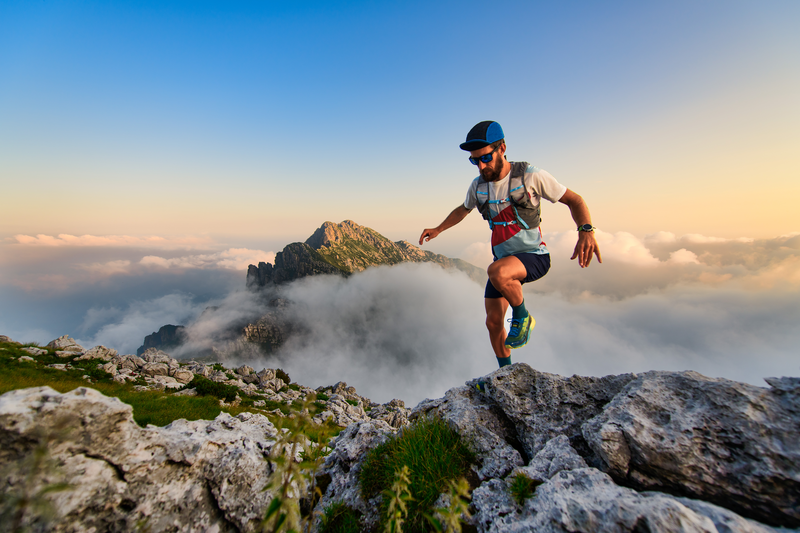
94% of researchers rate our articles as excellent or good
Learn more about the work of our research integrity team to safeguard the quality of each article we publish.
Find out more
REVIEW article
Front. Pharmacol.
Sec. Pharmacology of Anti-Cancer Drugs
Volume 16 - 2025 | doi: 10.3389/fphar.2025.1562167
This article is part of the Research Topic Exploring Novel Resistance Mechanisms in Cancer Treatments through High-Throughput Screening and Multi-Omics Analysis View all articles
The final, formatted version of the article will be published soon.
You have multiple emails registered with Frontiers:
Please enter your email address:
If you already have an account, please login
You don't have a Frontiers account ? You can register here
Inhibitors of Apoptosis Proteins (IAPs) are a family of anti-apoptotic proteins that play a pivotal role in apoptosis in general but also as oncoproteins in cancer progression and, more importantly, drug resistance. IAPs enable cancer cells to evade programmed cell death and adapt to therapeutic stress by inhibiting pro-apoptotic caspase activity as well as modulating pivotal survival pathways.Recent advancements in targeting IAPs, particularly through the use of SMAC (second mitochondria-derived activator of caspase) mimetics and other small-molecule antagonists or inhibitors, have opened new avenues for overcoming drug resistance in cancers. The current review attempted to summarize the status quo of IAPs' role in promoting chemotherapeutic drug resistance in various cancer treatments and discuss the most recent development of IAP-targeting therapies, particularly small-molecule inhibitors including their combinational strategies to enhance the sensitivity or achieve synergism to existing therapeutics. Additionally, we also outline the challenges and offer future perspectives for optimizing IAP-targeted approaches to improve clinical outcomes.
Keywords: Drug Resistance, Cancer, Inhibitors of apoptosis proteins, inhibitors, Overcome
Received: 17 Jan 2025; Accepted: 21 Mar 2025.
Copyright: © 2025 Ye, Zhuang, Li and Zhou. This is an open-access article distributed under the terms of the Creative Commons Attribution License (CC BY). The use, distribution or reproduction in other forums is permitted, provided the original author(s) or licensor are credited and that the original publication in this journal is cited, in accordance with accepted academic practice. No use, distribution or reproduction is permitted which does not comply with these terms.
* Correspondence:
Juan Li, Hubei University of Chinese Medicine, Wuhan, 430065, Hubei Province, China
Xin Zhou, The Fifth People’s Hospital of Hainan Province, Haikou, Hainan Province, China
Disclaimer: All claims expressed in this article are solely those of the authors and do not necessarily represent those of their affiliated organizations, or those of the publisher, the editors and the reviewers. Any product that may be evaluated in this article or claim that may be made by its manufacturer is not guaranteed or endorsed by the publisher.
Research integrity at Frontiers
Learn more about the work of our research integrity team to safeguard the quality of each article we publish.