Carlos Campos De Carvalho, Federal University of Rio de Janeiro, BrazilReviewed by:
Emiliano Medei, Federal University of Rio de Janeiro, BrazilCopyright © 2025 Zhao, Duan, Zhao, Lv, Tian, Yang and Dong. This is an open-access article distributed under the terms of the Creative Commons Attribution License (CC BY). The use, distribution or reproduction in other forums is permitted, provided the original author(s) and the copyright owner(s) are credited and that the original publication in this journal is cited, in accordance with accepted academic practice. No use, distribution or reproduction is permitted which does not comply with these terms.
*Correspondence: Sheng-Lei Yang, eTE3NjE2MjUxOTM5QDE2My5jb20=; Kun Dong, MTM5MzM1MzczNzNAMTYzLmNvbQ==
†These authors have contributed equally to this work and share first authorship
Disclaimer: All claims expressed in this article are solely those of the authors and do not necessarily represent those of their affiliated organizations, or those of the publisher, the editors and the reviewers. Any product that may be evaluated in this article or claim that may be made by its manufacturer is not guaranteed or endorsed by the publisher.
Research integrity at Frontiers
Learn more about the work of our research integrity team to safeguard the quality of each article we publish.