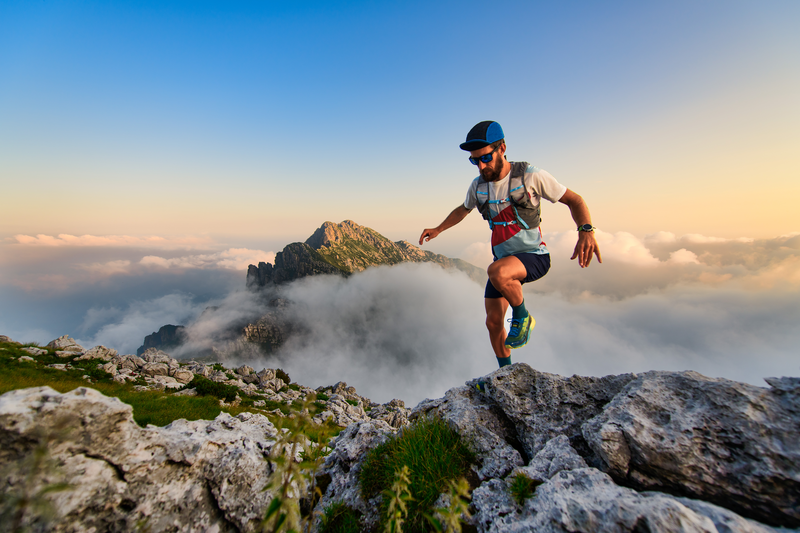
94% of researchers rate our articles as excellent or good
Learn more about the work of our research integrity team to safeguard the quality of each article we publish.
Find out more
ORIGINAL RESEARCH article
Front. Pharmacol.
Sec. Pharmacology of Infectious Diseases
Volume 16 - 2025 | doi: 10.3389/fphar.2025.1564070
This article is part of the Research Topic ADME of Drugs to Treat Infectious Diseases View all 6 articles
The final, formatted version of the article will be published soon.
You have multiple emails registered with Frontiers:
Please enter your email address:
If you already have an account, please login
You don't have a Frontiers account ? You can register here
Fluconazole pharmacokinetics in acute renal failure (ARF) patients undergoing continuous renal replacement therapy (CRRT) are significantly influenced by the combined effects of impaired renal function and CRRT, yet current dosing guidelines do not account for these complexities, leading to suboptimal therapy and treatment failure. This study aimed to address these limitations by developing a population pharmacokinetic model for fluconazole in ARF patients receiving CRRT, evaluating guideline-recommended dosing regimens for pharmacokinetic/pharmacodynamic target attainment, and then developing software to optimize fluconazole dosing in complex clinical CRRT scenarios. A total of 297 literature-sourced plasma concentration data points from 15 ARF patients and one patient with normal renal function, all receiving CRRT, were used for model construction. The treatment target was set as the 24-hour area under the free drug concentration-time curve to the minimum inhibitory concentration ratio ≥100. The web application was developed using R and R packages. The final pharmacokinetic model comprised a central and CRRT compartment, with renal failure and CRRT doses influencing clearance and body weight affecting central compartment distribution volume. Simulations revealed that the guideline-recommended loading (800 mg or 12 mg/kg QD) and maintenance doses (400 mg or 6 mg/kg QD) achieved limited target attainment at low CRRT doses and failed at moderate to high CRRT doses. Consequently, dose adjustments based on body weight and CRRT parameters are recommended. A user-friendly, visual, and interactive Shiny application was developed to assist clinicians in optimizing fluconazole dosing in 30 this challenging patient population.
Keywords: Fluconazole, Acute renal failure, continuous renal replacement therapy, population pharmacokinetics, PK/PD, Shiny application
Received: 21 Jan 2025; Accepted: 10 Mar 2025.
Copyright: © 2025 Zhang, Zhang, Wu, Qin and Pei. This is an open-access article distributed under the terms of the Creative Commons Attribution License (CC BY). The use, distribution or reproduction in other forums is permitted, provided the original author(s) or licensor are credited and that the original publication in this journal is cited, in accordance with accepted academic practice. No use, distribution or reproduction is permitted which does not comply with these terms.
* Correspondence:
Qi Pei, Department of Pharmacy, Third Xiangya Hospital, Central South University, Changsha, China
Disclaimer: All claims expressed in this article are solely those of the authors and do not necessarily represent those of their affiliated organizations, or those of the publisher, the editors and the reviewers. Any product that may be evaluated in this article or claim that may be made by its manufacturer is not guaranteed or endorsed by the publisher.
Research integrity at Frontiers
Learn more about the work of our research integrity team to safeguard the quality of each article we publish.