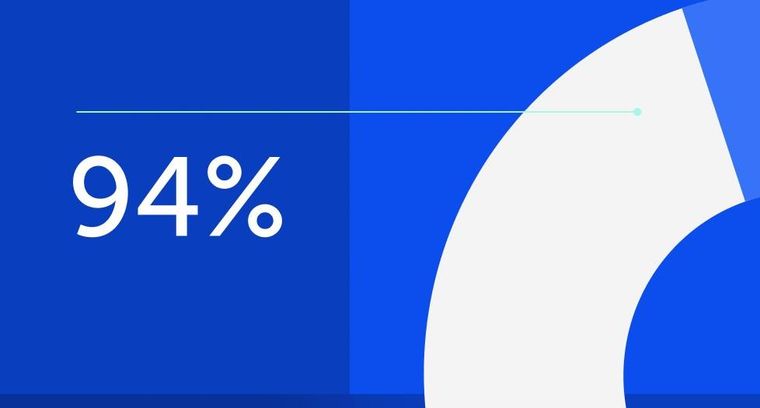
94% of researchers rate our articles as excellent or good
Learn more about the work of our research integrity team to safeguard the quality of each article we publish.
Find out more
REVIEW article
Front. Pharmacol., 28 March 2025
Sec. Pharmacology of Anti-Cancer Drugs
Volume 16 - 2025 | https://doi.org/10.3389/fphar.2025.1564911
Cancer is a leading cause of mortality worldwide, with most conventional treatments lacking efficacy and having significant challenges like drug resistance. Finding new molecules is quite challenging in terms of cost, time and setbacks. Hence, drug repurposing is considered sensible for skipping the long process of drug development. Dibenzazepine carboxamides, as traditional anticonvulsants, primarily function by blocking voltage-gated sodium channels, which not only mitigate seizures but also influence mood disorders through modulation of serotonin and dopamine. Recent studies have uncovered their anticancer properties, demonstrated by both in vitro and in vivo experiments. This review comprehensively examines dibenzazepine’s pharmacodynamics, pharmacokinetics, and clinical applications, focusing on their emerging role in oncology. By highlighting the anticancer mechanisms of action—including apoptosis induction, inhibition of HDAC, Wnt/β-Catenin signaling, and Voltage-gated sodium channels, we suggest further research to fully elucidate their therapeutic potential and application in cancer treatment.
Cancer remains one of the leading causes of mortality worldwide despite advances in conventional treatments such as surgery, chemotherapy, and radiation therapy. These standard modalities often have significant limitations, including drug resistance, off-target effects, and severe side effects, contributing to high mortality rates and poor quality of life for many patients (Yang et al., 2020; Tuck et al., 2023; Wills et al., 2024; Zolotykh et al., 2024). The development of drug resistance is one of the most intense challenges facing cancer treatment (Aroosa et al., 2023). Reasons for developing resistance include mutations in the drug target, reactivation of upstream or downstream signaling pathways, microenvironment-mediated resistance, intra-tumoral heterogeneity, and drug sensitivity (Ramos and Bentires-Alj, 2015). The high mortality rate, alongside increasing numbers of cases in recent years, highlights the importance of identifying novel therapeutic strategies that can overcome these barriers. Developing new anticancer drugs is complex, costly, and time-consuming, often requiring over a decade and billions of dollars before a new compound reaches clinical use (Juárez-López and Schcolnik-Cabrera, 2021). Drug repurposing—using existing drugs with established safety profiles for new therapeutic purposes—has emerged as a promising strategy to accelerate the availability of effective therapies. This approach allows researchers to bypass many early stages of drug development, reducing both time and cost (Lyne and Yamini, 2021; Malik et al., 2023). Thalidomide is a successful example of a repurposed candidate in cancer, initially designed for morning sickness in pregnancy, then got FDA approval for multiple myeloma (Gao et al., 2020). We have previously reported a few drugs with the potential for use in oncology, such as benzimidazoles, tetracyclines, and amino acetonitriles (Pourgholami et al., 2006; Pourgholami et al., 2013; Bahrami et al., 2021).
Dibenzazepine carboxamides, including carbamazepine (CBZ), oxcarbazepine (OXC), and eslicarbazepine (ESL), are well-known antiepileptic agents traditionally used to block voltage-gated sodium channels (VGSCs). In addition to their anticonvulsant properties, they are also effective in treating mood disorders, such as depression and bipolar disorder, by modulating neurotransmitter systems (Brodie, 2017; Lawthom et al., 2018; Maan et al., 2024). Recent studies have intriguingly revealed that dibenzazepine carboxamides possess potential anticancer properties, supported by both in vitro and in vivo evidence (Meng et al., 2011; Leslie et al., 2020; Zhao et al., 2020). Recent studies have shown that CBZ does not increase the risk of cancer in patients with epilepsy and even reduces it (Stritzelberger et al., 2021). For instance, our recent study demonstrated that eslicarbazepine induces apoptosis and cell cycle arrest in C6 glioma cells and significantly suppresses tumor growth in an intracranial rat model, underscoring its potential as an anticancer agent (Afsordeh et al., 2024). Furthermore, OXC and its metabolites have shown potent cytotoxic and genotoxic effects on human lymphocytes in culture media (Atlı Şekeroğlu et al., 2017).
This review aims to provide a comprehensive overview of dibenzazepine carboxamides’ pharmacodynamics, pharmacokinetics, and clinical applications, focusing on their emerging role in oncology. We will discuss the main molecular mechanisms underlying their anticancer activity, including apoptosis induction, deacetylases (HDACs) inhibition, suppression of Wnt/β-catenin signaling, and blockade of VGSCs. By highlighting these novel mechanisms, we advocate for further research to fully elucidate their therapeutic potential in cancer treatment and encourage their clinical evaluation as effective, repurposed anticancer agents.
The primary chemical structure of the dibenzazepines carboxamides family is composed of a dibenzazepine with a 5-carboxamide nucleus, as depicted in Figure 1 (Aledo-Serrano and Gil-Nagel, 2020). The first drug of the family, carbamazepine [CBZ, (5-carbamoyl-5H-dibenzo (b,f) azepine)], was synthesized by Walter Schindler a Swiss chemist in 1953 (Benes et al., 1999). Its structure is quite similar to tricyclic antidepressants such as imipramine (Al-Waili, 2000). CBZ was initially FDA-approved for trigeminal neuralgia in 1968 (Blom, 1962). Showing anticonvulsant properties in the early 1960s led to its approval for epilepsy in Switzerland and Great Britain in 1963 and later FDA-approved for partial or tonic-clonic seizures in 1974 (Bonduelle et al., 1964; Shorvon, 2009). After being in clinical use for several years, CBZ has shown some adverse effects, including hyponatremia, responsible for the commonly reported CBZ-induced adverse drug reactions (nausea, headache, and dizziness), diplopia, and skin rashes (Fricke-Galindo et al., 2018). Due to these adverse effects of carbamazepine (Al Khalili et al., 2023), OXC (OXC, 10,11 -dihydro- 10-0x0-carbamazepine) was developed, which is the 10 keto analog of CBZ (Shorvon, 2000). It is a lipophilic compound with low water solubility and the same mechanism of action as CBZ but with less severe side effects (Mazza et al., 2007; Garoufi et al., 2016). The drug received FDA approval for partial epilepsy treatment in 2000 (Preboth, 2000). However, OXC has a relatively short half-life and limited formulation options (Galiana et al., 2017). Following these revelations, esclicarbazepine [ESL [(S)-(--)-10-acetoxy-10,11-dihydro-5H-dibenz[b,f]azepine-5-carboxamide] was developed. ESL is the newest drug and third-generation of dibenzazepines, with FDA approval for the treatment of epilepsy granted in 2013 (Tambucci et al., 2016). ESL has a structural distinction from CBZ and OXC, which lies in the hydroxyl group in the 10, 11-position of the central dibenzoazepine ring instead of a keto group. This structural change resulted in distinct metabolism, pharmacokinetics, and pharmacodynamic advantages (Galiana et al., 2017). ESL does not metabolize to carbamazepine-10, 11-epoxide, the culprit of CBZ-induced adverse effects (Galiana et al., 2017). ESL has shown high efficacy and safety when used for epilepsy management (Almeida and Soares-da-Silva, 2007).
Figure 1. Chemical structure of dibenzazepine carboxamides .CBZ, OXC, and ESL all have a dibenzazepine nucleus bearing a 5-carboxamide substitute.
CBZ is predominantly metabolized in the liver, with less than 5% remaining unchanged (Kim et al., 2005). Cytochrome P450 subtype (CYP3A4) converts CBZ into its primary metabolite, carbamazepine-10,11-epoxide, which causes neurotoxicity (Aledo-Serrano and Gil-Nagel, 2020) and has anti-seizure effects like CBZ in animals (Bertilsson, 1978). About 15% of CBZ is metabolized to CBZ N-glucuronide by uridine diphosphate glucuronosyltransferase 2B7 (UGT2B7) (Fricke-Galindo et al., 2018). Another enzyme, myeloperoxidase (MPO), produces 2-hydroxycarbamazepine (2OH-CBZ), 3-hydroxycarbamazepine (3OH-CBZ), and 2,3 dihydroxycarbamazepine (Lu and Uetrecht, 2008). Approximately 75% of the drug is bound to plasma proteins (Bertilsson, 1978). After a single oral dose, the average half-life is 35 h, which decreases to 10–20 h after chronic use, leading to enzyme induction (Bertilsson, 1978). The maximum plasma concentration (Cmax) is reached or appears in 2–8 h (Spina et al., 1996), and the remainder of CBZ is excreted through urine (Bertilsson, 1978). CBZ passes through the placenta, and its plasma level decreases due to its high metabolism, making a relatively short plasma half-life in infants during the fetal period (Bertilsson, 1978). The most common adverse effects of CBZ use are dizziness, nausea, drowsiness, and weight gain (Koliqi et al., 2015). The side effects of this drug are usually mild in low doses, with severe side effects observed in high doses. Most patients treated with CBZ experience a decrease in the white blood cell count (Pellock, 1987; Delcker et al., 1997). In addition, the rate of change in the dose of CBZ can lead to psychomotor symptoms in the central nervous system (CNS), followed by gastrointestinal, hepatic, endocrine, and teratogenic side effects (Delcker et al., 1997; Tecoma, 1999). The second-generation carboxamide, OXC, is also metabolized by cytochrome P450 (CYP), CYP3A4, and CYP3A5 enzymes to 10, 11-dihydro-10-hydroxy-carbazepine (mono hydroxy derivative, MHD) in the liver (Schütz et al., 1986; May et al., 2003). After a single oral dose of OXC, peak concentrations (the highest level of medication in the blood) reach within approximately 1–3 h (May et al., 2003). The main route for OXC elimination is through renal excursion. The half-life of OXC has been reported to be between 1 and 5 h, whereas the half-life of MHD is up to 20 h. The MHD plasma protein binding rate is almost 40% (May et al., 2003). As the epoxide derived from CBZ is responsible for some of its toxic effects, the absence of this metabolite in OXC reduces the side effects (Shorvon, 2000). Studies have shown that symptoms such as nausea, digestive disorders, and ataxia may also occur with the use of OXC; however, the profile of side effects associated with the use of this drug is better than that of CBZ (Shorvon, 2000). OXC crosses the placenta in humans (May et al., 2003) and is not recommended for use during pregnancy (Shorvon, 2000). Owing to the structural change at positions 10 and 11, the third-generation drug, ESL, has a different metabolism. In the liver, enzyme CYP3A4 converts it into (S)-licarbazepine, oxcarbazepine (OXC), and (R)-licarbazepine, with the first enantiomer responsible for the most anticonvulsant activity of the drug through blockage of VGSCs (Almeida and Soares-da-Silva, 2007). The systemic amount of the ESL metabolite (eslicarbazepine) in the body after oral administration of ESL is 94% of the original dose (Bialer and Soares-da-Silva, 2012). ESL is more effective and has fewer side effects than the two earlier drugs, with the most adverse effects being non-serious (Ben-Menachem, 2010; Peltola et al., 2015; Guedes et al., 2023). Moreover, it also has additional benefits, including complete absorption after oral intake. Cytochrome P450 enzyme induction is much lower by ESL, and drug interactions are less prevalent (Shorvon, 2000). The drug decomposes with a half-life of 20–24 h (Almeida and Soares-da-Silva, 2007), and its binding rate to plasma proteins is less than 40% (Tambucci et al., 2016). ESL is also secreted 90% in urine (Almeida et al., 2009). Pharmacodynamic studies have shown that CBZ and OXC inactivate fast-voltage sodium channels, while ESL inactivates slow-voltage sodium channels (Lawthom et al., 2018). The pharmacokinetic and pharmacodynamic characteristics of the three drugs are compared in Table 1.
The primary actions of dibenzazepines include anticonvulsant properties via inactivation of VGSCs (Aledo-Serrano and Gil-Nagel, 2020). The effects of dibenzazepines, especially CBZ, on neurotransmitters such as GABA, glutamate, dopamine, and serotonin have also led to their use in the treatment of mood disorders (Wang, 2013; Shah et al., 2015; Ayano, 2016). CBZ also inhibits calcium and potassium channels (Grunze et al., 1998). The following sections will discuss the common uses of dibenzazepines in detail based on their mechanism of action.
Based on Na channel blocking abilities, dibenzazepines have anticonvulsant properties. Epilepsy is one of the most common diseases of the CNS that occurs with simultaneous abnormal activity in the function of brain neurons (Hu et al., 2023). With an approximate prevalence of 6 per 1,000 people (Brigo et al., 2022), it is one of the common public health challenges for physicians worldwide. More than 30 different drugs have been used to manage epilepsy (Hu et al., 2023). These drugs can improve the acute conditions of patients by influencing multiple molecular mechanisms (Rubio et al., 2023); among them, dibenzazepines, including CBZ, OXC, and ESL, are sodium channel blockers that treat epilepsy alone or in combination with other drugs (García-Peñas et al., 2020; Rocamora et al., 2020; Watkins et al., 2020; Nevitt et al., 2022). Through binding to the inactive state of the sodium channels in the membrane, CBZ slows down their reactivation. This delay in sodium channel reactivation reduces the chance of rhythmic propagation of action potentials and prevents the calcium entrance into synaptic membranes. Finally, synaptic function decreases with CBZ treatment, which reduces the excitability of neurons in the brain by increasing the release of GABA and decreasing glutamate release (Tolou-Ghamari et al., 2013). Figure 2 illustrates the anticonvulsant mechanism of dibenzazepines. The antiepileptic effects of OXC are the same as CBZ (Faught and Kim, 2015). The anticonvulsant effects of CBA and OXC have been demonstrated in animal studies of spontaneous seizures induced by kainite (Grabenstatter et al., 2007), pentylenetetrazol (PTZ) (Barzroodi Pour et al., 2021), and maximal electroshock (Tecoma, 1999; Zhen Sun et al., 2002). Double-blind studies have shown that oxcarbazepine use is associated with fewer side effects and better tolerance (Reinikainen et al., 1987). With the entry of the third-generation drug of this family (ESL) into the clinic, better seizure control and fewer side effects led to improved conditions for seizure control in epileptic patients (Mestre and Ferreira, 2009; Verrotti et al., 2014; Soares-da-Silva et al., 2015).
Figure 2. Main antiepileptic actions of dibenzazepines. 1: VGSCs of brain neurons become impermeable to sodium in the presence of dibenzazepines; 2: Hence, action potentials are blocked. 3: In addition, calcium entrance from synaptic space decreases. 4 and 5: Therefore, glutamate release and its effects on NMDA receptors are declined. 6: All these events reduce brain excitability. Voltage-gated Na+ channels: VGSCs, N-methyl-D-aspartate: NMDA. By Biorender.com.
It was later found that dibenzazepine carboxamides have other clinical uses, including mood stabilization (Maan et al., 2023). CBZ is used to treat bipolar disorder (Hirschfeld and Kasper, 2004), manic-depression (Ballenger and Post, 1980), attention-deficit hyperactivity disorder (ADHD) (Silva et al., 1996), and neuropathic pain (Buescher, 2006; Wiffen et al., 2014). OXC also showed promising effects on bipolar disorders in clinical studies (Centorrino et al., 2003; Benedetti et al., 2004). In patients with bipolar disorder, the level of GABA neurotransmitter decreases, which causes excitotoxicity and cell death through apoptosis. Chronic administration of CBZ as an agonist of GABA receptors α1, β2, and γ2 subunits can stabilize mood in these patients (Ayano, 2016). The mood-modifying effects of CBZ occur through five of the neurotransmitters in the brain, including A) Glutamate: CBZ has anti-glutamatergic effects by reducing its release and inhibiting calcium influx, which reduces the post-synaptic effects of glutamate (Kawata et al., 2001). B) Dopamine: dopamine is involved in the pathophysiology of bipolar disorder. CBZ indirectly affects dopaminergic receptors through the D2 receptor phosphorylation and density reduction (Kawata et al., 2001). C) Serotonin: like dopamine, serotonin affects the pathophysiology of bipolar disorder. CBZ controls this disease by increasing the release of serotonin and inhibiting its reabsorption (Dailey et al., 1998; Kawata et al., 2001). D) Norepinephrine: CBZ also reduces the level of norepinephrine, the most crucial neurotransmitter in bipolar disorder (Norman et al., 2009). However, the mood-stabilizing properties of CBZ are a matter of question as it has been shown that CBZ is a selective adrenaline A1 receptor antagonist and A2A receptor agonist (Van Calker et al., 1991; Okada et al., 2019). Activation of A2A receptors is attributed to depression-like symptoms, while increased A1 receptor signaling is associated with antidepressant effects (van Calker et al., 2019). Through antagonistic action on the A2A receptor, chronic administration of CBZ suppresses the activation of astroglial glutamatergic transmission induced by proinflammatory cytokines Interferon-gamma (IFNγ) and tumor necrosis factor-alpha (TNFα), implying the benefits of CBZ in the prevention of pathomechanisms development in several neuropsychiatric disorders, such as Niemann–Pick disease, schizophrenia, and autism (Okada et al., 2019). Other mechanisms include blocking cyclic adenosine monophosphate and G protein and increasing brain-derived neurotrophic factor (BDNF) (Ambrósio et al., 2002; Sadock et al., 2009; Ayano, 2016).
Another member of the family, OXC, has also been suggested as a potential treatment for ADHD in adults (Davids et al., 2006). Similar effects from OXC have been observed in controlling impulsive aggression in adults (Mattes, 2005). In this context, other studies have also suggested and demonstrated the mood-stabilizing effects of ESL (Popova et al., 2007; Nath et al., 2012). Moreover, its efficacy and safety in trigeminal neuralgia have been established (Sanchez-Larsen et al., 2018; Fortuna, 2022). Altogether, these data indicate the efficiency of carbamazepine and its derivatives in controlling mood disorders. Figure 3 shows the mood stabilization activities of dibenzazepines.
Figure 3. Summary of known actions of dibenzazepines in Mood stabilization. The effects of CBZ on mood regulation are through six mechanisms. Antiglutamatergic effects are through reducing glutamate release and calcium influx inhibition. The decrease in dopamine efficacy is induced by the decline in both the density and phosphorylation of D2 receptors. Moreover, these effects are shown by increasing the release of serotonin, decreasing the release of norepinephrine, antagonistic effects of adenosine on A2 receptors, and blocking the effects of cAMP and G proteins.
In addition to the aforementioned neurotherapeutic effects, these agents may also have anticancer properties. Over the past decades, some studies have provided encouraging results, suggesting that the pharmacological properties of dibenzazepines may interfere with cancerous tumor growth.
Dibenzazepines exert their anticancer effects through a variety of molecular mechanisms. These mechanisms include the induction of apoptosis and modulation of the cell cycle, autophagy induction, histone deacetylases (HDACs) inhibition, voltage-gated Na+ channels (VGSCs) inhibition, and interference with Wnt/β-catenin signaling. Dibenzazepines exhibit a multifaceted approach to cancer treatment by targeting multiple pathways, setting the stage for their potential use as anticancer agents. Understanding these pathways provides crucial insight into how these drugs may be repurposed for cancer therapy. The following section will explore the existing evidence for the anticancer effects of dibenzazepines, highlighting the leading studies demonstrating their efficacy in various cancer models.
Preliminary studies have shown the activation of apoptosis signaling in response to dibenzazepine exposure (Ota et al., 2021). Activation of apoptosis in platelets induces thrombocytopenia and bleeding in patients treated with CBZ. These pathways include decreased phosphorylation of the Bcl-xl/bcl-2-associated death promoter (BAD), vasodilator-stimulated-associated phosphoprotein (VASP), and glycoprotein 1b-beta (GPIbβ) in platelets, indicating an inhibitory effect on protein kinase A (PKA). In addition, PKA activity is reduced through PI3K/Akt/PDE3A signaling, resulting in apoptosis in platelets (Xiao et al., 2021). Through the activation of the Ras/Raf/ERK/p53 signaling pathway, CBZ induces hepatic DNA damage and mitochondrial apoptosis in Chinese rare minnows (Yan et al., 2021). After 60 days of daily oral CBZ intake (25 mg/kg) by Wistar-Albino rats, Caspase-3, PARP-1, and 8-hydroxy-2-deoxyguanosine immunoreactivity markedly increased, indicating apoptosis and oxidative stress as culprits of induced renal toxicity by CBZ (Erdem Guzel et al., 2023).
Administering CBZ and OXC on days 5–17 of pregnancy in rats leads to pro-apoptotic effects in the CA1, CA3, and dentate gyrus (DG) regions in the hippocampus of the offspring (González-Maciel et al., 2020). OXC-induced apoptosis occurs in the brain cells of adult and neonatal rats due to the activation of caspase-3 and Bax/Bcl-2 signaling (Song et al., 2018). Flow cytometric analysis showed that OXC caused the mitosis-phase cell cycle arrest and increased histone H3 phosphorylation, indicating cell mitosis. Also, it inhibited centrosome separation by reducing Polo-like kinase 1 (PLK1) activation and inducing apoptosis in NRK-52E cells via abnormal spindle formation (Ota et al., 2021). Cancer studies have demonstrated the induction of apoptosis by dibenzazepines. A recent in vitro study using MTT assay and AO/EB staining showed that CBZ induces apoptosis and cytotoxicity in the HT-29 human colon adenocarcinoma cell line (Sohaib and Ezhilarasan, 2020). In addition, immunofluorescence analysis showed that CBZ also increased caspase-3 activity, one of the key mediators in intrinsic apoptosis (Sohaib and Ezhilarasan, 2020). CBZ has protective and mitigating effects against ionizing irradiation in Murine Cells (Kim et al., 2012). Clinical observations using a wide range of anticonvulsants showed that among anticonvulsant drugs, CBZ significantly reduces the risk of skin and digestive cancers (Stritzelberger et al., 2021). OXC has anti-proliferative activity in HeLa and MCF7 cell lines and, to a lesser extent, in HepG2 (El Sharkawi et al., 2014). In human glioma cell lines with isocitrate dehydrogenase (IDH) mutations, OXC induces apoptosis with increased caspase 3/7 activity. OXC also inhibits cell growth and proliferation effects in vitro in glioma stem-like cells (Dao Trong et al., 2023). Recent studies have highlighted the potential anticancer effects of ESL, particularly in glioma models. ESL has been shown to induce apoptosis and promote G2/M cell cycle arrest in C6 glioma cells, suggesting it may be a promising candidate for drug repurposing in glioma treatment (Afsordeh et al., 2024).
These studies suggest a robust potential for dibenzoazepine carboxamides to activate apoptosis and exert anticancer effects. However, more precise studies, including well-designed preclinical and clinical trials, are needed to fully elucidate their therapeutic potential and optimize their use in oncology.
Autophagy is a cellular process in which damaged and abnormal cellular materials are removed by lysosomes. Autophagy has protective roles against many diseases (Debnath et al., 2023). However, the mechanism of autophagy in cancer treatment is controversial, and interventions to stimulate or inhibit autophagy in various cancers are proposed according to their underlying cause (Levy et al., 2017). HDAC inhibitor drugs such as CBZ can induce apoptosis and autophagy by inhibiting the PI3K-Akt-mTOR pathway (Aroosa et al., 2023). CBZ induces autophagy in liver cells by activating calpain, reducing Atg7 and Beclin-1 (Kim et al., 2013). SMARCA4 is a BRG1 protein-encoding gene identified as an oncogene in many cancers (Schiebler et al., 2015; Zhang et al., 2018). Recently, Shaykevich et al. have reported that CBZ lowers BRG1 protein and mRNA levels in KRAS-mutant colorectal cancer cells and upregulates it in KRAS-wild type ones. They also sought the ULK1 mRNA level (an initiator of autophagy) and found that CBZ decreased the ULK1 mRNA KRAS-mutated colorectal cancer cells at both 6 and 24 h. These results show that CBZ can inhibit ULK1 in KRAS-mutated cells, potentially leading to a reduction in autophagy and a subsequent decrease in SMARCA4 levels (Shaykevich et al., 2024). Also, at different concentrations, CBZ protected murine hematopoietic progenitor 32D cl 3 cells against radiation and induction of autophagy (Kim et al., 2011). Another study reported the protective effects of CBZ on radiation independent of autophagy (Kim et al., 2012). Although limited, based on these observations, this drug class seems to be an inducer of autophagy in some cells and conditions. This autophagy induction inevitably suggests an intriguing path for further research in this field and the autophagy-mediated treatment of various types of cancer.
Histone deacetylases are enzymes that remove acetyl groups from lysine residues of both histone and nonhistone proteins, leading to chromatin condensation and repression of gene expression (Seto and Yoshida, 2014), by which they affect a myriad of biological events such as cell cycle, differentiation, and apoptosis in cancer cells (Marks et al., 2000; Zhang and Zhong, 2014). There are three main groups of histone deacetylases: class I HDACs (1, 2, 3, and 8) are homologous to the yeast Rpd3 gene, ubiquitously expressed, and primarily located in the nucleus. Class II HDACs (4–10, except 8) are gene products that actively shuttle between the nucleus and the cytosol. Class III HDACs, such as Sir2, are protein deacetylases that appear to have targets other than histones (Marks et al., 2003). HDAC dysregulation contributes to tumor development and progression, and HDAC inhibitors are considered promising anticancer agents (Liang et al., 2023). Through epigenetic regulation of gene expression, HDAC inhibitors are crucial in inducing cancer cell cycle arrest, promoting differentiation, and triggering cell death. They also reduce angiogenesis and modulate the immune response (Eckschlager et al., 2017). Moreover, several laboratory and clinical studies have demonstrated the effectiveness of CBZ in inhibiting histone deacetylases in a cancer context. CBZ and its major metabolite CBZ-10, 11-epoxide inhibit HDAC (3 and 7) in the HepG2 liver carcinoma cell line (Beutler et al., 2005). CBZ causes degradation of the Her2 protein through proteosomes, and these effects are related to inhibiting HDAC in breast cancer (Meng et al., 2011). CBZ inhibits histone deacetylase type 6 in breast cancer by increasing alpha-tubulin acetylation (Beutler et al., 2005). Two of the most-known effects of CBZ, including mood stabilization and teratogenicity, are caused by histone deacetylase inhibitory (HDACi) properties (Phiel et al., 2001; Beutler et al., 2005).
Furthermore, it has been shown that CBZ decreases interleukin-6 and prostate-specific antigen (PSA) secretion in different human prostate cancer cell lines (Abdul and Hoosein, 2001). Treatment of the LNCaP cell line (prostate cancer) with CBZ and OXC reduces PSA levels. Also, chronic exposure to CBZ and OXC slows the transformation of pre-tumor cells into prostate cancer and reduces its risk (Stettner et al., 2012). In line with the preventive effects, a case-control study found that CBZ reduced the risk of advanced prostate cancer (Salminen et al., 2016). In addition, a new study in 2021 showed that CBZ users had a lower risk of advanced prostate cancer than other HDAC-inhibiting antiepileptic drugs, such as valproate and phenobarbital (Salminen et al., 2021). However, regarding the prognosis of this disease with dibenzazepines, Salminen et al. have reported no beneficial effect on the mortality caused by this cancer in the users of HDAC inhibitor antiepileptic drugs compared to other antiepileptic drugs (Salminen et al., 2022). These findings indicate that dibenzazepines can modulate cancer progression through HDAC inhibition.
Dibenzazepines primarily act through the VGSCs inhibition (Hebeisen et al., 2015). VGSCs are composed of nine α (Nav1.1-Nav1.9) and four β subunits (β1-4) (Goldin et al., 2000; Brackenbury and Djamgoz, 2006). Researchers have shown that these sodium channel functions are phagocytosis, endocytosis, secretion, proliferation, and differentiation in non-excitable cells (Xia et al., 2017). For example, in human colon cancer, the SCN5A gene, which encodes Nav1.5 isoform, plays a critical role in cell invasion (House et al., 2010). VGSCs are aberrantly expressed in multiple human cancers (Bian et al., 2023). Compared to normal brain tissue, Nav1.2 and Nav1.6 subunits are highly expressed in glioma tissue (Griffin et al., 2020). The protein expression of Nav1.3 and Nav1.6 subunits increases in low-grade astrocytoma (Guan et al., 2018). In addition, a study has reported the role of the Nav1.7 subunit in gastric cancer (Xia et al., 2016). In non-small cell lung cancer, this invasive pathway occurs in the Nav1.7 subunit through the epidermal growth factor (EGF) - signal-regulated and kinase 1/2 (ERK1/2) pathway (Campbell et al., 2013). Also, in human cervical cancer, overexpression of Nav1.5 has been implicated in cell invasion (Hernandez-Plata et al., 2012). A recent meta-analysis study confirmed that VGSCs promote prostate cancer cell metastasis and suggested targeting VGSCs as a treatment option (Yildirim-Kahriman, 2023). Recently, studies by Theresa et al., in 2020 showed that ESL inhibited both transient and persistent sodium currents, demonstrating, for the first time, the potent inhibitory effects of ESL on the Nav1.5 isoform. Many cancer cells express Nav1.5 and voltage-dependent sodium channels that enhance invasion and metastasis, and inhibiting these channels may be effective in cancer control (Leslie et al., 2020). In addition, another mechanism that can affect the metastasis and migration of cancer cells under the influence of these drugs is the process of inhibiting nuclear factor kappa B (NF-κB) activity CBZ, also significantly inactivates the motility proteins, including myosin light chain 2 (MLC2), myosin phosphatase 1 target subunit 1 (MYPT1), and focal adhesion kinase (FAK), which are necessary for lymph endothelial cell migration and can reduce metastasis in a three-dimensional model of breast cancer cell culture (Teichmann et al., 2014).
Considering the role and importance of VGSCs and their subunits in the pathophysiology of all types of cancer, especially cell invasion and metastasis, it is necessary to identify the mechanisms behind these processes. Based on these observations and reports, dibenzazepines are worthy of further research in this area of oncology.
Wnt (Wingless/Integrated) signaling is crucial in physiological processes such as embryonic development and adult homeostasis (Zhong and Virshup, 2020). Wnts are a broad family of glycoproteins that regulate multiple developmental pathways (Boyer et al., 2010; Grishanova et al., 2023). Wnt signaling occurs in two pathways. A) β-catenin dependent (canonical) or B) nondependent or non-canonical (Zhan et al., 2017). Dysregulation of this pathway is a hallmark of many cancers, where overactive Wnt signaling leads to increased β-catenin accumulation in the cytoplasm and its subsequent translocation to the nucleus. In the nucleus, β-catenin binds to T-cell factor/lymphoid enhancer factor (TCF/LEF) transcription factors, promoting the transcription of genes involved in cancer cell proliferation, invasion, and metastasis(Yu et al., 2020). Therefore, inhibiting this pathway can be one of the novel strategies to control many cancers (Chen et al., 2017; Yang et al., 2018; Rogaczewski et al., 2022). CBZ has been investigated as a Wnt/β-catenin signaling inhibitor in several studies (Parveen et al., 2018; Im et al., 2019; Bai et al., 2021).
In vitro, studies have shown the effectiveness of CBZ in human colon cancer cell lines (SW480) and suggested that this drug probably plays a role in reducing the levels of VEGF and β-catenin in this cell line (Akbarzadeh et al., 2016) and mouse adipocyte 3T3-L1 cells (Im et al., 2019). In addition, recently, surface plasmon resonance (SPR) studies showed that CBZ is a ligand for the FTZ receptor of Wnt/β-catenin signaling (Zhao et al., 2020), which specifically binds to a novel pocket on the Frizzled-8 (FZD8CRD), and does not overlap with the known Wnt binding sites, suggesting that CBZ may directly inhibit Wnt signaling by interfering with receptor-ligand interactions. FZD proteins, as essential Wnt receptors, are a central point for Wnt signaling intervention in diseases such as cancer (Zhong and Virshup, 2020). Figure 4. Shows the anticancer mechanisms of dibenzazepines.
Figure 4. Summary of known actions of dibenzazepines in cancer. Cancer cells exposed to dibenzazepines increase the ratio of Bax/Bcl2 ratio and caspase 3 and 7, induce G2-M cell cycle arrest, enhance histone H3 phosphorylation, and decrease the activity of PLK1, all leading to apoptotic signaling. Dibenzazepine’s action through VGSCs with unknown mechanisms leads to a decrease in the NF-kB activity, MLC2, MYPT1, and FAK lead to a reduction in metastasis of cancer cells treated with these drugs. Also, dibenzazepines reduce VEGF levels. Dibenzazepines also inhibit the deacetylation of chromatin by inhibiting histone deacetylases (3, 6, and 7) and suppress tumor growth. They also inhibit the Wnt/β-catenin pathway by binding to the FZ receptor and finally inhibit gene transcription. NF-kB: Nuclear factor kappa-light-chain-enhancer of activated B cells, PLK1: Polo-like kinase 1, MLC2: myosin light chain 2, MYPT1: myosin phosphatase 1 target subunit 1, FAK: focal adhesion kinase, LRP: Lipoprotein receptor-related proteins, FZ:N-terminal extra-cellular cysteine-rich domain of a Frizzled, TCF/LEF: T cell factor/lymphoid enhancer factor. By Biorender.com.
Seizures are well-known symptoms of early brain tumors and can occur at any point during the disease, considered a warning sign for the diagnosis or progression of brain tumors (Pignatti et al., 2002). The incidence of seizures is about 80% in patients with low-grade gliomas (LGGs) (Kurzwelly et al., 2010) and 60% in high-grade gliomas (HGGs) (van Breemen et al., 2007). Seizures in patients with LGGs are most resistant to drug treatments (Berntsson et al., 2018). The extensive use of antiepileptic drugs in brain tumors and their potential for cancer control made them highly desirable for both laboratory and clinical studies.
Patients with brain tumors and seizures who do not receive antiepileptic drugs have shorter survival rates (Cacho-Diaz et al., 2018). CBZ has similar effects to valproate in the survival of patients with GBM owing to its inhibitory effects on histone deacetylation (Guthrie and Eljamel, 2013). Carbamazepine, oxcarbazepine, and Eslicarbazepine are all FDA-approved drugs utilized clinically in epileptic seizures. This is a huge advantage to patients with GBM suffering from seizures.
In addition, in vitro tests on human glioma cell lines U-87 MG and T98G revealed the efficacy of OXC to be equal to temozolomide in MTT and cell cycle tests (Lee et al., 2016). CBZ also suppresses cell growth of A 172, AM 38, and YH 13 cell lines (Yagi et al., 2022). A cohort study showed the effectiveness of ESL on tumor-induced seizures in glioma patients (Zoccarato et al., 2021). The promising results of ESL in our recent findings of reduced tumor growth and induced apoptosis in glioma cells suggest that this drug warrants further investigation in clinical trials to assess its therapeutic potential in patients with brain tumors (Afsordeh et al., 2024). Table 2. Summarizes reported studies on dibenzazepines in cancer. As mentioned, one of the main symptoms associated with brain tumors is seizures. Due to the prominent role of dibenzazepines in controlling seizures and the positive results of preliminary studies in inhibiting the growth of brain cancer cell lines, dibenzazepines may be suitable candidates for further investigation to inhibit tumor growth in addition to seizure control. The promising outcomes from preclinical studies warrant further investigation into their mechanisms of action and therapeutic benefits in GBM as a priority.
Previous studies have shown that valproic acid, another antiepileptic drug, also has anti-cancer potential through tumor growth reduction and metastasis suppression (Blaheta and Cinatl, 2002). This drug, shares signaling pathway crosstalk with dibenzazepines. It inhibits WNT and histone deacetylases and has shown effectiveness in some in vitro and in vivo cancer studies(Duenas-Gonzalez et al., 2008). On the other hand, laboratory studies have shown that treatment with valproic acid, a histone deacetylase inhibitor, was beneficial in animals with diabetes-related colon cancer (Patel and Patel, 2018). This is while valproic acid, used to treat epilepsy have not shown a protective role against cancer (Hallas et al., 2009). Also, the use of valproic acid as a histone deacetylase inhibitor with a cumulative dose of 1,500 g over 5 years is not associated with a reduced risk of cancer (Blaheta et al., 2002). Given that valproic acid, like dibenzazepines, is a well-known drug in the treatment of epilepsy and bipolar disorders, and studies have shown that it can also be useful for controlling cancer, further studies in vitro and in systematic reviews are needed to compare these drugs to reach a definitive conclusion about their advantages and disadvantages. However, there is still much work to be done to understand the effectiveness of these drugs on cancer and reduce the suffering of patients.
Dibenzazepine carboxamides, first known as anticonvulsants and mood stabilizers, exhibit potential as multifunctional anticancer agents due to their ability to target multiple signaling pathways in cancer. This review has highlighted several of their promising mechanisms of action, including the induction of apoptosis, modulation of the cell cycle, and inhibition of key oncogenic pathways such as HDACs and Wnt/β-catenin signaling. However, the current body of evidence on the anticancer properties of dibenzazepines is limited, with most studies conducted at the preclinical level. The lack of clinical studies is a significant gap that needs to be marked to validate their therapeutic potential in oncology. Given their established safety profiles and known pharmacokinetics, repurposing dibenzazepines, particularly ESL, offers a strategic advantage by potentially bypassing the high costs and extended timelines associated with the new anticancer drug development. Future research should focus on further exploring these compounds in diverse cancer models, elucidating their precise mechanisms of action, optimizing dosing regimens, and advancing toward pilot clinical trials. There is a need for future studies to explore the pharmacokinetics and safety profiles of dibenzazepines in the context of cancer. Such efforts are essential a complete assessment of their anticancer capabilities. The transition from anticonvulsant use to cancer therapy exemplifies the innovative potential of drug repurposing and opens new horizons in the search for effective, multi-targeted cancer treatments.
NA: Writing–original draft. SP: Writing–review and editing. JM-Z: Writing–review and editing. MP: Conceptualization, Supervision, Writing–review and editing.
The author(s) declare that no financial support was received for the research and/or publication of this article.
The authors declare that the research was conducted in the absence of any commercial or financial relationships that could be construed as a potential conflict of interest.
The author(s) declare that no Generative AI was used in the creation of this manuscript.
All claims expressed in this article are solely those of the authors and do not necessarily represent those of their affiliated organizations, or those of the publisher, the editors and the reviewers. Any product that may be evaluated in this article, or claim that may be made by its manufacturer, is not guaranteed or endorsed by the publisher.
CBZ, carbamazepine; OXC, oxcarbazepine; ESL, eslicarbazepine; VGSCs, voltage-gated Na+ channels; CYP3A4, Cytochrome P450 subtype; UGT2B7, uridine diphosphate glucuronosyltransferase; MPO, myeloperoxidase; 2OH-CBZ, 2-hydroxycarbamazepine; 3OH-CBZ, 3-hydroxycarbamazepine; CYP, cytochrome P450; PTZ, pentylenetetrazol; ADHD, attention-deficit hyperactivity disorder; BDNF: brain-derived neurotrophic factor; IFNγ, Interferon-gamma; TNFα, tumur necrosis factor-alpha; VASP, vasodilator-stimulated-associated phosphoprotein; GPIbβ, glycoprotein 1b-beta; PKA, protein kinase A; PLK1, Polo-like kinase 1; IDH, isocitrate dehydrogenase; HDAC, histone deacetylases; PSA, prostate-specific antigen; EGF, epidermal growth factor; MLC2, myosin light chain 2; MYPT1, myosin phosphatase 1 target subunit 1; FAK, focal adhesion kinase; SPR, surface plasmon resonance; FZD, Frizzled; HGGs, high-grade gliomas; LGGs, low-grade gliomas.
Abdul, M., and Hoosein, N. (2001). Inhibition by anticonvulsants of prostate-specific antigen and interleukin-6 secretion by human prostate cancer cells. Anticancer Res. 21, 2045–2048.
Afsordeh, N., Pournajaf, S., Bayat, H., Mohajerani, F., Shojaei, A., Mirnajafi-Zadeh, J., et al. (2024). Eslicarbazepine induces apoptosis and cell cycle arrest in C6 glioma cells in vitro and suppresses tumor growth in an intracranial rat model. BMC Cancer 24, 1099. doi:10.1186/s12885-024-12840-3
Akbarzadeh, L., Moini Zanjani, T., and Sabetkasaei, M. (2016). Comparison of anticancer effects of carbamazepine and valproic acid. Iran. Red. Crescent Med. J. 18, e37230. doi:10.5812/ircmj.37230
Aledo-Serrano, A., and Gil-Nagel, A. (2020). Anticonvulsant agents: carbamazepine, oxcarbazepine, and eslicarbazepine acetate. NeuroPsychopharmacotherapy, 1–8.
Al Khalili, Y., Sekhon, S., and Jain, S. (2023). “Carbamazepine toxicity,” in StatPearls (Treasure Island FL: StatPearls Publishing).
Almeida, L., Bialer, M., and Soares-Da-Silva, P. (2009). “Eslicarbazepine acetate,” in The treatment of epilepsy, 485–498.
Almeida, L., and Soares-Da-Silva, P. (2007). Eslicarbazepine acetate (BIA 2-093). Neurotherapeutics 4, 88–96. doi:10.1016/j.nurt.2006.10.005
Al-Waili, N. S. (2000). Carbamazepine to treat primary nocturnal enuresis: double-blind study. Eur. J. Med. Res. 5, 40–44.
Ambrósio, A. F., Soares-Da-Silva, P., Carvalho, C. M., and Carvalho, A. P. (2002). Mechanisms of action of carbamazepine and its derivatives, oxcarbazepine, BIA 2-093, and BIA 2-024. Neurochem. Res. 27, 121–130. doi:10.1023/a:1014814924965
Aroosa, M., Malik, J. A., Ahmed, S., Bender, O., Ahemad, N., and Anwar, S. (2023). The evidence for repurposing anti-epileptic drugs to target cancer. Mol. Biol. Rep. 50, 7667–7680. doi:10.1007/s11033-023-08568-1
Atlı Şekeroğlu, Z., Kefelioğlu, H., Kontaş Yedier, S., Şekeroğlu, V., and Delmecioğlu, B. (2017). Oxcarbazepine-induced cytotoxicity and genotoxicity in human lymphocyte cultures with or without metabolic activation. Toxicol. Mech. Methods 27, 201–206. doi:10.1080/15376516.2016.1273430
Ayano, G. (2016). Bipolar disorders and carbamazepine: pharmacokinetics, pharmacodynamics, therapeutic effects and indications of carbamazepine: review of articles. J. Neuropsychopharmacol. Ment. Health 1, 2. doi:10.4172/2472-095x.1000112
Bahrami, F., Mekkawy, A. H., Badar, S., Morris, D. L., and Pourgholami, M. H. (2021). Monepantel antitumor activity is mediated through inhibition of major cell cycle and tumor growth signaling pathways. Am. J. Cancer Res. 11, 3098–3110.
Bai, Z., Jia, K., Chen, G., Liao, X., Cao, Z., Zhao, Y., et al. (2021). Carbamazepine induces hepatotoxicity in zebrafish by inhibition of the Wnt/β-catenin signaling pathway. Environ. Pollut. 276, 116688. doi:10.1016/j.envpol.2021.116688
Ballenger, J. C., and Post, R. M. (1980). Carbamazepine in manic-depressive illness: a new treatment. Am. J. psychiatry 137, 782–790. doi:10.1176/ajp.137.7.782
Barzroodi Pour, M., Bayat, M., Navazesh, A., Soleimani, M., and Karimzadeh, F. (2021). Exercise improved the anti-epileptic effect of carbamazepine through gaba enhancement in epileptic rats. Neurochem. Res. 46, 2112–2130. doi:10.1007/s11064-021-03349-3
Benedetti, A., Lattanzi, L., Pini, S., Musetti, L., Dell’osso, L., and Cassano, G. B. (2004). Oxcarbazepine as add-on treatment in patients with bipolar manic, mixed or depressive episode. J. Affect. Disord. 79, 273–277. doi:10.1016/S0165-0327(02)00407-X
Benes, J., Parada, A., Figueiredo, A. A., Alves, P. C., Freitas, A. P., Learmonth, D. A., et al. (1999). Anticonvulsant and sodium channel-blocking properties of novel 10,11-dihydro-5H-dibenz[b,f]azepine-5-carboxamide derivatives. J. Med. Chem. 42, 2582–2587. doi:10.1021/jm980627g
Ben-Menachem, E. (2010). Eslicarbazepine acetate: a well-kept secret? Epilepsy Curr. 10, 7–8. doi:10.1111/j.1535-7511.2009.01337.x
Berntsson, S. G., Merrell, R. T., Amirian, E. S., Armstrong, G. N., Lachance, D., Smits, A., et al. (2018). Glioma-related seizures in relation to histopathological subtypes: a report from the glioma international case-control study. J. Neurol. 265, 1432–1442. doi:10.1007/s00415-018-8857-0
Bertilsson, L. (1978). Clinical pharmacokinetics of carbamazepine. Clin. Pharmacokinet. 3, 128–143. doi:10.2165/00003088-197803020-00003
Beutler, A. S., Li, S., Nicol, R., and Walsh, M. J. (2005). Carbamazepine is an inhibitor of histone deacetylases. Life Sci. 76, 3107–3115. doi:10.1016/j.lfs.2005.01.003
Bialer, M., and Soares-Da-Silva, P. (2012). Pharmacokinetics and drug interactions of eslicarbazepine acetate. Epilepsia 53, 935–946. doi:10.1111/j.1528-1167.2012.03519.x
Bian, Y., Tuo, J., He, L., Li, W., Li, S., Chu, H., et al. (2023). Voltage-gated sodium channels in cancer and their specific inhibitors. Pathol. Res. Pract. 251, 154909. doi:10.1016/j.prp.2023.154909
Blaheta, R. A., and Cinatl, J. J. M. R. R. (2002). Anti-tumor mechanisms of valproate: a novel role for an old drug, Med. Res. Rev. 22, 492–511. doi:10.1002/med.10017
Blaheta, R. A., Nau, H., Michaelis, M., and Cinatl, J. (2002). Valproate and valproate-analogues: potent tools to fight against cancer, Curr. Med. Chem. 9, 1417–1433. doi:10.2174/0929867023369763
Blom, S. (1962). Trigeminal neuralgia: its treatment with a new anticonvulsant drug (G-32883). Lancet 1, 839–840. doi:10.1016/s0140-6736(62)91847-0
Bonduelle, M., Bouygues, P., Jolivet, B., and Sallou, C. (1964). Automatisms of long duration and epileptic fugues in adults. Ann. Med. Psychol. Paris. 122, 169–174.
Boyer, A., Goff, A. K., and Boerboom, D. (2010). WNT signaling in ovarian follicle biology and tumorigenesis. Trends Endocrinol. Metab. 21, 25–32. doi:10.1016/j.tem.2009.08.005
Brackenbury, W. J., and Djamgoz, M. B. (2006). Activity-dependent regulation of voltage-gated Na+ channel expression in Mat-LyLu rat prostate cancer cell line. J. Physiol. 573, 343–356. doi:10.1113/jphysiol.2006.106906
Brigo, F., Igwe, S. C., Bragazzi, N. L., and Lattanzi, S. (2022). Clonazepam monotherapy for treating people with newly diagnosed epilepsy. Cochrane Database Syst. Rev. 2, Cd013028. doi:10.1002/14651858.CD013028.pub3
Brodie, M. J. (2017). Sodium Channel blockers in the treatment of epilepsy. CNS Drugs 31, 527–534. doi:10.1007/s40263-017-0441-0
Buescher, J. J. (2006). Carbamazepine for acute and chronic pain, Am. Fam. Physician. 73, 1549–1550.
Cacho-Diaz, B., San-Juan, D., Salmeron, K., Boyzo, C., and Lorenzana-Mendoza, N. (2018). Choice of antiepileptic drugs affects the outcome in cancer patients with seizures. Clin. Transl. Oncol. 20, 1571–1576. doi:10.1007/s12094-018-1892-6
Campbell, T. M., Main, M. J., and Fitzgerald, E. M. (2013). Functional expression of the voltage-gated Na+-channel Nav1.7 is necessary for EGF-mediated invasion in human non-small cell lung cancer cells. J. Cell Sci. 126, 4939–4949. doi:10.1242/jcs.130013
Centorrino, F., Albert, M. J., Berry, J. M., Kelleher, J. P., Fellman, V., Line, G., et al. (2003). Oxcarbazepine: clinical experience with hospitalized psychiatric patients. Bipolar Disord. 5, 370–374. doi:10.1034/j.1399-5618.2003.00047.x
Chen, Y., Rao, X., Huang, K., Jiang, X., Wang, H., and Teng, L. (2017). FH535 inhibits proliferation and motility of colon cancer cells by targeting wnt/β-catenin signaling pathway. J. Cancer 8, 3142–3153. doi:10.7150/jca.19273
Dailey, J. W., Reith, M. E., Steidley, K. R., Milbrandt, J. C., and Jobe, P. C. (1998). Carbamazepine-induced release of serotonin from rat hippocampus in vitro. Epilepsia 39, 1054–1063. doi:10.1111/j.1528-1157.1998.tb01290.x
Dao Trong, P., Jungwirth, G., Unterberg, A., Herold-Mende, C., and Warta, R. (2023). The antiepileptic drug oxcarbazepine inhibits the growth of patient-derived isocitrate dehydrogenase mutant glioma stem-like cells. Cells 12, 1200. doi:10.3390/cells12081200
Davids, E., Kis, B., Specka, M., and Gastpar, M. (2006). A pilot clinical trial of oxcarbazepine in adults with attention-deficit hyperactivity disorder. Prog. Neuro-Psychopharmacology Biol. Psychiatry 30, 1033–1038. doi:10.1016/j.pnpbp.2006.03.035
Debnath, J., Gammoh, N., and Ryan, K. M. (2023). Autophagy and autophagy-related pathways in cancer. Nat. Rev. Mol. Cell Biol. 24, 560–575. doi:10.1038/s41580-023-00585-z
Delcker, A., Wilhelm, H., Timmann, D., and Diener, H. C. (1997). Side effects from increased doses of carbamazepine on neuropsychological and posturographic parameters of humans. Eur. Neuropsychopharmacol. 7, 213–218. doi:10.1016/s0924-977x(97)00406-9
Duenas-Gonzalez, A., Candelaria, M., Perez-Plascencia, C., Perez-Cardenas, E., De La Cruz-Hernandez, E., and Herrera, L.J. C. T. R. (2008). Valproic acid as epigenetic cancer drug: preclinical, clinical and transcriptional effects on solid tumors, Cancer Treat. Rev. 34, 206–222. doi:10.1016/j.ctrv.2007.11.003
Eckschlager, T., Plch, J., Stiborova, M., and Hrabeta, J. (2017). Histone deacetylase inhibitors as anticancer drugs. Int. J. Mol. Sci. 18, 1414. doi:10.3390/ijms18071414
El Sharkawi, F. Z., El Shemy, H. A., and Khaled, H. M. (2014). Possible anticancer activity of rosuvastatine, doxazosin, repaglinide and oxcarbazepin. Asian Pac J. Cancer Prev. 15, 199–203. doi:10.7314/apjcp.2014.15.1.199
Erdem Guzel, E., Kaya Tektemur, N., Tektemur, A., and Etem Önalan, E. (2023). Carbamazepine-induced renal toxicity may be associated with oxidative stress and apoptosis in male rat. Drug Chem. Toxicol. 46, 136–143. doi:10.1080/01480545.2021.2014859
Fortuna, G. (2022). Highest dose of eslicarbazepine for refractory secondary trigeminal neuralgia. Am. J. Ther. 10, 1097. doi:10.1097/MJT.0000000000001714
Fotherby, K. (1995). Levonorgestrel. Clinical pharmacokinetics. Clin. Pharmacokinet. 28, 203–215. doi:10.2165/00003088-199528030-00003
Fricke-Galindo, I., Llerena, A., Jung-Cook, H., and López-López, M. (2018). Carbamazepine adverse drug reactions. Expert Rev. Clin. Pharmacol. 11, 705–718. doi:10.1080/17512433.2018.1486707
Galiana, G. L., Gauthier, A. C., and Mattson, R. H. (2017). Eslicarbazepine acetate: a new improvement on a classic drug family for the treatment of partial-onset seizures. Drugs R. D. 17, 329–339. doi:10.1007/s40268-017-0197-5
Gao, S., Wang, S., Fan, R., and Hu, J. (2020). Recent advances in the molecular mechanism of thalidomide teratogenicity. Biomed. Pharmacother. 127, 110114. doi:10.1016/j.biopha.2020.110114
García-Peñas, J. J., Smeyers-Durá, P., Roldán-Aparicio, S., and Aparicio, J. (2020). The role of eslicarbazepine acetate in the treatment of focal-onset epilepsy in pediatric age: practical issues. Rev. Neurol. 71, S1–S17. doi:10.33588/rn.71s01.2020562
Garoufi, A., Vartzelis, G., Tsentidis, C., Attilakos, A., Koemtzidou, E., Kossiva, L., et al. (2016). Weight gain in children on oxcarbazepine monotherapy. Epilepsy Res. 122, 110–113. doi:10.1016/j.eplepsyres.2016.03.004
Goldin, A. L., Barchi, R. L., Caldwell, J. H., Hofmann, F., Howe, J. R., Hunter, J. C., et al. (2000). Nomenclature of voltage-gated sodium channels. Neuron 28, 365–368. doi:10.1016/s0896-6273(00)00116-1
González-Maciel, A., Romero-Velázquez, R. M., Alfaro-Rodríguez, A., Sanchez Aparicio, P., and Reynoso-Robles, R. (2020). Prenatal exposure to oxcarbazepine increases hippocampal apoptosis in rat offspring. J. Chem. Neuroanat. 103, 101729. doi:10.1016/j.jchemneu.2019.101729
Grabenstatter, H. L., Clark, S., and Dudek, F. E. (2007). Anticonvulsant effects of carbamazepine on spontaneous seizures in rats with kainate-induced epilepsy: comparison of intraperitoneal injections with drug-in-food protocols. Epilepsia 48, 2287–2295. doi:10.1111/j.1528-1167.2007.01263.x
Griffin, M., Khan, R., Basu, S., and Smith, S. (2020). Ion channels as therapeutic targets in high grade gliomas. Cancers (Basel) 12, 3068. doi:10.3390/cancers12103068
Grishanova, A. Y., Klyushova, L. S., and Perepechaeva, M. L. (2023). AhR and wnt/β-catenin signaling pathways and their interplay. Curr. Issues Mol. Biol. 45, 3848–3876. doi:10.3390/cimb45050248
Grunze, H., Von Wegerer, J., Greene, R., and Walden, J. (1998). Modulation of calcium and potassium currents by lamotrigine. Neuropsychobiology 38, 131–138. doi:10.1159/000026528
Guan, G., Zhao, M., Xu, X., Boczek, T., Mao, X., Li, Z., et al. (2018). Abnormal changes in voltage-gated sodium channels subtypes Na(V)1.1, Na(V)1.2, Na(V)1.3, Na(V)1.6 and CaM/CaMKII pathway in low-grade astrocytoma. Neurosci. Lett. 674, 148–155. doi:10.1016/j.neulet.2018.03.047
Guedes, L., Vieira, M., Gama, H., Magano, D., Fernandes, M., Calero, P., et al. (2023). Thirteen years of experience with eslicarbazepine acetate in the United Kingdom and Republic of Ireland: a safety perspective. Epileptic Disord. 25, 803–814. doi:10.1002/epd2.20146
Guthrie, G. D., and Eljamel, S. (2013). Impact of particular antiepileptic drugs on the survival of patients with glioblastoma multiforme. J. Neurosurg. 118, 859–865. doi:10.3171/2012.10.JNS12169
Hallas, J., Friis, S., Bjerrum, L., Støvring, H., Narverud, S. F., Heyerdahl, T., et al. (2009). Cancer risk in long-term users of valproate: a population-based case-control study, Cancer Epidemiol. Biomarkers Prev. 18, 1714–1719. doi:10.1158/1055-9965.EPI-08-0646
Hebeisen, S., Pires, N., Loureiro, A. I., Bonifácio, M. J., Palma, N., Whyment, A., et al. (2015). Eslicarbazepine and the enhancement of slow inactivation of voltage-gated sodium channels: a comparison with carbamazepine, oxcarbazepine and lacosamide. Neuropharmacology 89, 122–135. doi:10.1016/j.neuropharm.2014.09.008
Hernandez-Plata, E., Ortiz, C. S., Marquina-Castillo, B., Medina-Martinez, I., Alfaro, A., Berumen, J., et al. (2012). Overexpression of NaV 1.6 channels is associated with the invasion capacity of human cervical cancer. Int. J. Cancer 130, 2013–2023. doi:10.1002/ijc.26210
Hirschfeld, R. M., and Kasper, S. (2004). A review of the evidence for carbamazepine and oxcarbazepine in the treatment of bipolar disorder. Int. J. Neuropsychopharmacol. 7, 507–522. doi:10.1017/S1461145704004651
House, C. D., Vaske, C. J., Schwartz, A. M., Obias, V., Frank, B., Luu, T., et al. (2010). Voltage-gated Na+ channel SCN5A is a key regulator of a gene transcriptional network that controls colon cancer invasion. Cancer Res. 70, 6957–6967. doi:10.1158/0008-5472.CAN-10-1169
Shah, J. H., Hindupur, R. M., and N Pati, H. (2015). Pharmacological and biological activities of benzazepines: an overview. Curr. Bioact. Compd. 11, 170–188. doi:10.2174/1573407211666150910202200
Hu, T., Zhang, J., Wang, J., Sha, L., Xia, Y., Ortyl, T. C., et al. (2023). Advances in epilepsy: mechanisms, clinical trials, and drug therapies. J. Med. Chem. 66, 4434–4467. doi:10.1021/acs.jmedchem.2c01975
Im, D. U., Kim, S. C., Chau, G. C., and Um, S. H. (2019). Carbamazepine enhances adipogenesis by inhibiting wnt/β-catenin expression. Cells 8, 1460. doi:10.3390/cells8111460
Juárez-López, D., and Schcolnik-Cabrera, A. (2021). Drug repurposing: considerations to surpass while Re-directing old compounds for new treatments. Arch. Med. Res. 52, 243–251. doi:10.1016/j.arcmed.2020.10.021
Kawata, Y., Okada, M., Murakami, T., Kamata, A., Zhu, G., and Kaneko, S. (2001). Pharmacological discrimination between effects of carbamazepine on hippocampal basal, Ca2+-and K+-evoked serotonin release. Br. J. Pharmacol. 133, 557–567. doi:10.1038/sj.bjp.0704104
Kim, H., Bernard, M. E., Farkas, A., Goff, J., Kalash, R., Houghton, F., et al. (2012). Ionizing irradiation protection and mitigation of murine cells by carbamazepine is p53 and autophagy independent. Vivo 26, 341–354. doi:10.21873/invivo.6614
Kim, H., Bernard, M. E., Flickinger, J., Epperly, M. W., Wang, H., Dixon, T. M., et al. (2011). The autophagy-inducing drug carbamazepine is a radiation protector and mitigator. Int. J. Radiat. Biol. 87, 1052–1060. doi:10.3109/09553002.2011.587860
Kim, J. S., Wang, J. H., Biel, T. G., Kim, D. S., Flores-Toro, J. A., Vijayvargiya, R., et al. (2013). Carbamazepine suppresses calpain-mediated autophagy impairment after ischemia/reperfusion in mouse livers. Toxicol. Appl. Pharmacol. 273, 600–610. doi:10.1016/j.taap.2013.10.006
Kim, K. A., Oh, S. O., Park, P. W., and Park, J. Y. (2005). Effect of probenecid on the pharmacokinetics of carbamazepine in healthy subjects. Eur. J. Clin. Pharmacol. 61, 275–280. doi:10.1007/s00228-005-0940-7
Koliqi, R., Polidori, C., and Islami, H. (2015). Prevalence of side effects treatment with carbamazepine and other antiepileptics in patients with epilepsy. Mater Sociomed. 27, 167–171. doi:10.5455/msm.2015.27.167-171
Kurzwelly, D., Herrlinger, U., and Simon, M. (2010). Seizures in patients with low-grade gliomas--incidence, pathogenesis, surgical management, and pharmacotherapy. Adv. Tech. Stand Neurosurg. 35, 81–111. doi:10.1007/978-3-211-99481-8_4
Lawthom, C., Peltola, J., Mcmurray, R., Dodd, E., and Villanueva, V. (2018). Dibenzazepine agents in epilepsy: How does eslicarbazepine acetate differ? Neurol. Ther. 7, 195–206. doi:10.1007/s40120-018-0111-2
Lee, C. Y., Lai, H. Y., Chiu, A., Chan, S. H., Hsiao, L. P., and Lee, S. T. (2016). The effects of antiepileptic drugs on the growth of glioblastoma cell lines. J. Neurooncol 127, 445–453. doi:10.1007/s11060-016-2056-6
Leslie, T. K., Brückner, L., Chawla, S., and Brackenbury, W. J. (2020). Inhibitory effect of eslicarbazepine acetate and S-licarbazepine on Na(v)1.5 channels. Front. Pharmacol. 11, 555047. doi:10.3389/fphar.2020.555047
Levy, J. M. M., Towers, C. G., and Thorburn, A. (2017). Targeting autophagy in cancer. Nat. Rev. Cancer 17, 528–542. doi:10.1038/nrc.2017.53
Liang, T., Wang, F., Elhassan, R. M., Cheng, Y., Tang, X., Chen, W., et al. (2023). Targeting histone deacetylases for cancer therapy: trends and challenges. Acta Pharm. Sin. B 13, 2425–2463. doi:10.1016/j.apsb.2023.02.007
Lu, W., and Uetrecht, J. P. (2008). Peroxidase-mediated bioactivation of hydroxylated metabolites of carbamazepine and phenytoin. Drug Metab. Dispos. 36, 1624–1636. doi:10.1124/dmd.107.019554
Lyne, S. B., and Yamini, B. (2021). An alternative pipeline for glioblastoma therapeutics: a systematic review of drug repurposing in glioblastoma. Cancers (Basel) 13, 1953. doi:10.3390/cancers13081953
Maan, J. S., Duong, T. V. H., and Saadabadi, A. (2023). “Carbamazepine,” in StatPearls (Treasure Island (FL): StatPearls Publishing).
Maan, J. S., Duong, T. V. H., and Saadabadi, A. (2024). “Carbamazepine, in StatPearls. Treasure island (FL) ineligible companies,” in Disclosure: truc vi Duong declares no relevant financial relationships with ineligible companies. Disclosure: abdolreza Saadabadi declares no relevant financial relationships with ineligible companies. StatPearls Publishing.
Malik, J. A., Ahmed, S., Momin, S. S., Shaikh, S., Alafnan, A., Alanazi, J., et al. (2023). Drug repurposing: a new hope in drug discovery for prostate cancer. ACS Omega 8, 56–73. doi:10.1021/acsomega.2c05821
Marks, P. A., Miller, T., and Richon, V. M. (2003). Histone deacetylases. Curr. Opin. Pharmacol. 3, 344–351. doi:10.1016/s1471-4892(03)00084-5
Marks, P. A., Richon, V. M., and Rifkind, R. A. (2000). Histone deacetylase inhibitors: inducers of differentiation or apoptosis of transformed cells. J. Natl. Cancer Inst. 92, 1210–1216. doi:10.1093/jnci/92.15.1210
Mattes, J. A. (2005). Oxcarbazepine in patients with impulsive aggression: a double-blind, placebo-controlled trial. J. Clin. Psychopharmacol. 25, 575–579. doi:10.1097/01.jcp.0000186739.22395.6b
May, T. W., Korn-Merker, E., and Rambeck, B. (2003). Clinical pharmacokinetics of oxcarbazepine. Clin. Pharmacokinet. 42, 1023–1042. doi:10.2165/00003088-200342120-00002
Mazza, M., Di Nicola, M., Martinotti, G., Taranto, C., Pozzi, G., Conte, G., et al. (2007). Oxcarbazepine in bipolar disorder: a critical review of the literature. Expert Opin. Pharmacother. 8, 649–656. doi:10.1517/14656566.8.5.649
Meng, Q., Chen, X., Sun, L., Zhao, C., Sui, G., and Cai, L. (2011). Carbamazepine promotes Her-2 protein degradation in breast cancer cells by modulating HDAC6 activity and acetylation of Hsp90. Mol. Cell Biochem. 348, 165–171. doi:10.1007/s11010-010-0651-y
Mestre, T., and Ferreira, J. (2009). Eslicarbazepine acetate: a new option for the treatment of focal epilepsy. Expert Opin. Investig. Drugs 18, 221–229. doi:10.1517/13543780802635107
Nath, K., Bhattacharya, A., and Praharaj, S. K. (2012). Eslicarbazepine acetate in the management of refractory bipolar disorder. Clin. Neuropharmacol. 35, 295. doi:10.1097/WNF.0b013e318271220b
Nevitt, S. J., Sudell, M., Cividini, S., Marson, A. G., and Tudur Smith, C. (2022). Antiepileptic drug monotherapy for epilepsy: a network meta-analysis of individual participant data. Cochrane Database Syst. Rev. 4, Cd011412. doi:10.1002/14651858.CD011412.pub4
Norman, S., and Kaplan, M. (2009). Sadock’s Comprehensive textbook of psychiatry. 9th edn. Editor B. J. Sadock, V. A. Sadock, and P. Ruiz (Philadelphia, PA: Wolters Kluwer/Lippincott Williams & Wilkins), 1139–1151.
Okada, M., Fukuyama, K., Shiroyama, T., and Ueda, Y. (2019). Carbamazepine attenuates astroglial L-glutamate release induced by pro-inflammatory cytokines via chronically activation of adenosine A(2A) receptor. Int. J. Mol. Sci. 20, 3727. doi:10.3390/ijms20153727
Ota, M., Funakoshi, T., Aki, T., Unuma, K., and Uemura, K. (2021). Oxcarbazepine induces mitotic catastrophe and apoptosis in NRK-52E proximal tubular cells. Toxicol. Lett. 350, 240–248. doi:10.1016/j.toxlet.2021.07.018
Parveen, B., Tiwari, A. K., Jain, M., Pal, S., Chattopadhyay, N., Tripathi, M., et al. (2018). The anti-epileptic drugs valproate, carbamazepine and levetiracetam cause bone loss and modulate Wnt inhibitors in normal and ovariectomised rats. Bone 113, 57–67. doi:10.1016/j.bone.2018.05.011
Patel, M. M., and Patel, B. M. J. E. J. O. P. S. (2018). Repurposing of sodium valproate in colon cancer associated with diabetes mellitus: role of HDAC inhibition, Eur. J. Pharm. Sci. 121, 188–199. doi:10.1016/j.ejps.2018.05.026
Pearce, R. E., Lu, W., Wang, Y., Uetrecht, J. P., Correia, M. A., and Leeder, J. S. (2008). Pathways of carbamazepine bioactivation in vitro. III. The role of human cytochrome P450 enzymes in the formation of 2,3-dihydroxycarbamazepine. Drug Metab. Dispos. 36, 1637–1649. doi:10.1124/dmd.107.019562
Pearce, R. E., Vakkalagadda, G. R., and Leeder, J. S. (2002). Pathways of carbamazepine bioactivation in vitro I. Characterization of human cytochromes P450 responsible for the formation of 2- and 3-hydroxylated metabolites. Drug Metab. Dispos. 30, 1170–1179. doi:10.1124/dmd.30.11.1170
Pellock, J. M. (1987). Carbamazepine side effects in children and adults. Epilepsia 28, S64–S70. doi:10.1111/j.1528-1157.1987.tb05780.x
Peltola, J., Holtkamp, M., Rocamora, R., Ryvlin, P., Sieradzan, K., and Villanueva, V. (2015). Practical guidance and considerations for transitioning patients from oxcarbazepine or carbamazepine to eslicarbazepine acetate--Expert opinion. Epilepsy Behav. 50, 46–49. doi:10.1016/j.yebeh.2015.05.036
Phiel, C. J., Zhang, F., Huang, E. Y., Guenther, M. G., Lazar, M. A., and Klein, P. S. (2001). Histone deacetylase is a direct target of valproic acid, a potent anticonvulsant, mood stabilizer, and teratogen. J. Biol. Chem. 276, 36734–36741. doi:10.1074/jbc.M101287200
Pignatti, F., Van Den Bent, M., Curran, D., Debruyne, C., Sylvester, R., Therasse, P., et al. (2002). Prognostic factors for survival in adult patients with cerebral low-grade glioma. J. Clin. Oncol. 20, 2076–2084. doi:10.1200/JCO.2002.08.121
Popova, E., Leighton, C., Bernabarre, A., Bernardo, M., and Vieta, E. (2007). Oxcarbazepine in the treatment of bipolar and schizoaffective disorders. Expert Rev. Neurother. 7, 617–626. doi:10.1586/14737175.7.6.617
Pourgholami, M. H., Ataie-Kachoie, P., Badar, S., and Morris, D. L. (2013). Minocycline inhibits malignant ascites of ovarian cancer through targeting multiple signaling pathways. Gynecol. Oncol. 129, 113–119. doi:10.1016/j.ygyno.2012.12.031
Pourgholami, M. H., Yan Cai, Z., Lu, Y., Wang, L., and Morris, D. L. (2006). Albendazole: a potent inhibitor of vascular endothelial growth factor and malignant ascites formation in OVCAR-3 tumor-bearing nude mice. Clin. Cancer Res. 12, 1928–1935. doi:10.1158/1078-0432.CCR-05-1181
Ramos, P., and Bentires-Alj, M. (2015). Mechanism-based cancer therapy: resistance to therapy, therapy for resistance. Oncogene 34, 3617–3626. doi:10.1038/onc.2014.314
Reinikainen, K. J., Keranen, T., Halonen, T., Komulainen, H., and Riekkinen, P. J. (1987). Comparison of oxcarbazepine and carbamazepine: a double-blind study. Epilepsy Res. 1, 284–289. doi:10.1016/0920-1211(87)90003-9
Rocamora, R., Peltola, J., Assenza, G., Mcmurray, R., and Villanueva, V. (2020). Safety, tolerability and effectiveness of transition to eslicarbazepine acetate from carbamazepine or oxcarbazepine in clinical practice. Seizure 75, 121–128. doi:10.1016/j.seizure.2019.12.022
Rogaczewski, P., Janiak, M., Borysewicz, K., Głos, T., Ahmadi, N., and Szylberg, Ł. (2022). Clinical significancy of WNT pathway inhibition in various cancers. J. Educ. Health Sport 12, 183–191. doi:10.12775/jehs.2022.12.11.024
Rubio, C., Gatica, F., Uribe, E., Ochoa, E., Campos, V., and Rubio-Osornio, M. (2023). Molecular and genetic mechanisms of neurotoxicity during anti-seizure medications use. Rev. Invest Clin. 75, 1–12. doi:10.24875/RIC.22000260
Sadock, B., Sadock, V., and Ruiz, P. (2009). Compre-hensive textbook of psychiatry. 9th edn. Lippincott Williams Wilkins. Phila-delphia.
Salminen, J. K., Kuoppamäki, V., Talala, K., Taari, K., Mäkinen, J., Peltola, J., et al. (2021). Antiepileptic drugs and prostate cancer risk in the Finnish randomized study of screening for prostate cancer. Int. J. Cancer 149, 307–315. doi:10.1002/ijc.33535
Salminen, J. K., Mehtola, A., Talala, K., Taari, K., Mäkinen, J., Peltola, J., et al. (2022). Anti-epileptic drugs and prostate cancer-specific mortality compared to non-users of anti-epileptic drugs in the Finnish Randomized Study of Screening for Prostate Cancer. Br. J. Cancer 127, 704–711. doi:10.1038/s41416-022-01817-3
Salminen, J. K., Tammela, T. L., Auvinen, A., and Murtola, T. J. (2016). Antiepileptic drugs with histone deacetylase inhibition activity and prostate cancer risk: a population-based case-control study. Cancer Causes Control 27, 637–645. doi:10.1007/s10552-016-0737-2
Sanchez-Larsen, A., Sopelana, D., Diaz-Maroto, I., Perona-Moratalla, A., Gracia-Gil, J., García-Muñozguren, S., et al. (2018). Assessment of efficacy and safety of eslicarbazepine acetate for the treatment of trigeminal neuralgia. Eur. J. pain 22, 1080–1087. doi:10.1002/ejp.1192
Schiebler, M., Brown, K., Hegyi, K., Newton, S. M., Renna, M., Hepburn, L., et al. (2015). Functional drug screening reveals anticonvulsants as enhancers of mTOR-independent autophagic killing of Mycobacterium tuberculosis through inositol depletion. EMBO Mol. Med. 7, 127–139. doi:10.15252/emmm.201404137
Schütz, H., Feldmann, K. F., Faigle, J. W., Kriemler, H. P., and Winkler, T. (1986). The metabolism of 14C-oxcarbazepine in man. Xenobiotica 16, 769–778. doi:10.3109/00498258609043567
Seto, E., and Yoshida, M. (2014). Erasers of histone acetylation: the histone deacetylase enzymes. Cold Spring Harb. Perspect. Biol. 6, a018713. doi:10.1101/cshperspect.a018713
Shaykevich, A., Chae, D., Silverman, I., Bassali, J., Louloueian, N., Siegman, A., et al. (2024). Impact of carbamazepine on SMARCA4 (BRG1) expression in colorectal cancer: modulation by KRAS mutation status. Invest New Drugs 42, 229–239. doi:10.1007/s10637-024-01418-2
Shorvon, S. D. (2009). Drug treatment of epilepsy in the century of the ILAE: the second 50 years, 1959-2009. Epilepsia 50 (Suppl. 3), 93–130. doi:10.1111/j.1528-1167.2009.02042.x
Silva, R. R., Munoz, D. M., and Alpert, M. (1996). Carbamazepine use in children and adolescents with features of attention-deficit hyperactivity disorder: a meta-analysis. J. Am. Acad. Child and Adolesc. Psychiatry 35, 352–358. doi:10.1097/00004583-199603000-00017
Soares-Da-Silva, P., Pires, N., Bonifácio, M. J., Loureiro, A. I., Palma, N., and Wright, L. C. (2015). Eslicarbazepine acetate for the treatment of focal epilepsy: an update on its proposed mechanisms of action. Pharmacol. Res. and Perspect. 3, e00124. doi:10.1002/prp2.124
Sohaib, M., and Ezhilarasan, D. (2020). Carbamazepine, a histone deacetylase inhibitor induces apoptosis in human colon adenocarcinoma cell line HT-29. J. Gastrointest. Cancer 51, 564–570. doi:10.1007/s12029-019-00286-x
Song, Y., Zhong, M., and Cai, F. C. (2018). Oxcarbazepine causes neurocyte apoptosis and developing brain damage by triggering Bax/Bcl-2 signaling pathway mediated caspase 3 activation in neonatal rats. Eur. Rev. Med. Pharmacol. Sci. 22, 250–261. doi:10.26355/eurrev_201801_14126
Spina, E., Pisani, F., and Perucca, E. (1996). Clinically significant pharmacokinetic drug interactions with carbamazepine. An update. Clin. Pharmacokinet. 31, 198–214. doi:10.2165/00003088-199631030-00004
Staines, A. G., Coughtrie, M. W., and Burchell, B. (2004). N-glucuronidation of carbamazepine in human tissues is mediated by UGT2B7. J. Pharmacol. Exp. Ther. 311, 1131–1137. doi:10.1124/jpet.104.073114
Stettner, M., Krämer, G., Strauss, A., Kvitkina, T., Ohle, S., Kieseier, B. C., et al. (2012). Long-term antiepileptic treatment with histone deacetylase inhibitors may reduce the risk of prostate cancer. Eur. J. Cancer Prev. 21, 55–64. doi:10.1097/CEJ.0b013e32834a7e6f
Stritzelberger, J., Lang, J. D., Mueller, T. M., Reindl, C., Westermayer, V., Kostev, K., et al. (2021). Anti-seizure medication is not associated with an increased risk to develop cancer in epilepsy patients. J. Neurol. 268, 2185–2191. doi:10.1007/s00415-020-10379-4
Tambucci, R., Basti, C., Maresca, M., Coppola, G., and Verrotti, A. (2016). Update on the role of eslicarbazepine acetate in the treatment of partial-onset epilepsy. Neuropsychiatric Dis. Treat. 12, 1251–1260. doi:10.2147/NDT.S86765
Teichmann, M., Kretschy, N., Kopf, S., Jarukamjorn, K., Atanasov, A. G., Viola, K., et al. (2014). Inhibition of tumour spheroid-induced prometastatic intravasation gates in the lymph endothelial cell barrier by carbamazepine: drug testing in a 3D model. Arch. Toxicol. 88, 691–699. doi:10.1007/s00204-013-1183-5
Tolou-Ghamari, Z., Zare, M., Habibabadi, J. M., and Najafi, M. R. (2013). A quick review of carbamazepine pharmacokinetics in epilepsy from 1953 to 2012. J. Res. Med. Sci. 18, S81–S85.
Tuck, C. Z., Cooper, R., Aryeetey, R., Gray, L. A., and Akparibo, R. (2023). A critical review and analysis of the context, current burden, and application of policy to improve cancer equity in Ghana. Int. J. Equity Health 22, 254. doi:10.1186/s12939-023-02067-2
Van Breemen, M. S., Wilms, E. B., and Vecht, C. J. (2007). Epilepsy in patients with brain tumours: epidemiology, mechanisms, and management. Lancet Neurol. 6, 421–430. doi:10.1016/S1474-4422(07)70103-5
Van Calker, D., Biber, K., Domschke, K., and Serchov, T. (2019). The role of adenosine receptors in mood and anxiety disorders. J. Neurochem. 151, 11–27. doi:10.1111/jnc.14841
Van Calker, D., Steber, R., Klotz, K. N., and Greil, W. (1991). Carbamazepine distinguishes between adenosine receptors that mediate different second messenger responses. Eur. J. Pharmacol. 206, 285–290. doi:10.1016/0922-4106(91)90111-t
Verrotti, A., Loiacono, G., Rossi, A., and Zaccara, G. (2014). Eslicarbazepine acetate: an update on efficacy and safety in epilepsy. Epilepsy Res. 108, 1–10. doi:10.1016/j.eplepsyres.2013.10.005
Watkins, L., O'dwyer, M., Oak, K., Lawthom, C., Maguire, M., Thomas, R., et al. (2020). The evidence for switching dibenzazepines in people with epilepsy. Acta Neurol. Scand. 142, 121–130. doi:10.1111/ane.13248
Wiffen, P. J., Derry, S., Moore, R. A., and Kalso, E. A. (2014). Carbamazepine for chronic neuropathic pain and fibromyalgia in adults. Cochrane database Syst. Rev. 2014, CD005451. doi:10.1002/14651858.CD005451.pub3
Wills, L., Nagarwalla, D., Pearson, C., Mcphail, S., Hinchliffe, R., Sharpless, B., et al. (2024). Estimating surgery, radiotherapy and systemic anti-cancer therapy treatment costs for cancer patients by stage at diagnosis. Eur. J. Health Econ. 25, 763–774. doi:10.1007/s10198-023-01623-5
Xia, J., Huang, N., Huang, H., Sun, L., Dong, S., Su, J., et al. (2016). Voltage-gated sodium channel Nav 1.7 promotes gastric cancer progression through MACC1-mediated upregulation of NHE1. Int. J. Cancer 139, 2553–2569. doi:10.1002/ijc.30381
Xia, J., Wang, H., Li, S., Wu, Q., Sun, L., Huang, H., et al. (2017). Ion channels or aquaporins as novel molecular targets in gastric cancer. Mol. Cancer 16, 54. doi:10.1186/s12943-017-0622-y
Xiao, W., Zhou, K., Yang, M., Sun, C., Dai, L., Gu, J., et al. (2021). Carbamazepine induces platelet apoptosis and thrombocytopenia through protein kinase A. Front. Pharmacol. 12, 749930. doi:10.3389/fphar.2021.749930
Yagi, C., Tatsuoka, J., Sano, E., Hanashima, Y., Ozawa, Y., Yoshimura, S., et al. (2022). Anti-tumor effects of anti-epileptic drugs in malignant glioma cells. Oncol. Rep. 48, 216. doi:10.3892/or.2022.8431
Yan, S., Chen, R., Wang, M., and Zha, J. (2021). Carbamazepine at environmentally relevant concentrations caused DNA damage and apoptosis in the liver of Chinese rare minnows (Gobiocypris rarus) by the Ras/Raf/ERK/p53 signaling pathway. Environ. Pollut. 270, 116245. doi:10.1016/j.envpol.2020.116245
Yang, D., Zhang, X., Zhang, W., and Rengarajan, T. (2018). Vicenin-2 inhibits Wnt/β-catenin signaling and induces apoptosis in HT-29 human colon cancer cell line. Drug Des. Devel Ther. 12, 1303–1310. doi:10.2147/DDDT.S149307
Yang, L., Shi, P., Zhao, G., Xu, J., Peng, W., Zhang, J., et al. (2020). Targeting cancer stem cell pathways for cancer therapy. Signal Transduct. Target Ther. 5, 8. doi:10.1038/s41392-020-0110-5
Yildirim-Kahriman, S. (2023). Effect of voltage-gated sodium channel inhibitors on the metastatic behavior of prostate cancer cells: a meta-analysis. Pak J. Biol. Sci. 26, 419–426. doi:10.3923/pjbs.2023.419.426
Yu, W. K., Xu, Z. Y., Yuan, L., Mo, S., Xu, B., Cheng, X. D., et al. (2020). Targeting β-catenin signaling by natural products for cancer prevention and therapy. Front. Pharmacol. 11, 984. doi:10.3389/fphar.2020.00984
Zhan, T., Rindtorff, N., and Boutros, M. (2017). Wnt signaling in cancer. Oncogene 36, 1461–1473. doi:10.1038/onc.2016.304
Zhang, J., and Zhong, Q. (2014). Histone deacetylase inhibitors and cell death. Cell. Mol. life Sci. 71, 3885–3901. doi:10.1007/s00018-014-1656-6
Zhang, J. J., Zhou, Q. M., Chen, S., and Le, W. D. (2018). Repurposing carbamazepine for the treatment of amyotrophic lateral sclerosis in SOD1-G93A mouse model. CNS Neurosci. Ther. 24, 1163–1174. doi:10.1111/cns.12855
Zhao, Y., Ren, J., Hillier, J., Lu, W., and Jones, E. Y. (2020). Antiepileptic drug carbamazepine binds to a novel pocket on the Wnt receptor frizzled-8. J. Med. Chem. 63, 3252–3260. doi:10.1021/acs.jmedchem.9b02020
Zhen Sun, M., Van Rijn, C. M., Xi Liu, Y., and Zheng Wang, M. (2002). Combination of carbamazepine and valproate in different dose proportions in maximal electroshock seizure model in mice. Epilepsy Res. 51, 5–11. doi:10.1016/s0920-1211(02)00053-0
Zhong, Z., and Virshup, D. M. (2020). Wnt signaling and drug resistance in cancer. Mol. Pharmacol. 97, 72–89. doi:10.1124/mol.119.117978
Zoccarato, M., Basile, A. M., Padovan, M., Caccese, M., Zagonel, V., and Lombardi, G. (2021). Eslicarbazepine in patients with brain tumor-related epilepsy: a single-center experience. Int. J. Neurosci. 131, 879–884. doi:10.1080/00207454.2020.1759590
Keywords: dibenzazepines, carbamazepine, oxcarbazepine, eslicarbazepine, cancer therapy
Citation: Afsordeh N, Pournajaf S, Mirnajafi-Zadeh J and Pourgholami MH (2025) The potential of dibenzazepine carboxamides in cancer therapy. Front. Pharmacol. 16:1564911. doi: 10.3389/fphar.2025.1564911
Received: 22 January 2025; Accepted: 17 March 2025;
Published: 28 March 2025.
Edited by:
Deqiang Dou, Liaoning University of Traditional Chinese Medicine, ChinaReviewed by:
Xiangxuan Zhao, Liaoning University of Traditional Chinese Medicine, ChinaCopyright © 2025 Afsordeh, Pournajaf, Mirnajafi-Zadeh and Pourgholami. This is an open-access article distributed under the terms of the Creative Commons Attribution License (CC BY). The use, distribution or reproduction in other forums is permitted, provided the original author(s) and the copyright owner(s) are credited and that the original publication in this journal is cited, in accordance with accepted academic practice. No use, distribution or reproduction is permitted which does not comply with these terms.
*Correspondence: Mohammad Hossein Pourgholami, cG91cmdob2xhbWkubWhAZ21haWwuY29t
Disclaimer: All claims expressed in this article are solely those of the authors and do not necessarily represent those of their affiliated organizations, or those of the publisher, the editors and the reviewers. Any product that may be evaluated in this article or claim that may be made by its manufacturer is not guaranteed or endorsed by the publisher.
Research integrity at Frontiers
Learn more about the work of our research integrity team to safeguard the quality of each article we publish.