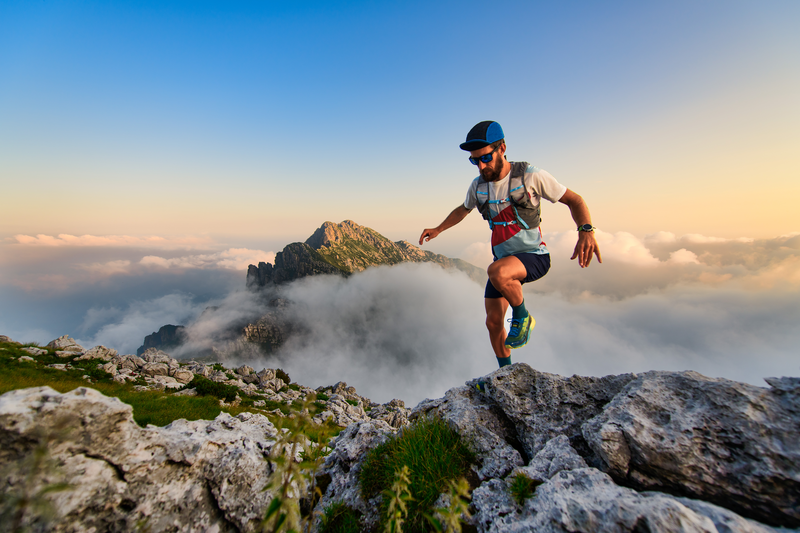
94% of researchers rate our articles as excellent or good
Learn more about the work of our research integrity team to safeguard the quality of each article we publish.
Find out more
ORIGINAL RESEARCH article
Front. Pharmacol.
Sec. Neuropharmacology
Volume 16 - 2025 | doi: 10.3389/fphar.2025.1564851
The final, formatted version of the article will be published soon.
You have multiple emails registered with Frontiers:
Please enter your email address:
If you already have an account, please login
You don't have a Frontiers account ? You can register here
There is increasing evidence that astrocytes are involved in the therapeutic action of antidepressants. The fast antidepressant YL-0919 may interact with activation of astrocytic sigma-1 receptors (sigma-1R). In this study, function of astrocytic sigma-1R in ventral hippocampus (vHIP) mediating the rapid antidepressant effect of YL-0919 were investigated. Adeno-associated virus (AAV) expressing shRNA was constructed to knock down astrocytic sigma-1R in vHIP, and the role of astrocytic sigma-1R on the rapid antidepressant action of YL-0919 were tested in chronic restraint stress (CRS) model of mice. Small interfering RNA (siRNA) was used to knock down sigma-1R in primary astrocytes, and we explored the mitochondrial function and BDNF expression of primary astrocytes after YL-0919 and siRNA treatments. The results indicated knocking down astrocytic sigma-1R in vHIP induced anxiety-like and depressive-like behavior in mice, and blocked the rapid anti-depressant and anxiolytic effects of YL-0919. Knocking down sigma-1R in primary astrocytes inhibited the YL-0919 induced enhancement of mitochondrial function and increased level of BDNF expression. In addition, increased BDNF in vHIP might play a role in fast antidepressant impact of YL-0919. Taken together, the data provide further evidence for a role of astrocyte receptors in the mechanisms of action of antidepressants.
Keywords: Astrocytes, sigma-1 receptor, Antidepression, YL-0919, BDNF (brain derived neurotrophic factor)
Received: 22 Jan 2025; Accepted: 03 Mar 2025.
Copyright: © 2025 Li, Chang, Zhao, Bao, Dai and Li. This is an open-access article distributed under the terms of the Creative Commons Attribution License (CC BY). The use, distribution or reproduction in other forums is permitted, provided the original author(s) or licensor are credited and that the original publication in this journal is cited, in accordance with accepted academic practice. No use, distribution or reproduction is permitted which does not comply with these terms.
* Correspondence:
Wei Dai, Beijing Institute of Pharmacology & Toxicology, Beijing, China
Disclaimer: All claims expressed in this article are solely those of the authors and do not necessarily represent those of their affiliated organizations, or those of the publisher, the editors and the reviewers. Any product that may be evaluated in this article or claim that may be made by its manufacturer is not guaranteed or endorsed by the publisher.
Research integrity at Frontiers
Learn more about the work of our research integrity team to safeguard the quality of each article we publish.