- Department of Crop Sciences and Agroforestry, Faculty of Tropical AgriSciences, Czech University of Life Sciences Prague, Prague, Czechia
The association and causal role of infectious agents in chronic inflammatory diseases have major implications for public health, treatment, and prevention. Pharmacological treatment of combined infectious and inflammatory diseases requires the administration of multiple drugs, including antibiotics and anti-inflammatory drugs. However, this can cause adverse effects, and therefore, dual-action drugs need to be developed. Anti-inflammatory drugs that have already shown antimicrobial properties appear to be promising candidates. NSAIDs, namely aceclofenac, diclofenac, and ibuprofen, were tested in clinical trials with patients diagnosed with uncomplicated urinary tract infections (UTIs) and cellulitis. The administration of ibuprofen, a drug tested in the highest number of studies, resulted in symptom resolution in patients with UTIs. Additionally, ibuprofen caused a high survival rate in mice infected with Pseudomonas aeruginosa and demonstrated potent in vitro antibacterial effects against Bacillus cereus, Escherichia coli, and Staphylococcus aureus, including methicillin-resistant S. aureus (MRSA) (MIC 0.625–2.5 mg/L). For most anti-inflammatory drugs, only data showing their in vitro and in vivo antimicrobial effects are available. Among these, auranofin caused a high survival rate in mice infected with Enterococcus faecium, S. aureus, and Clostridioides difficile. It also produced a strong in vitro growth-inhibitory effect against Streptococcus agalactiae, S. pneumoniae, S. aureus, S. epidermidis, Bacillus subtilis, C. difficile, E. faecalis, E. faecium, and Mycobacterium tuberculosis (MIC 0.0015–5 mg/L). Similarly, aspirin caused a high survival rate in M. tuberculosis-infected mice and strong to moderate in vitro activity against E. coli, B. cereus, P. aeruginosa, Enterobacter aerogenes, Klebsiella pneumoniae and Salmonella choleraesuis (MIC 1.2–5 mg/L). Moreover, topical application of celecoxib resulted in a high reduction in MRSA burden in mice. However, it only caused moderate in vitro effects against S. epidermidis, S. aureus and Bacillus subitilis (MIC 16–64 mg/L). These data suggest that certain non-steroidal anti-inflammatory drugs (NSAIDs) are promising drug candidates for the development of dual-action drugs for the potential treatment of combined infectious and inflammatory diseases such as tuberculosis, musculoskeletal infections and UTIs. Nevertheless, future clinical trials must be conducted to ascertain the antibacterial effect of these NSAIDs before their practical use.
1 Introduction
Clinical and epidemiological studies have suggested an association between infectious agents and chronic inflammatory disorder (Karin et al., 2006). For example, tuberculosis (TB), musculoskeletal infections (MSKIs) and urinary tract infections (UTIs) may trigger chronic inflammation, which may lead to severe tissue damage (Lew and Waldvogel, 2004; Zumla et al., 2015; Abraham et al., 2001). Combating these infections may require that patients be treated with a combination of antibiotics and anti-inflammatory drugs. However, multiple drug administration (known as polypharmacy) may lead to adverse health consequences due to drug-drug and drug-disease interactions (Patyar et al., 2011; Steinman et al., 2006; Field et al., 2004). For example, the administration of high doses of amoxicillin/clavulanate in combination with warfarin is associated with a higher risk of over-anticoagulation (Abdel-Aziz et al., 2016). There is also a concern that the interaction between non-steroidal anti-inflammatory drugs (NSAIDs) and antihypertensive drugs may increase the risk of acute kidney disease (Lapi et al., 2013). To minimise the severe side effects caused by polypharmacy, there is a prevalent need to develop dual-action drugs, which are compounds that combine two different desired pharmacological actions and possess dual mechanistic effects due to their targeting of different effector mechanisms (Patyar et al., 2011). Besides the decreased adverse effects, the use of such drugs can lead to improved medication efficacy (Patyar et al., 2011). For example, the administration of rivastigmine, a dual inhibitor of acetylcholinesterase and butyrylcholinesterase, demonstrated significantly greater responses of cognitive and behavioural functions in patients with dementia than the selective acetylcholinesterase inhibitors such as donepezil and galantamine (Ballard, 2002; Kandiah et al., 2017). Similarly, preclinical and clinical data demonstrated that bupropion, an antidepressant medication used to treat depression, acts through the dual inhibition of norepinephrine and dopamine reuptake. Besides its efficacy, which is comparable to other antidepressants, bupropion therapy is not associated with common antidepressant-associated side effects, such as sexual dysfunction, weight gain, and sedation (Stahl et al., 2004). Romosozumab is an osteoanabolic drug with dual action used for the treatment of osteoporosis. It binds to and inhibits sclerostin (a natural inhibitor of bone formation) and exhibits a dual effect by stimulating bone formation and reducing bone resorption. Thus, romosozumab can be best characterised as a dual agent, demonstrating osteoanabolic and antiresorptive functions (Tabacco and Bilezikian, 2019). The case of romosozumab can also clearly illustrate the future market potential of dual-action drugs. For example, the sales of romosozumab increased 36% year-over-year to $431 million in the fourth quarter of 2024 and 35% for the full year (Amgen, 2024). Moreover, clinical experts suggest that the market share of romosozumab will increase each year until 2026/2027 when it reaches 50% of the eligible population (National Institute for Health and Care Excellence, 2022). Additionally, 12 months of treatment of osteoporosis patients with romosozumab followed by 4 years of alendronate was a more cost-effective option with greater quality-adjusted life years compared to other alternatives (Tabacco and Bilezikian, 2019; Amgen Canada Inc, 2022). Latanoprostene bunod, used for the treatment of glaucoma, is another example of a dual-action drug with great market potential. This agent increases trabecular and uveoscleral outflow and lowers intraocular pressure much better than other prostaglandin analogues (Mendelsohn, 2022). The drug, which recorded 18% total prescription growth in the fourth quarter of 2022, was launched in 15 countries in 2022 and was set to expand to 10 more by 2023 (Bausch and Lomb, 2022).
Another promising alternative to polypharmacy is the use of multi-target drugs, which simultaneously act on multiple pathways. This approach offers safer and more effective treatment options for complex diseases such as cancer, inflammation, diabetes, and central nervous system disorders (Brown and Superti-Furga, 2003; Kamb et al., 2007; Cavalli et al., 2008). For example, imatinib is an effective multi-target drug used for the treatment of chronic myelogenous leukaemia and gastrointestinal stromal tumours. This drug specifically targets proto-oncogene c-Kit, tyrosine-protein kinase ABL1, and platelet-derived growth factor receptors involved in cancer signalling (Slomovitz et al., 2004). Other strategies include targeted drug delivery systems, drug repurposing and deprescribing. A targeted drug delivery system facilitates the delivery of drugs to their specific target site in the body, thereby enhancing therapeutic effects with reduced adverse effects (Rayaprolu et al., 2018; Vargason et al., 2021). Nanocarriers are promising targeted systems that selectively and effectively deliver drugs to targeted sites through enhanced permeability and retention (Dang and Guan, 2020). Additionally, implantable local delivery carriers used for the treatment of osteomyelitis deliver antibiotics in a controlled, slow and sustained manner from an implant to the infection site (Smith et al., 2022). On the other hand, drug repurposing involves finding new therapeutic uses for existing drugs outside the scope of their original medical indication (Pinzi et al., 2024). This approach reduces risks as the compounds have already passed toxicity and safety studies (March-Vila et al., 2017). It also creates the opportunity for repurposing the already-in-use drugs for a second indication, thereby providing treatments for unmet medical needs (Pinzi et al., 2024). For example, sildenafil developed as an anti-hypertensive and anti-anginal drug (Ghofrani et al., 2006), is repurposed for the treatment of erectile dysfunction (Ashburn and Thor, 2004). Deprescribing is the supervised discontinuation of a drug (Reeve et al., 2015) to reduce overtreatment and prevent adverse effects (Wu et al., 2021; Page et al., 2016). For example, lowering the doses of proton pump inhibitors was more beneficial because it reduces the risk of side effects and drug interactions and potentially reduces the cost of drugs (Farrell et al., 2017). Despite these alternative therapeutic approaches to polypharmacy, dual-action drugs have not been fully developed for the treatment of combined infection-inflammatory conditions such as MSKIs, TB, and UTIs.
MSKIs are inflammatory conditions that affect the bones and joints, causing serious morbidity and posing significant management challenges (Arkader et al., 2016). Osteomyelitis and septic arthritis are the most severe forms of MSKIs (Colston and Atkins, 2018), and Staphylococcus aureus together with S. epidermidis are their primary causative agents (Kavanagh et al., 2018; Goldenberg, 1998). Other bacteria such as Escherichia coli, Haemophilus influenzae, Neisseria gonorrhoeae, N. meningitidis, Pseudomonas aeruginosa, Proteus mirabilis, and Streptococcus pneumoniae can also be involved in MSKIs (Goldenberg, 1998; Tarkowski, 2006). Epidemiological studies showed that the total osteomyelitis prevalence increased to 10.44% in Germany from 2008 to 2018 (Walter et al., 2021), while 16, 382 emergency visits were recorded in the USA in 2012 for septic arthritis (Singh and Yu, 2018). Additionally, it is estimated that the cost of osteomyelitis treatment may rise to € 500,000,00 per case (Hogan et al., 2013). Although septic arthritis and osteomyelitis are primarily caused by microbial infections (Kalinka et al., 2014; Beck-Broichsitter et al., 2015; Goldenberg, 1998), pre-existing inflammatory conditions such as rheumatoid arthritis (RA) are one of the most common risk factors associated with these diseases (Krasselt et al., 2021; Dinescu et al., 2021). Certain cases of MSKIs are also characterised by early progressive inflammatory destruction of bone, contributing to the disease pathogenesis (Lew and Waldvogel, 2004). Therefore, the efficient treatment of MSKIs may require co-administration of antibiotics and NSAIDs. For example, antibiotics co-administered with anti-inflammatory drugs to patients with chronic osteomyelitis caused complete symptom resolution (Kudva et al., 2019). Antibiotic therapy (e.g. vancomycin) and surgical debridement are the most common treatment options for septic arthritis and osteomyelitis (Lew and Waldvogel, 2004; Copley, 2009; Kolinsky and Liang, 2018). However, the administration of antibiotics may cause adverse reactions such as phlebitis, hypotension, ototoxicity, nephrotoxicity, hypersensitivity, neutropenia, and interstitial nephritis (Bruniera et al., 2015). Surgical debridement involves the removal of the necrotic bone and tissue to improve the infected local environment and enhance antibiotic delivery (Urish and Cassat, 2020). Since traumatic surgical debridement may cause pain and inflammation (Lima et al., 2014), NSAIDs such as naproxen may be administered to patients with the aim of minimising inflammatory reactions (Chen et al., 2018).
TB is a severe communicable chronic disease caused by M. tuberculosis (Lawn and Zumla, 2011; Spitaleri et al., 2019), with a higher rate of infection found in children and the elderly (Esmail et al., 2018; Tahan et al., 2020). This disease remains a serious threat to global health, with an increase in morbidity and mortality worldwide (Mahmoud et al., 2016; Sood et al., 2016). Yearly, about 1.8 million people die from TB (Sasindran and Torrelles, 2011). During the primary stage of TB infection, the bacteria replicate in the lungs, causing inflammation by attracting monocytes and other inflammatory cells (Sasindran and Torrelles, 2011). Thus, inflammatory responses in patients are the most common pathological characteristic of M. tuberculosis (Liu et al., 2018). Moreover, the emergence of multidrug-resistant TB exacerbated the spread of infection (Richeldi et al., 2004). The recommended treatment for drug-susceptible TB may include a 4-month regimen of rifapentine (belonging to rifamycins), isoniazid, pyrazinamide, and moxifloxacin (Carr et al., 2022). However, the rise in multidrug-resistant TB (TB resistant to isoniazid and rifampicin) has led to the search for new anti-TB drugs (Svensson et al., 2015). Moreover, pathologic host immune reactions and excessive inflammation, which may result in tissue damage, are responsible for treatment failure in TB patients (Zumla et al., 2015). Thus, NSAIDs have been recommended as host-directed therapy (Ivanyi and Zumla, 2013), which can act on host immune effectors to decrease host-destructive pathology, including inflammation (Kroesen et al., 2017).
UTI is the most common bacterial infection in women, and half of the female population develops UTIs at least once in their lifetime (Colgan and Williams, 2011). Uncomplicated UTIs, such as cystitis, affect people with no structural urinary tract abnormalities (Hooton, 2012; Nielubowicz and Mobley, 2010; Hannan et al., 2012), while complicated UTIs affect people with conditions that may compromise their immune defence systems, such as renal transplantation, pregnancy, and indwelling catheters (Lichtenberger and Hooton, 2008; Levison and Kaye, 2013). Although microorganisms such as Klebsiella pneumonia (6%), Staphylococcus saprophyticus (6%), Enterococcus spp (5%), and P. mirabilis (2%) may also be involved, E. coli (75%) is the main cause of UTIs (Flores-Mireles et al., 2015). The expression of type 1 fimbriae virulent factor, which promotes strong adherence of E. coli to the uroepithelium, may cause inflammation of the urinary tract (Abraham et al., 2001). Moreover, a previous report showed that this factor modulates inflammatory responses of the host immune cells by binding to neutrophils, macrophages, and lymphocytes, thereby triggering the release of inflammatory mediators (Abraham et al., 1999). Therefore, the anti-inflammatory effect of NSAIDs on the urothelium may help alleviate the symptoms of uncomplicated UTIs (Sachdeva et al., 2021). For example, a previous study showed that administering NSAIDs to patients with recurrent cystitis resulted in positive clinical outcomes (Chung, 2016). Since evidence indicates that certain NSAIDs have produced antimicrobial action in various in vitro, in vivo studies and produce symptom resolution in clinical experiments (Thangamani et al., 2015; Dutta et al., 2007a; Cassetta et al., 2014; Chan et al., 2017; Abutaleb and Seleem, 2020a; Bleidorn et al., 2010), they are considered good candidates for developing dual-action drugs for the treatment of UTI and other inflammation-associated infections, including TB and MSKIs.
Anti-inflammatory drugs are among the most used therapeutic groups of agents worldwide (Domingos et al., 2019). They are used for a wide variety of indications, including pain treatment, traumatism, inflammatory and autoimmune diseases, and many of them can be obtained over the counter (Gomez-Acebo et al., 2018). Corticosteroids and NSAIDs are the two main groups of anti-inflammatory drugs. Corticosteroids are steroid hormones produced physiologically by vertebrates and their synthetic analogues (Ferrara et al., 2019), which inhibit the phospholipase A2 enzyme (Whittle, 2000). They exhibit potent anti-inflammatory and immunosuppressive effects (Pallio et al., 2016; Stanbury and Graham, 1998). Although certain corticosteroids (e.g., corticosterone) exhibit some antibacterial effects, only a few of them produce this action which is inferior to the current antibacterial drugs (Dogan et al., 2017). On the other hand, the antimicrobial activities of NSAIDs have been demonstrated in a number of in vitro and in vivo studies (Zhang et al., 2021; Thangamani et al., 2015). Their anti-inflammatory mechanism of action is through the inhibition of cyclooxygenases (COX-1 and COX-2), the key enzymes involved in prostaglandin synthesis (Bindu et al., 2020; Yao and Narumiya, 2019). According to the WHO List of Essential Medicines, NSAIDs are among the most frequently prescribed anti-inflammatory drugs (Bindu et al., 2020). In addition, they are also used as antipyretics to reduce fever and as analgesics in pain management (Bindu et al., 2020; Yao and Narumiya, 2019). Evidence has shown that apart from taking antibiotics, patients are usually administered anti-inflammatory drugs to manage inflammation associated with several diseases. Thus, NSAIDs have become first-choice drugs for this purpose (Nugrahani et al., 2023). Because of the rich evidence of their antimicrobial effects (Thangamani et al., 2015; Chan et al., 2017), they are promising candidates for developing drugs with dual anti-inflammatory and antimicrobial activities to potentially treat combined infectious and inflammatory diseases such as MSKIs, TB and UTIs. Most importantly, using NSAIDs as a single-drug therapy could reduce the risk of adverse drug reactions caused by multiple drug co-administration. However, the results of current research on the antimicrobial effects of these drugs have yet to be sufficiently reviewed. Thus, this review summarises and critically analyses the in vitro, in vivo, and clinical data on the antimicrobial efficacy of anti-inflammatory drugs.
2 Antimicrobial activities of anti-inflammatory drugs
2.1 In vitro studies
The literature analysis identified 17 anti-inflammatory drugs, namely aspirin, auranofin, bromfenac, carprofen, celecoxib, diacerein, diclofenac, flufenamic acid, flurbiprofen, ibuprofen, indomethacin, meclofenamic acid, naproxen, nimesulide, sodium salicylate, tolfenamic acid and vedaprofen producing in vitro antimicrobial activity against 60 bacterial and fungal species including Gram-positive bacteria (Bacillus anthracis, Bacillus cereus, Bacillus subtilis, Clostridioides difficile, E. durans, E. faecalis, E. faecium, Gemella haemolysans, Listeria monocytogenes, Micrococcus luteus, Mycobacterium smegmatis, M. tuberculosis, S. aureus, S. capitis, S. epidermidis, S. haemolyticus, S. hominis, S. intermedius, S. xylosus, Streptococcus agalactiae, S. mitis, S. pneumoniae, S. salivarius, and S. sanguinis) and Gram-negative bacteria (Acinetobacter baumannii, A. baylyi, Enterobacter aerogenes, E. cloacae, E. coli, Francisella novicida, F. tularensis, Helicobacter pylori, K. pneumoniae, N. gonorrhoeae, Paracoccus yeei, P. aeruginosa, Salmonella choleraesuis, S. typhi, and S. typhimurium) and fungi (Aspergillus brasiliensis, A. fumigatus, Candida albicans, C. glabrata, C. guilliermondi, C. krusei, C. lusitaniae, C. neoformans, C. parapsilosis, C. tropicalis, Cryptococcus gattii, C. neoformans, Epidermophyton fluccosum, Microsporum canis, M. fulva, M. gypseum, Trichophyton interdigitale, T. mentagrophytes, T. rubrum, T. tonsurans and T. violaceum). Detailed data on the in vitro antimicrobial activity of anti-inflammatory drugs, including their MIC and MBC values, are shown in Table 1. Aspirin, auranofin, celecoxib, diclofenac, diacerein, and ibuprofen belong to the most frequently tested and antimicrobially active anti-inflammatory drugs.
Auranofin is a trialkylphosphine gold complex approved for the treatment of RA (Harbut et al., 2015; Cassetta et al., 2014; Mingh, 2007) that has been in clinical use since 1985 (Shaw, 1999). Although the knowledge of the use of gold complexes in clinical settings is not new, the interest in them and their derivatives has risen in recent years due to their broad-spectrum antimicrobial activities and unique modes of action (Ratia et al., 2022). According to the literature data, auranofin is an anti-inflammatory agent most frequently studied for its antimicrobial activity, which produced the strongest in vitro growth inhibitory effect against S. agalactiae, S. pneumoniae, S. aureus, S. epidermidis B. subtilis, C. difficile, E. faecalis, E. faecium, and M. tuberculosis with MIC ranging from 0.0015 to 5 mg/L (Thangamani et al., 2016a; Thangamani et al., 2016b; Cassetta et al., 2014; Harbut et al., 2015; Abdelkhalek et al., 2019; Abdelkhalek et al., 2018; Abutaleb and Seleem, 2020a; Abutaleb and Seleem, 2020b). More importantly, the drug also showed potent activity against S. aureus, including drug-resistant clinical isolates, vancomycin-intermediate S. aureus (VISA), vancomycin-resistant S. aureus (VRSA) and methicillin-resistant S. aureus (MRSA), (MIC ranging from 0.0625–1.357 mg/L), the principal bacterial agent responsible for the most severe forms of MSKIs, such as osteomyelitis and septic arthritis (Cassetta et al., 2014; Harbut et al., 2015; Thangamani et al., 2016b; Ferretti et al., 2025; Quadros Barse et al., 2024; Chiaverini et al., 2022; Tong et al., 2015). Despite the broader range of MICs observed for S. aureus, the results adequately characterise its in vitro susceptibility to auranofin because, in seven independent studies, a total of 39 strains were tested, including clinical isolates and resistant strains. Auranofin also exhibited anti-biofilm properties as it reduced S. aureus biofilm mass by >60% at a concentration of 1 mg/L, which indicates its anti-biofilm activity (Thangamani et al., 2016b). Studies on the drug’s antimicrobial mechanism of action showed that it inhibits the bacterial thioredoxin reductase enzyme, which protects Gram-positive bacteria such as S. aureus against reactive oxidative species (Harbut et al., 2015; Liao et al., 2017).
The antimicrobial activity of aspirin was also investigated in a number of studies (Al-Bakri et al., 2009; Chan et al., 2017; Ozturk et al., 2021). Aspirin (also known as acetylsalicylic acid), a non-selective COX inhibitor used in managing acute MSKI-related pain, belongs to the salicylic acid derivatives (Ornelas et al., 2017; Kowalski and Stevenson, 2013; Davis et al., 2022). Research findings showed that this NSAID exhibited broad-spectrum antimicrobial activity against Gram-positive (B. cereus), Gram-negative (E. aerogenes, K. pneumoniae, E. coli, P. aeruginosa, S. choleraesuis) bacteria and yeast (C. albicans) with MIC of ≥1.2 mg/L (Al-Bakri et al., 2009; Chan et al., 2017). In addition, it exerted a moderate bactericidal effect against methicillin-susceptible S. aureus (MSSA) ATCC 25923 and MRSA ATCC 33591 at an MBC of 2.5 mg/L (Chan et al., 2017). At the same time, it showed weak MBC activity against MRSA clinical isolates, B. cereus, Gram-negative bacteria such as S. choleraesuis, E. aerogenes, K. pneumoniae, P. aeruginosa, E. coli and yeast (C. albicans) at MBC ≥4.8 mg/L (Chan et al., 2017; Al-Bakri et al., 2009). Interestingly, aspirin exerted a similar antimicrobial effect against MSSA (ATCC25923) and MRSA (ATCC 33591) strains, including resistant clinical isolates with a MIC of 2.5 mg/L (Chan et al., 2017). However, there were some differences in the effect of aspirin against E. coli strains. For example, the MIC obtained when aspirin was tested against E. coli ATCC 8739 and E. coli ATCC 25922 was 1.2 mg/L and 5 mg/L. Similarly, the susceptibility of P. aeruginosa ATCC 9027 and P. aeruginosa ATCC 10145 to aspirin were with MIC of 2.03 mg/L and 5 mg/L, respectively (Chan et al., 2017; Al-Bakri et al., 2009). Aspirin also exhibited an antibiofilm activity against E. coli, P. aeruginosa, and C. albicans, causing a concentration-dependent reduction of the viable bacteria count. The kinetics of antibiofilm effect results showed that an exposure time of 4 h caused a percentage reduction of 98.23, 94.25 and 93.61 in the viable counts of P. aeruginosa, E. coli and C. albicans biofilms, respectively. Aspirin’s minimal biofilm eradication concentration values against the established biofilms ranged between 1.35 and 3.83 mg/L (Al-Bakri et al., 2009). The antibiofilm activity of aspirin against certain bacteria (e.g. P. aeruginosa) is believed to stem from its ability to inhibit quorum sensing by downregulating key quorum-sensing genes (lasI, lasR, rhlR, pqsA, pqsR). This disruption leads to reduced production of biofilm, adhesins, and toxins (El-Mowafy et al., 2014). Moreover, another study found that aspirin downregulates algD expression, increasing bacterial susceptibility to antibiotics (Tabatabaeifar et al., 2022). The algD operon in P. aeruginosa regulates alginate synthesis, thereby promoting biofilm formation and bacteria resistance to phagocytosis and antibiotics (Blanco-Cabra et al., 2020; Powell et al., 2018). Aspirin also exhibits antibiofilm activity against S. aureus by the inhibition of agrA-regulated virulence genes and downregulating biofilm-associated genes such as icaA and fnbA (Tabatabaeifar et al., 2022). Notably, aspirin suppresses icaA expression, which is crucial for producing polysaccharide intercellular adhesin, a key structural component of S. aureus biofilms (Cramton et al., 1999). Moreover, clinical evidence suggests that aspirin therapy reduces the risk of S. aureus-induced bacteremia; therefore, it is recommended for postoperative treatment to decrease graft-related infections emanating from coagulase-negative staphylococci such as S. epidermidis, which is implicated in osteomyelitis and septic arthritis (Sedlacek et al., 2007; Demirag et al., 2007). However, studies have shown that aspirin slightly increases fluoroquinolone resistance in ciprofloxacin-susceptible and resistant S. aureus strains (Gustafson et al., 1999). It enhances fluoroquinolone resistance by inducing the S. aureus multiple antibiotic resistance operon, which increases the production of the S. aureus NorA efflux pump, consequently reducing fluoroquinolone accumulation (Ohshita et al., 1990). Additionally, Verma et al. (2018) demonstrated that exposure of planktonic E. coli to aspirin could increase resistance to ciprofloxacin and tetracycline.
Ibuprofen, another NSAID possessing antimicrobial activity, is an essential drug developed in the 1960s for treating RA. It is currently one of the most used non-prescription drugs globally (Davies, 1998; Oliveira et al., 2019), which belongs to the aryl propionic acid class of compounds (Kowalski and Stevenson, 2013). Chan et al. (2017) evaluated the antibacterial activity of ibuprofen against several bacteria. Findings showed that the drug mostly inhibited the growth of B. cereus, followed by MRSA and MSSA, with MIC ranging from 0.625 to 2.5 mg/L. Further bactericidal studies confirmed the potency of this compound against B. cereus and S. aureus with an MBC range of 2.5–5.0 mg/L (Chan et al., 2017). The drug also showed bactericidal activity against the MRSA clinical isolates (MBC ≥5 mg/L), thus exhibiting both bacteriostatic and bactericidal effects against B. cereus and S. aureus (Chan et al., 2017). In another study, Al-Janabi. (2010) demonstrated that P. yeei and S. aureus were the most susceptible pathogens to ibuprofen and obtained a lower MIC of 1.25 mg/L against S. aureus. The investigation of ibuprofen’s antimicrobial mechanism of action on S. aureus using potassium iodide uptake and intracellular K+ release tests showed evidence of cytoplasmic membrane destabilisation and disruption (Oliveira et al., 2019). By monitoring the number of colony-forming units and growth kinetics, Shah et al. (2018) demonstrated that ibuprofen reduced the growth rate of P. aeruginosa. This pathogen causes life-threatening MSKIs such as osteomyelitis. Similarly, Dai et al. (2019) proved that ibuprofen inhibited P. aeruginosa biofilm formation. Ibuprofen demonstrated antibiofilm effects by interfering with quorum-sensing signalling molecules (lasI, lasR, rhll, rhlR, pqsA, and pqsR) in P. aeruginosa, thereby reducing the expression of biofilm-associated genes. Additionally, ibuprofen reduced the release of pyocyanin, rhamnolipid and protease—key virulence factors regulated in P. aeruginosa by quorum-sensing (Dai et al., 2019). Also, a recent study demonstrated that ibuprofen significantly decreased the transcription level of algD in P. aeruginosa and icaA in S. aureus (Tabatabaeifar et al., 2022). Since quorum sensing inhibitors act to directly prevent biofilm formation and limit the production of virulence factors, they are being considered as a novel strategy for treating P. aeruginosa infections (Le Berre et al., 2006). Thus, the antibiofilm and anti-quorum sensing activity of ibuprofen indicates that it can be a candidate drug for the treatment of clinical infections caused by P. aeruginosa (Dai et al., 2019). Besides its antibacterial action, ibuprofen also inhibited the growth of several Candida strains with a MIC ranging from 1 to 3 mg/L (Pina-Vaz et al., 2000).
Diclofenac is among the most used NSAIDs for reducing fever, pain, and inflammation, especially in patients with arthritis (Zhang et al., 2021; Hamed et al., 2021). The drug, which belongs to the heteroaryl acetic acid class of NSAIDs (Kowalski and Stevenson, 2013), produced antibacterial properties against several bacteria (Chan et al., 2017). Among them, MRSA and B. cereus were the most susceptible pathogens, with MIC ranging from 0.3125 to 2.5 mg/L. However, Dastidar et al. (2000) demonstrated that diclofenac inhibited the growth of S. aureus at a much higher MIC of 50 mg/L. Variations in the MIC values observed may be attributed to the difference in the susceptibility of standard strains and clinical isolates tested. Diclofenac was also bactericidal against B. cereus and MRSA ATCC 33591 with an MBC of 2.5 mg/L (Chan et al., 2017). Notably, this agent displayed a low resistance rate compared to antibiotics, such as daptomycin and vancomycin (Humphries et al., 2013; Zhang et al., 2021). A study that investigated the anti-virulence effect of diclofenac against multi-drug resistance MRSA clinical isolates demonstrated a significant reduction in biofilm formation. Also, remarkable inhibition of hemolysin activity was observed. In addition, diclofenac has inhibitory activity against staphyloxanthin production and downregulated MRSA virulence genes, including SarA, Hla, FnbA, IcaA, SigB, CrtM and AgrA, which is considered a quorum sensing regulatory gene of S. aureus and plays a role in the upregulation of superantigens, cytotoxins, and secreted enzymes (Abbas et al., 2020). Similarly, Elmesseri et al.(2023) showed that diclofenac potently inhibited the synthesis of staphyloxanthin, a key virulence factor for the survival of MRSA against host innate immunity. Additionally, treated cells revealed a significant downregulation of virulence genes responsible for staphyloxanthin synthesis, such as crtM, crtN and global transcriptional regulator sigB, along with the Hla gene (Elmesseri et al., 2023). Investigation of proteomic alterations in MRSA showed that diclofenac alters the pathways associated with β-lactams resistance, energy metabolism, and peptidoglycan biosynthesis (Zhang et al., 2021).
Celecoxib is a selective COX-2 inhibitor approved for treating RA (Silverstein et al., 2000; Krasselt et al., 2021; Dinescu et al., 2021). It belongs to the pyrazole class of drugs and has several clinical applications due to its antimicrobial, anti-inflammatory, and analgesic properties (Kucukguzel and Senkardes, 2015). Thangamani et al. (2015) investigated celecoxib’s antibacterial effect against several multidrug-resistant and Gram-negative bacteria strains. The drug showed a moderate antibacterial effect against all the Gram-positive bacteria tested, including B. anthracis, B. subtilis, MRSA, VRSA, L. monocytogenes, MRSA, and VISA clinical isolates (MIC = 16–32 mg/L). In contrast, the drug was inactive against all the Gram-negative bacteria. Chiu et al. (2012) and Gajdacs and Spengler (2019) demonstrated the anti-staphylococcal effect of this drug against S. aureus and S. epidermidis with MIC ranging from 15 to 32 mg/L. In addition, Okpala et al. (2024) recently showed that celecoxib inhibited the growth of S. aureus, including the clinical isolates at MIC ranging from 32 to 64 mg/L. Regarding the drug’s antimicrobial mechanisms of action, Thangamani et al. (2015) demonstrated that celecoxib inhibits RNA, DNA, and protein synthesis in S. aureus.
Diacerein, a semisynthetic anthraquinone derivative that inhibits interleukin-1β is commonly used as a slow-acting drug to treat joint diseases such as osteoarthritis (Bruneton, 1999; Fidelix et al., 2014; Pavelka et al., 2016). Zhang et al. (2019) examined the antibacterial activity of diacerein against several Gram-positive cocci isolated from bacterial keratitis patients. Their results showed that the most susceptible bacteria to this agent were S. epidermidis, S. xylosus, S. intermedius, and S. haemolyticus, with MIC ranging from 1 to 16 mg/L. In a separate study, Nguon et al. (2013) also demonstrated the anti-staphylococcal effect of diacerein against several S. aureus strains, including MSSA and MRSA, with MIC values ranging from 16 to 43 mg/L.
Based on the literature data, the NSAIDs produced a stronger effect against Gram-positive (MICs = 0.0015–2000 mg/L, MBCs = 2–40 mg/L) than towards Gram-negative (MICs = 1.2–4,000 mg/L, MBCs = 4.8–500 mg/L) bacteria, which suggests a higher susceptibility of Gram-positive strains to these agents. The higher resistance of Gram-negative bacteria to anti-inflammatory drugs may be due to the permeability barrier conferred by their outer membrane (Thangamani et al., 2015) and the presence of more effective multi-drug resistance efflux pump systems (Laudy, 2018; Quadros Barse et al., 2024). For example, the antimicrobial activity of celecoxib was restored when the outer membrane barrier was compromised with the antibiotic colistin (Thangamani et al., 2015). In another study, the deficiency of TolC proteins in the outer membrane of E. coli increased its susceptibility to auranofin (Quadros Barse et al., 2024). Additionally, deleting the AcrAB efflux pump in E. coli restored their susceptibility to celecoxib treatment (Thangamani et al., 2015).
2.2 In vivo experiments
Preclinical research with animal models has been the gold standard for decades, and it is an important criterion for determining the safety and efficacy of drugs before introducing them to the market (Mahalmani et al., 2023). However, it has also been observed that effects found in animal models cannot always be translated to the clinic (Martic-Kehl et al., 2012), thereby questioning the relevance of preclinical studies. For example, Langley (2009) stated that less than 50% of animal studies predicted human outcomes sufficiently. Additionally, animal models are poor predictors of drug safety in humans. Consequently, humans have been exposed to toxicity in the clinical testing of drugs that were believed to be safe in animal studies (Van Norman, 2019). For example, an analysis of 2, 366 drugs showed that results obtained from animal testing (e.g. rat, mouse and rabbit models) were inconsistent predictors of toxic responses in humans (Bailey et al., 2014). Since the drugs analysed in this review are currently in use in clinical settings, having undergone clinical trials and passing extensive toxicity and safety evaluations, they carry lower risks of adverse reactions (March-Vila et al., 2017). The in vivo studies were conducted to evaluate the antimicrobial effects of five anti-inflammatory drugs, namely auranofin, aspirin, ibuprofen, celecoxib, and diclofenac against seven pathogenic bacteria (C. difficile, E. faecium, E. faecalis, M. tuberculosis, P. aeruginosa, S. aureus, and S. enterica serotype Typhimurium) as well as the fungus Histoplasma capsulatum. These studies were performed in various infected mouse strains (BALB/c, C3HeB/FeJ, C57BL/6, CD1, and Swiss albino mice). Among the tested drugs, auranofin and ibuprofen were the most frequently assessed for their antimicrobial activity, while M. tuberculosis was the most commonly studied bacterial pathogen. Detailed data on the in vivo antimicrobial activity of these anti-inflammatory drugs are presented in Table 2.
2.2.1 Auranofin
Among all the anti-inflammatory drugs tested for in vivo antimicrobial effects, auranofin produced the effect at the lowest doses administered to the experimental animals. In the experiment with mice infected with vancomycin-resistant E. faecium (VRE), the animals showed a significantly higher survival rate (100%) compared to the control group (30%) when administered subcutaneously with 0.0625 mg/kg/day and orally with 0.125 mg/kg/day of auranofin (Abutaleb and Seleem, 2020a). In comparison, the subcutaneous administration of glycopeptide antibiotic teicoplanin at 40 mg/kg/day increased the survival rate only in two of five mice strains infected with VRE (Song et al., 2008). In another study performed with mice challenged with E. faecium, the animals experienced 98% and 99% reduction in bacterial burdens after oral treatment with 0.5 mg/kg/day of auranofin for 3 and 5 days, respectively. On the other hand, no reduction in infection was observed in the untreated control group. Interestingly, auranofin outperformed linezolid, a synthetic oxazolidinone antimicrobial drug approved for treating VRE infections, which failed to reduce the VRE burden in the mice within 3 days period and was only able to reduce bacterial burden by 52% after 5 days of treatment (Abdelkhalek et al., 2018). In another study, the antimicrobial action of auranofin was investigated against mice infected with S. aureus (Thangamani et al., 2016a). After administering 0.25 mg/kg/day of the drug orally to the mice, the animals recorded an 80% survival rate compared to <40% in the control group. Similarly, oral treatment with antibiotic linezolid achieved a high survival rate in mice. However, this was at a much higher dose of 25 mg/kg/day (Thangamani et al., 2016a). The intraperitoneal administration of 0.12 mg/kg/day and 0.012 mg/kg/day of auranofin to mice infected with S. aureus for 7 days only caused moderate to weak effects, with the animals showing 50% and 37% survival rates, respectively. All the animals in the control group died by day 4 (Harbut et al., 2015). Auranofin at low doses (0.125 mg/kg and 0.25 mg/kg) significantly protected mice against C. difficile infection (CDI) with a 100% and 80% survival rate. At these doses, the agent prevented CDI recurrence in the animals compared to vancomycin, which had a similar effect but at a higher dose of 10 mg/kg (Abutaleb and Seleem, 2020b). These results demonstrated auranofin’s strong in vivo antimicrobial effect against pathogenic bacteria such as S. aureus, E. faecium, and C. difficile. Moreover, evidence from toxicology studies shows that auranofin is safe. For example, no significant histopathologic lesions were observed on porcine skin after exposure to 1%, 2%, and 3% auranofin (topically) for 4–14 days. In addition, no systemic toxicity was observed in these pigs after exposure to this agent (Mohammad et al., 2021). Therefore, auranofin, an FDA-approved drug with a long history of clinical use, holds promise as a dual-action therapy with fewer side effects (Ito et al., 2022).
2.2.2 Ibuprofen
Shah et al. (2018) investigated the in vivo antimicrobial action of ibuprofen against mice infected with P. aeruginosa. After the oral administration of 0.75 mg/kg of ibuprofen, the animals showed a 92% survival rate compared to the control group (57%). In comparison, the intraperitoneal administration of either ceftazidime (1,000 mg/kg) or ciprofloxacin (100 mg/kg) every 8 h for 7 days to mice with P. aeruginosa infection resulted in 40%–60% animal survival (Song et al., 2012). When 80 mg/kg/day of the drug was orally given to the mice infected with M. tuberculosis, the animals experienced an 80% cure rate in their infected lungs. At the same time, the control group showed a 21% survival rate (Vilaplana et al., 2013). In addition, the oral administration of the combination of 20 mg/kg/day of ibuprofen with 150 mg/kg/day of pyrazinamide resulted in the eradication of M. tuberculosis infection from the spleen of 60% of the treated mice (Byrne et al., 2007).
2.2.3 Aspirin
The antimicrobial activity of aspirin alone or in combination with standard anti-TB drugs was investigated in an experimental murine model of acute TB. The treatment of TB-infected mice with 3 mg/kg/day of aspirin resulted in >90% of the animals surviving compared to about 70% in the control group within the same period (Kroesen et al., 2018). Similarly, mice treated with the standard antibiotic combination known as RIMSTAR also achieved a high survival rate in mice. Nevertheless, this was attained at high RIMSTAR doses comprising rifampicin 150 mg, isoniazid 75 mg, pyrazinamide 400 mg, and ethambutol 275 mg (Kroesen et al., 2018). In another study, mice infected with TB were investigated to determine the combined antimicrobial effect of anti-tuberculosis agent pyrazinamide and aspirin. This combination, which involved 20 mg/kg/day of aspirin and 150 mg/kg/day of pyrazinamide, completely eradicated TB infection from the spleen of 40% of the treated mice after 1 month of oral administration. When administered alone orally to mice infected with M. tuberculosis, pyrazinamide caused a reduction of infection in the lung and spleen of the mice at a dose of 150 mg/kg per day after 1 month of treatment (Byrne et al., 2007). Toxicity evaluations of aspirin revealed varying safety profiles across different animal species. For example, rats and mice exhibited different responses when fed diets containing aspirin at concentrations of 0, 0.3, 0.6, and 1.2% for one and 4 weeks. The rats group exhibited dose-dependent haemorrhagic anaemia and death, while no apparent signs of haemorrhage were observed in mice (Takahashi and Hiraga, 1985). The toxicity of aspirin to cats is due to the poor metabolism of the drug in these animals compared to humans (Bell, 2019). Unlike humans, cats lack the key enzymes responsible for aspirin clearance (Court, 2013). Despite this, aspirin remains widely used and, when taken correctly, offers significant benefits such as reducing the risk of heart attacks, stroke and blood flow disorders in individuals with cardiovascular disease (FDA, 2019).
2.2.4 Diclofenac
When M. tuberculosis-infected mice were treated with 10 mg/g of diclofenac (orally), they showed a 60% cure rate. A combined co-administration of diclofenac at 10 mg/g/day (orally) and streptomycin at 150 mg/g/day (subcutaneously) resulted in a 100% survival rate in these animals. In addition, this combination treatment regimen statistically caused significantly fewer bacteria in the lungs and spleen of these mice compared with those receiving streptomycin alone (Dutta et al., 2007a). This suggests that diclofenac synergistically enhances the efficacy of streptomycin. Also, a survival rate of 65% was attained when 15 mg/g/day of diclofenac was administered intraperitoneally to mice suffering from salmonella infection. Subsequent administration of this drug at higher doses of 30 and 60 mg/g/day increased the animal’s survival rate to 70% and 75%, respectively. In contrast, all the animals in the control group died (Dutta et al., 2007b).
2.2.5 Celecoxib
Celecoxib demonstrated in vivo antibacterial activity in a mouse model of MRSA skin infection. The topical application of 1 and 2% celecoxib cream (20 mg 2x/day) significantly reduced the bacterial burden in infected mice by 72% and 87%, respectively (Thangamani et al., 2015). Besides their antibacterial activity, celecoxib also demonstrated anti-fungal action by causing a 70% survival rate in mice with lethal H. capsulatum infection after oral treatment with 1 mg/kg/0.5/day of celecoxib (Pereira et al., 2013). Moreover, preclinical animal studies suggest that celecoxib has a favourable safety profile in rats, as it did not cause gastrointestinal mucosal damage (Altinkaynak et al., 2003; Li et al., 2003). Also, it is well tolerated in humans for long-term oral use with fewer gastrointestinal side effects compared to traditional NSAIDs (Kishore et al., 2016; Sozer et al., 2011). Nevertheless, there has been reported exacerbation of inflammation-associated colonic injury in rats treated with celecoxib (Zhang et al., 2004). Additionally, the agent has been linked with an elevated risk of heart-related conditions in humans, especially when taken at substantially high doses beyond those recommended for arthritis treatment (FitzGerald, 2003; Howes, 2007).
2.3 Clinical trials
In general, the number of clinical trials on the antimicrobial effects of anti-inflammatory drugs is very low and available studies are focused mainly on uncomplicated UTIs. NSAIDs, namely aceclofenac, diclofenac, and ibuprofen, were tested in clinical trials with patients diagnosed with uncomplicated UTIs and cellulitis (Gagyor et al., 2015; Bleidorn et al., 2010; Vik et al., 2018; Dall et al., 2005; Davis et al., 2017; Kronenberg et al., 2017; Ko et al., 2018). Among these drugs, ibuprofen was the most frequently tested in double-blind, randomised, controlled trials with UTI patients. Detailed data on the efficacy of anti-inflammatory drugs against UTIs and cellulitis are summarised in Table 3.
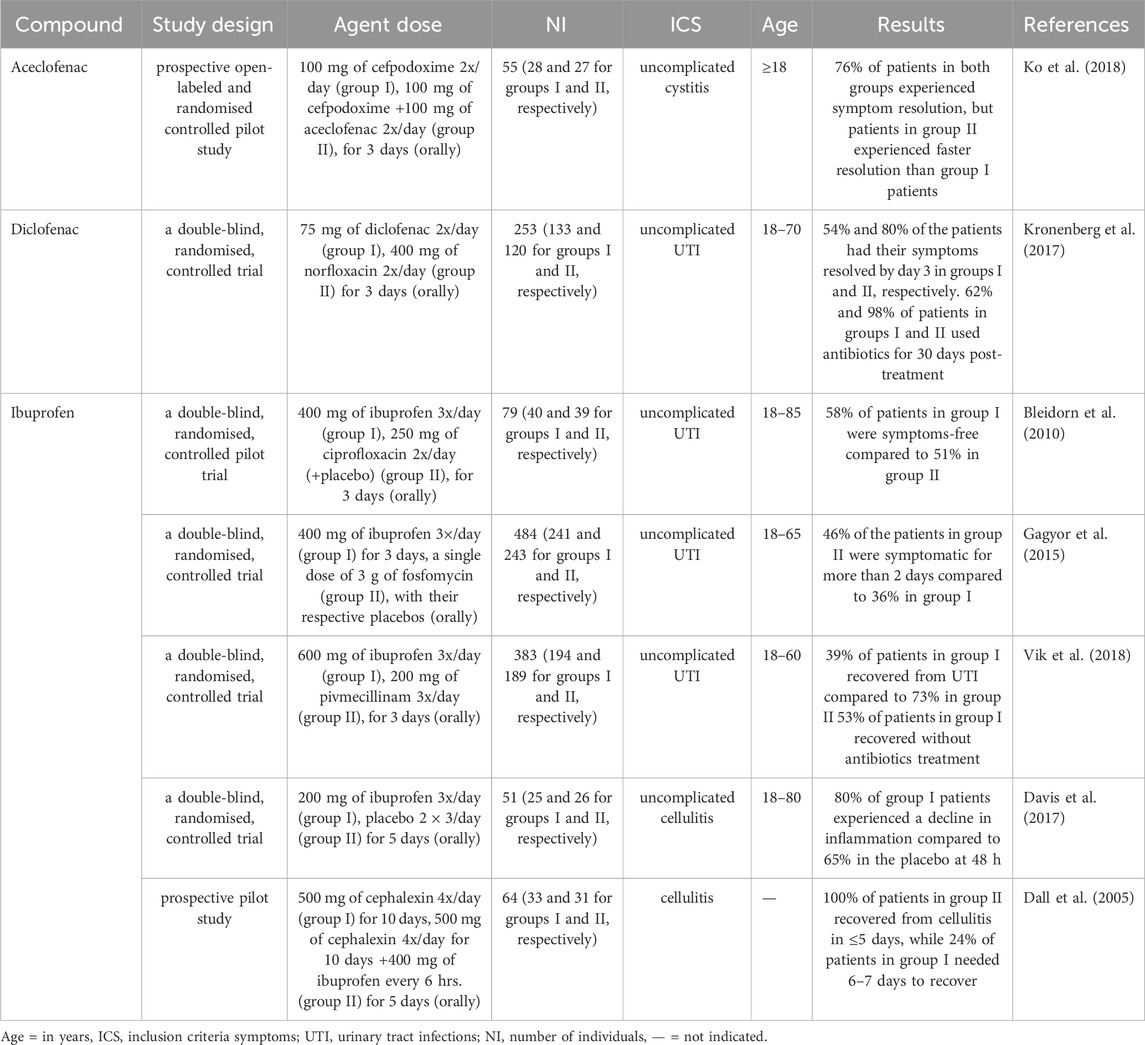
Table 3. Clinical trials demonstrating the effectiveness of anti-inflammatory drugs in symptom resolution among UTI and cellulitis patients.
Primary care physicians usually prescribe antibiotics for treating uncomplicated UTIs (Butler et al., 2017; Fahey et al., 2003), but NSAIDs have also been recommended as a first-line treatment option in women aged <65 years with suspected uncomplicated lower UTIs who experienced mild symptoms and as an alternative to antibiotics when the symptoms are moderate to severe (Scottish Intercollegiate Guidelines Network, 2020). Consequently, ibuprofen has been recommended for pain management and symptom relief in patients with lower UTI, such as cystitis (National Institute for Health and Care Excellence, 2018; Bettcher et al., 2021). Correspondingly, ibuprofen is also the most studied NSAID in clinical trials, resulting in positive outcomes for UTI patients. For example, the administration of 400 mg 3x/day of this agent to UTI patients for 3 days resulted in 58% and 75% rates in symptom resolution by days 4 and 7, respectively, compared to 51% and 60% in those treated with 250 mg of ciprofloxacin 2x/day (Bleidorn et al., 2010). In another study, only a lesser percentage (36%) of patients administered 400 mg of ibuprofen 3x/day for 3 days experienced UTI symptoms over 2 days compared with 46% of those treated with a single dose of 3 g fosfomycin. Also, cases of recurrent UTI were reported more (23%) by women assigned to the fosfomycin group compared to the ibuprofen group with 17%. Additionally, the initial treatment of patients with ibuprofen caused a 67% reduction in antibiotic use. However, women using ibuprofen were more likely to report a higher burden of symptoms over the first 7 days after the start of their treatment compared with those using only fosfomycin (Gagyor et al., 2015). Although ibuprofen at 600 mg 3x/day was inferior to pivmecillinam at 200 mg 3x/day in treating uncomplicated UTIs, 53% of the patients treated with ibuprofen recovered without antibiotic use after 4 weeks of follow-up (Vik et al., 2018). Also, ibuprofen seems relatedly safe in animal models. For example, a study on the gastrointestinal and renal safety of piglets administered with 5 mg/kg of ibuprofen 3x/day for 5 days demonstrated that this agent was well-tolerated because no severe lesions or significant histological changes were detected in their stomach or kidneys (Millecam et al., 2019). However, caution is advised when using ibuprofen, as its administration may lead to a dose-dependent increase in gastrointestinal permeability (Khazaeinia and Jamali, 2000). Thus, as a non-COX inhibitor, it can compromise gastric mucosal integrity, potentially leading to intramucosal haemorrhages, though this effect is typically observed with chronic use (Ershad et al., 2024).
A separate study investigating the superiority of 75 mg 2x/day diclofenac over 400 mg 2x/day norfloxacin in UTI patients after 3 days showed that diclofenac was less effective than norfloxacin; however, those patients on diclofenac were 37% less likely to receive antibiotic treatment until day 30 post-randomisation (Kronenberg et al., 2017). Antibiotics used by UTI patients account for 10%–20% of all the antibiotics prescribed in ambulatory care (Melnyk et al., 2024). Furthermore, many studies have shown that there is a clear Incorrelation between antibiotic consumption and increased drug resistance in uropathogens such as E.coli Therefore, reducing the prescription and use of antibiotics for UTI treatment could help reduce the risk of antibiotic resistance in the population (Kronenberg et al., 2017). When considering side effects, diclofenac is generally safe, with its benefits outweighing potential risks. For example, it is well-tolerated for topical treatment of musculoskeletal conditions (European Medicine Agency, 2013; Taylor et al., 2011). Nevertheless, animal studies show evidence of toxicity, including gastric damage in rats at high doses (Aycan et al., 2018). Also, in humans, there is a small risk of heart attack or stroke with prolonged systemic exposure to high doses (150 mg daily) of this agent (European Medicine Agency, 2013). Another study evaluated the efficacy of combining 100 mg of aceclofenac (an analogue of diclofenac) 2x/day with 100 mg of cefpodoxime for the treatment of uncomplicated cystitis (a form of UTI) compared to 100 mg of cefpodoxime 2x/day for 3 days. Results showed that 76% of the patients in both groups experienced symptom resolution. However, patients in the combination group had a faster symptom resolution than those administered with cefpodoxime alone (Ko et al., 2018).
Cellulitis is a diffuse spreading infection with inflammation of the deeper dermis and subcutaneous fat. It is caused mainly by S. aureus (Horseman and Bowman, 2013). A study that compared the efficacy of 200 mg 3x/day of ibuprofen to a placebo 2 × 3/day for 5 days in treating uncomplicated cellulitis showed 80% inflammation regression in the ibuprofen-treated group compared to 65% in the placebo. (Davis et al., 2017). Additionally, administering a combination of 400 mg of ibuprofen every 6 hours for 5 days and 500 mg of cephalexin 4x/day for 10 days led to a total recovery of all the patients from cellulitis infection in 4–5 days. In contrast, only about 24% of patients treated with 500 mg of cephalexin 4x/day for 10 days recovered, which took 6–7 days, while 6% required 7 days or more to recover (Dall et al., 2005). Overall, these agents appear effective in alleviating symptoms in UTI patients.
3 Conclusion
In conclusion, the literature analysis of in vitro, in vivo and clinical data identified 18 anti-inflammatory drugs with antibacterial activities. Among three drugs (aceclofenac, diclofenac and ibuprofen) studied in clinical trials, ibuprofen was the most frequently tested agent, and its administration resulted in a higher rate of symptom resolution and reduced antibiotic use in UTI patients. Although there is no clear evidence about its antimicrobial effect from clinical trials, in vivo results showed that ibuprofen at a low dose significantly increased the survival rate in mice infected with P. aeruginosa. It also demonstrated potent in vitro growth and inhibitory effects against B. cereus, E. coli, MRSA and MSSA. For the remaining 15 drugs (auranofin, aspirin, bromfenac, carprofen, celecoxib, diacerein, flufenamic acid, flurbiprofen, indomethacin, meclofenamic acid, naproxen, nimesulide, sodium salicylate, tolfenamic acid and vedaprofen), only data from in vitro and in vivo experiments are available. Among these, auranofin caused a significantly higher survival rate in mice infected with vancomycin-resistant E. faecium, S. aureus and C. difficile. It also produced a strong in vitro growth-inhibitory effect against S. agalactiae, S. pneumoniae, S. aureus, S. epidermidis, B. subtilis, C. difficile, E. faecalis, E. faecium, and M. tuberculosis. Among other antimicrobially active anti-inflammatory drugs, aspirin showed a significant effect in vivo against M. tuberculosis and strong to moderate in vitro activity against E. coli, B. cereus, P. aeruginosa, E. aerogenes, K. pneumoniae and S. choleraesuis. Similarly, celecoxib produced significant in vivo effects when applied topically against MRSA but only moderate in vitro effects against S. epidermidis, S. aureus and B. subitilis. These data suggest that certain NSAIDs are promising drug candidates for the development of dual-action drugs for the potential treatment of TB, MSKIs, and UTIs. However, future clinical trials are required to evaluate the antibacterial efficacy of anti-inflammatory drugs in treating TB, MSKIs, and UTIs before they can be integrated into clinical practice. Additionally, future research is needed to investigate their dual mechanism of action—combining anti-inflammatory and antimicrobial effects. Moreover, exploring targeted drug delivery systems for these agents could further enhance their therapeutic potential by minimising systemic side effects or off-target exposure, especially in the treatment of complex infectious and inflammatory diseases such as osteomyelitis.
Author contributions
OO: Data curation, Formal Analysis, Investigation, Writing – original draft, Writing – review and editing. JR: Investigation, Writing – original draft, Writing – review and editing. LK: Conceptualization, Supervision, Writing – original draft, Writing – review and editing.
Funding
The author(s) declare that financial support was received for the research and/or publication of this article. This work was financially supported by the Internal Grant Agency of the Faculty of Tropical AgriSciences (grant no. IGA 20243109).
Conflict of interest
The authors declare that the research was conducted in the absence of any commercial or financial relationships that could be construed as a potential conflict of interest.
The author(s) declared that they were an editorial board member of Frontiers, at the time of submission. This had no impact on the peer review process and the final decision.
Generative AI statement
The author(s) declare that no Generative AI was used in the creation of this manuscript.
Publisher’s note
All claims expressed in this article are solely those of the authors and do not necessarily represent those of their affiliated organizations, or those of the publisher, the editors and the reviewers. Any product that may be evaluated in this article, or claim that may be made by its manufacturer, is not guaranteed or endorsed by the publisher.
References
Abbas, H. A., Atallah, H., El-Sayed, M. A., and El-Ganiny, A. M. (2020). Diclofenac mitigates virulence of multidrug-resistant Staphylococcus aureus. Arch. Microbiol. 202, 2751–2760. doi:10.1007/s00203-020-01992-y
Abdel-Aziz, M. I., Ali, M. A., Hassan, A. K., and Elfaham, T. H. (2016). Warfarin-drug interactions: an emphasis on influence of polypharmacy and high doses of amoxicillin/clavulanate. J. Clin. Pharmacol. 56, 39–46. doi:10.1002/jcph.583
AbdelKhalek, A., Abutaleb, N. S., Elmagarmid, K. A., and Seleem, M. N. (2018). Repurposing auranofin as an intestinal decolonizing agent for vancomycin-resistant enterococci. Sci. Rep. 8, 8353. doi:10.1038/s41598-018-26674-0
AbdelKhalek, A., Abutaleb, N. S., Mohammad, H., and Seleem, M. N. (2019). Antibacterial and anti-virulence activities of auranofin against Clostridium difficile. Int. J. Antimicrob. Agents 53, 54–62. doi:10.1016/j.ijantimicag.2018.09.018
Abraham, S., Sharon, N., and Ofek, I. (1999). “Adhesion of bacteria to mucosal surfaces,” in Mucosal immunology. Editors P. L. Ogra, M. J. Mestecky, M. E. Lamm, W. Strober, J. Bienenstock, and J. R. McGhee (San Diego: Academic Press).
Abraham, S., Shin, J., and Malaviya, R. (2001). Type 1 fimbriated Escherichia coli-mast cell interactions in cystitis. J. Infect. Dis. 183 (Suppl. 1), S51–S55. doi:10.1086/318853
Abutaleb, N. S., and Seleem, M. N. (2020a). Anti-virulence activity of auranofin against vancomycin-resistant enterococci: in vitro and in vivo studies. Int. J. Antimicrob. Agents 55, 105828. doi:10.1016/j.ijantimicag.2019.10.009
Abutaleb, N. S., and Seleem, M. N. (2020b). Auranofin, at a clinically achievable dose, protects mice and prevents recurrence from Clostridioides difficile infection. Sci. Rep. 10, 7701. doi:10.1038/s41598-020-64882-9
Al-Bakri, A. G., Othman, G., and Bustanji, Y. (2009). The assessment of the antibacterial and antifungal activities of aspirin, EDTA and aspirin-EDTA combination and their effectiveness as antibiofilm agents. J. Appl. Microbiol. 107, 280–286. doi:10.1111/j.1365-2672.2009.04205.x
Al-Janabi, A. A. (2010). In vitro antibacterial activity of ibuprofen and acetaminophen. J. Glob. Infect. Dis. 2, 105–108. doi:10.4103/0974-777X.62880
Altinkaynak, K., Suleyman, H., and Akçay, F. (2003). Effect of nimesulide, rofecoxib and celecoxib on gastric tissue glutathione level in rats with indomethacin-induced gastric ulcerations. Pol. J. Pharmacol. 55, 645–648.
Alves de Lima e Silva, A., Viana, A. S., Silva, P. M., de Matos Nogueira, E., Salgado, L. T., Tomazetto de Carvalho, R., et al. (2021). Diclofenac may induce PIA-independent biofilm formation in Staphylococcus aureus strains. Int. Microbiol. 2021, 1–11. doi:10.1155/2021/8823775
Amgen (2024). Amgen reports fourth quarter and full year 2024 financial results. Available online at: https://www.amgen.com/newsroom/press-releases/2025/02/amgen-reports-fourth-quarter-and-full-year-2024-financial-results (Accessed February 26, 2025).
Amgen Canada Inc (2022). Romosozumab (evenity): CADTH reimbursement review: therapeutic area: osteoporosis. Available online at: https://www.cda-amc.ca/sites/default/files/DRR/2022/SR0676-Evenity-combined-report.pdf (Accessed February 18, 2025).
Arkader, A., Brusalis, C., Warner, W. C., Conway, J. H., and Noonan, K. (2016). Update in pediatric musculoskeletal infections: when it is, when it isn't, and what to do. Am. Acad. Orthop. Surg. 24, e112–e121. doi:10.5435/JAAOS-D-15-00714
Ashburn, T. T., and Thor, K. B. (2004). Drug repositioning: identifying and developing new uses for existing drugs. Nat. Rev. Drug Discov. 3, 673–683. doi:10.1038/nrd1468
Aycan, I. O., Elpek, O., Akkaya, B., Kıraç, E., Tuzcu, H., Kaya, S., et al. (2018). Diclofenac-induced gastrointestinal and renal toxicity is alleviated by thymoquinone treatment. Food Chem. Toxicol. 118, 795–804. doi:10.1016/j.fct.2018.06.038
Bailey, J., Thew, M., and Balls, M. (2014). An analysis of the use of animal models in predicting human toxicology and drug safety. Altern. Lab. Anim. 42, 181–199. doi:10.1177/026119291404200306
Ballard, C. G. (2002). Advances in the treatment of Alzheimer's disease: benefits of dual cholinesterase inhibition. Eur. Neurol. 47, 64–70. doi:10.1159/000047952
Bausch and Lomb (2022). 4Q22 and FY22 financial results: FY22 financial highlights and segment drivers. Available online at: https://ir.bausch.com/sites/bauschhealth-ir/files/2023-02/4q22-bausch-lomb-earnings-presentation.pdf (Accessed February 18, 2025).
Beck-Broichsitter, B. E., Smeets, R., and Heiland, M. (2015). Current concepts in pathogenesis of acute and chronic osteomyelitis. Curr. Opin. Infect. Dis. 8, 240–245. doi:10.1097/QCO.0000000000000155
Bell, J. (2019). Aspirin killed the cat: animal research models do not always apply to humans. Expert Opin. Drug Metab. Toxicol. 15, 683–685. doi:10.1080/17425255.2019.1652596
Bettcher, C. M., Campbell, E., Petty, L. A., Rew, K. T., Zelnik, J. C., Lane, G. I., et al. (2021). Urinary tract infection. Michigan, United States Michigan Medicine University of Michigan. Available online at: https://www.ncbi.nlm.nih.gov/books/NBK572335/ (Accessed February 24, 2025)
Bindu, S., Mazumder, S., and Bandyopadhyay, U. (2020). Non-steroidal anti-inflammatory drugs (NSAIDs) and organ damage: a current perspective. Biochem. Pharmacol. 180, 114147. doi:10.1016/j.bcp.2020.114147
Blanco-Cabra, N., Paetzold, B., Ferrar, T., Mazzolini, R., Torrents, E., Serrano, L., et al. (2020). Characterisation of different alginate lyases for dissolving Pseudomonas aeruginosa biofilms. Sci. Rep. 10, 9390. doi:10.1038/s41598-020-66293-2
Bleidorn, J., Gagyor, I., Kochen, M. M., Wegscheider, K., and Hummers-Pradier, E. (2010). Symptomatic treatment (ibuprofen) or antibiotics (ciprofloxacin) for uncomplicated urinary tract infection? results of a randomised controlled pilot trial. BMC Med. 8, 30. doi:10.1186/1741-7015-8-30
Brown, D., and Superti-Furga, G. (2003). Rediscovering the sweet spot in drug discovery. Drug Discov. Today 8, 1067–1077. doi:10.1016/s1359-6446(03)02902-7
Bruneton, J. (1999). Quinones. Pharmacognosy, phytochemistry, medicinal plants. 2nd ed. Paris, Lavoisier, 409–444.
Bruniera, F. R., Ferreira, F. M., Saviolli, L. R., Bacci, M. R., Feder, D., da Luz Goncalves Pedreira, M., et al. (2015). The use of vancomycin with its therapeutic and adverse effects: a review. Eur. Rev. Med. Pharmacol. Sci. 19, 694–700.
Butler, C. C., Francis, N., Thomas-Jones, E., Lloret, C., Bongard, E., Moore, M., et al. (2017). Variations in presentation, management, and patient outcomes of urinary tract infection: a prospective four-country primary care observational cohort study. Br. J. Gen. Pract. 67, e830–e841. doi:10.3399/bjgp17X693641
Byrne, S. T., Denkin, S. M., and Zhang, Y. (2007). Aspirin and ibuprofen enhance pyrazinamide treatment of murine tuberculosis. J. Antimicrob. Chemother. 59, 313–316. doi:10.1093/jac/dkl486
Carr, W., Kurbatova, E., Starks, A., Goswami, N., Allen, L., and Winston, C. (2022). Interim guidance: 4-month rifapentine-moxifloxacin regimen for the treatment of drug-susceptible pulmonary tuberculosis - United States, 2022. MMWR Morb. Mortal. Wkly. Rep. 71, 285–289. doi:10.15585/mmwr.mm7108a1
Cassetta, M. I., Marzo, T., Fallani, S., Novelli, A., and Messori, L. (2014). Drug repositioning: auranofin as a prospective antimicrobial agent for the treatment of severe staphylococcal infections. Biometals 27, 787–791. doi:10.1007/s10534-014-9743-6
Cavalli, A., Bolognesi, M. L., Minarini, A., Rosini, M., Tumiatti, V., Recanatini, M., et al. (2008). Multi-target-directed ligands to combat neurodegenerative diseases. J. Med. Chem. 51, 347–372. doi:10.1021/jm7009364
Chan, E. W. L., Yee, Z. Y., Raja, I., and Yap, J. K. Y. (2017). Synergistic effect of non-steroidal anti-inflammatory drugs (NSAIDs) on antibacterial activity of cefuroxime and chloramphenicol against methicillin-resistant Staphylococcus aureus. J. Glob. Antimicrob. Resist 10, 70–74. doi:10.1016/j.jgar.2017.03.012
Chen, Q., Ilanga, M., Simbassa, S. B., Chirra, B., Shah, K. N., and Cannon, C. L. (2023). Synergistic antimicrobial effects of ibuprofen combined with standard-of-care antibiotics against cystic fibrosis pathogens. Biomedicines 11, 2936. doi:10.3390/biomedicines11112936
Chen, Z., Cheng, L., and Feng, G. (2018). Bone inflammation and chronic recurrent multifocal osteomyelitis. Eur. Rev. Med. Pharmacol. Sci. 22, 1380–1386. doi:10.26355/eurrev_201803_14482
Chiaverini, L., Pratesi, A., Cirri, D., Nardinocchi, A., Tolbatov, I., Marrone, A., et al. (2022). Anti-staphylococcal activity of the auranofin analogue bearing acetylcysteine in place of the thiosugar: an experimental and theoretical investigation. Molecules 27, 2578. doi:10.3390/molecules27082578
Chiu, H. C., Lee, S. L., Kapuriya, N., Wang, D., Chen, Y. R., Yu, S. L., et al. (2012). Development of novel antibacterial agents against methicillin-resistant Staphylococcus aureus. Bioorg Med. Chem. 20, 4653–4660. doi:10.1016/j.bmc.2012.06.018
Chiu, H. C., Yang, J., Soni, S., Kulp, S. K., Gunn, J. S., Schlesinger, L. S., et al. (2009). Pharmacological exploitation of an off-target antibacterial effect of the cyclooxygenase-2 inhibitor celecoxib against Francisella tularensis. Antimicrob Agents Chemother. Antimicrob. Agents Chemother. 53, 2998–3002. doi:10.1128/AAC.00048-09
Chowdhury, B., Adak, M., and Bose, S. K. (2003). Flurbiprofen, a unique non-steroidal anti-inflammatory drug with antimicrobial activity against Trichophyton, Microsporum and Epidermophyton species. Antimicrob. Agents Chemother. 64, 158–161. doi:10.1046/j.1472-765x.2003.01370.x
Chung, H. (2016). The association between chronic inflammation and recurrent cystitis in women. Urogenit. Tract. Infect. 11, 86–92. doi:10.14777/uti.2016.11.3.86
Colgan, R., and Williams, M. (2011). Diagnosis and treatment of acute uncomplicated cystitis. Am. Fam. Physician 84, 771–776.
Colston, J., and Atkins, B. (2018). Bone and joint infection. Clin. Med. (Lond). 18, 150–154. doi:10.7861/clinmedicine.18-2-150
Copley, L. A. (2009). Pediatric musculoskeletal infection: trends and antibiotic recommendations. J. Am. Acad. Orthop. Surg. 17, 618–626. doi:10.5435/00124635-200910000-00004
Court, M. H. (2013). Feline drug metabolism and disposition: pharmacokinetic evidence for species differences and molecular mechanisms. Vet. Clin. North Am. Small Anim. Pract. 43, 1039–1054. doi:10.1016/j.cvsm.2013.05.002
Cramton, S. E., Gerke, C., Schnell, N. F., Nichols, W. W., and Gotz, F. (1999). The intercellular adhesion (ica) locus is present in Staphylococcus aureus and is required for biofilm formation. Infect. Immun. 67, 5427–5433. doi:10.1128/IAI.67.10.5427-5433.1999
Dai, L., Wu, T. Q., Xiong, Y. S., Ni, H. B., Ding, Y., Zhang, W. C., et al. (2019). Ibuprofen-mediated potential inhibition of biofilm development and quorum sensing in Pseudomonas aeruginosa. Life Sci. 237, 116947. doi:10.1016/j.lfs.2019.116947
Dall, L., Peterson, S., Simmons, T., and Dall, A. (2005). Rapid resolution of cellulitis in patients managed with combination antibiotic and anti-inflammatory therapy. Cutis 75, 177–180.
Dang, Y., and Guan, J. (2020). Nanoparticle-based drug delivery systems for cancer therapy. Smart Mater Med. 1, 10–19. doi:10.1016/j.smaim.2020.04.001
Dastidar, S. G., Ganguly, K., Chaudhuri, K., and Chakrabarty, A. N. (2000). The anti-bacterial action of diclofenac shown by inhibition of DNA synthesis. Int. J. Antimicrob. Agents 14, 249–251. doi:10.1016/s0924-8579(99)00159-4
Davies, N. M. (1998). Clinical pharmacokinetics of ibuprofen. The first 30 years. Clin. Pharmacokinet. 34, 101–154. doi:10.2165/00003088-199834020-00002
Davis, A., Fassassi, C., Dove, D., Drapkin, J., Likourezos, A., Gohel, A., et al. (2022). Analgesic efficacy of oral aspirin/ketamine combination for management of acute musculoskeletal pain in the emergency department - a proof-of-concept pilot study. J. Emerg. Med. 62, 750–759. doi:10.1016/j.jemermed.2022.01.029
Davis, J. S., Mackrow, C., Binks, P., Fletcher, W., Dettwiller, P., Marshall, C., et al. (2017). A double-blind randomized controlled trial of ibuprofen compared to placebo for uncomplicated cellulitis of the upper or lower limb. Clin. Microbiol. Infect. 23, 242–246. doi:10.1016/j.cmi.2017.02.036
de Matos, R. F., Mendonca, L. C. V., da Silva Souza, K. G., Fonseca, A. A. D., Costa, E. M. S., de Lima, M. V. D., et al. (2017). Nimesulide inhibits pathogenic fungi: PGE2-dependent mechanisms. Folia Microbiol. (Praha) 62, 169–174. doi:10.1007/s12223-016-0483-6
Demirag, M. K., Esen, S., Zivalioglu, M., Leblebicioglu, H., and Keceligil, H. T. (2007). The effect of aspirin on adherence of slime-producing, coagulase-negative staphylococci to vascular grafts. Ann. Vasc. Surg. 21, 464–467. doi:10.1016/j.avsg.2006.06.006
Dinescu, S. C., Barbulescu, A. L., Firulescu, S. C., Chisalau, A. B., Parvanescu, C. D., Ciurea, P. L., et al. (2021). Staphylococcus aureus-induced septic arthritis of the ankle related to malum perforans in a diabetes patient. Rom. J. Morphol. Embryol. 62, 615–619. doi:10.47162/RJME.62.2.31
Dogan, A., Otlu, S., Celebi, O., Aksu, P., Saglam, A. G., Dogan, A. N. C., et al. (2017). An investigation of antibacterial effects of steroids. Turk. J. Vet. Anim. Sci. 41, 22. doi:10.3906/vet-1510-24
Domingos, O. D. S., Alcantara, B. G. V., Santos, M. F. C., Maiolini, T. C. S., Dias, D. F., Baldim, J. L., et al. (2019). Anti-inflammatory derivatives with dual mechanism of action from the metabolomic screening of Poincianella pluviosa. Molecules 24, 4375. doi:10.3390/molecules24234375
Dutta, N. K., Annadurai, S., Mazumdar, K., Dastidar, S. G., Kristiansen, J., Ec Molnar, J., et al. (2007b). Potential management of resistant microbial infections with a novel non-antibiotic: the anti-inflammatory drug diclofenac sodium. Int. J. Antimicrob. Agents 30, 242–249. doi:10.1016/j.ijantimicag.2007.04.018
Dutta, N. K., Mazumdar, K., Dastidar, S. G., and Park, J. H. (2007a). Activity of diclofenac used alone and in combination with streptomycin against Mycobacterium tuberculosis in mice. Int. J. Antimicrob. Agents 30, 336–340. doi:10.1016/j.ijantimicag.2007.04.016
Elmesseri, R. A., Saleh, S. E., Ghobish, S. A., Majrashi, T. A., Elsherif, H. M., and Aboshanab, K. M. (2023). Diclofenac and meloxicam exhibited anti-virulence activities targeting staphyloxanthin production in methicillin-resistant Staphylococcus aureus. Antibiot. (Basel) 12, 277. doi:10.3390/antibiotics12020277
El-Mowafy, S. A., Abd El Galil, K. H., El-Messery, S. M., and Shaaban, M. I. (2014). Aspirin is an efficient inhibitor of quorum sensing, virulence and toxins in Pseudomonas aeruginosa. Microb. Pathog. 74, 25–32. doi:10.1016/j.micpath.2014.07.008
Ershad, M., Ameer, M. A., Chen, R. J., and Vearrier, D. (2024). “Ibuprofen toxicity,” in StatPearls (Florida, United States StatPearls Publishing). Available online at: https://www.ncbi.nlm.nih.gov/books/NBK526078/(Accessed February 23, 2025).
Esmail, A., Sabur, N. F., Okpechi, I., and Dheda, K. (2018). Management of drug-resistant tuberculosis in special sub-populations, including those with HIV co-infection, pregnancy, diabetes, organ-specific dysfunction, and in the critically ill. J. Thorac. Dis. 10, 3102–3118. doi:10.21037/jtd.2018.05.11
European Medicine Agency (2013). New safety advice for diclofenac–CMDh endorses PRAC recommendation. Available online at: https://www.ema.europa.eu/en/news/new-safety-advice-diclofenac-cmdh-endorses-prac-recommendation (Accessed February 22, 2025).
Fahey, T., Webb, E., Montgomery, A. A., and Heyderman, R. S. (2003). Clinical management of urinary tract infection in women: a prospective cohort study. Fam. Pract. 20, 1–6. doi:10.1093/fampra/20.1.1
Farrell, B., Pottie, K., Thompson, W., Boghossian, T., Pizzola, L., Rashid, F. J., et al. (2017). Deprescribing proton pump inhibitors: evidence-based clinical practice guideline. Can. Fam. Physician 63, 354–364.
FDA (2019). Aspirin for reducing your risk of heart attack and stroke: know the facts: information on using aspirin daily, over-the-counter, with other medicines, as well as its side effects. Available online at: https://www.fda.gov/drugs/safe-use-aspirin/aspirin-reducing-your-risk-heart-attack-and-stroke-know-facts (Accessed February 23, 2025).
Ferrara, G., Petrillo, M. G., Giani, T., Marrani, E., Filippeschi, C., Oranges, T., et al. (2019). Clinical use and molecular action of corticosteroids in the pediatric age. Int. J. Mol. Sci. 20, 444. doi:10.3390/ijms20020444
Ferretti, C., Chiaverini, L., Poma, N., Dalli, A., Di Leo, R., Rindi, L., et al. (2025). Antimicrobial and antibiofilm activity of auranofin and its two derivatives bearing naproxen and acetylcysteine as ligands against Staphylococci. Antibiotics 14, 118. doi:10.3390/antibiotics14020118
Fidelix, T. S., Macedo, C. R., Maxwell, L. J., and Fernandes Moca Trevisani, V. (2014). Diacerein for osteoarthritis. Cochrane Database Syst. Rev. 2014, CD005117. doi:10.1002/14651858.CD005117.pub3
Field, T. S., Gurwitz, J. H., Harrold, L. R., Rothschild, J., DeBellis, K. R., Seger, A. C., et al. (2004). Risk factors for adverse drug events among older adults in the ambulatory setting. J. Am. Geriatr. Soc. 52, 1349–1354. doi:10.1111/j.1532-5415.2004.52367.x
FitzGerald, G. A. (2003). COX-2 and beyond: approaches to prostaglandin inhibition in human disease. Nat. Rev. Drug Discov. 2, 879–890. doi:10.1038/nrd1225
Flores-Mireles, A. L., Walker, J. N., Caparon, M., and Hultgren, S. J. (2015). Urinary tract infections: epidemiology, mechanisms of infection and treatment options. Nat. Rev. Microbiol. 13, 269–284. doi:10.1038/nrmicro3432
Gagyor, I., Bleidorn, J., Kochen, M. M., Schmiemann, G., Wegscheider, K., and Hummers-Pradier, E. (2015). Ibuprofen versus fosfomycin for uncomplicated urinary tract infection in women: randomised controlled trial. BMJ 351, h6544. doi:10.1136/bmj.h6544
Gajdacs, M., and Spengler, G. (2019). The role of drug repurposing in the development of novel antimicrobial drugs: non-antibiotic pharmacological agents as quorum sensing-inhibitors. Antibiot. (Basel) 8, 270. doi:10.3390/antibiotics8040270
Ghofrani, H. A., Osterloh, I. H., and Grimminger, F. (2006). Sildenafil: from angina to erectile dysfunction to pulmonary hypertension and beyond. Nat. Rev. Drug Discov. 5, 689–702. doi:10.1038/nrd2030
Gomez-Acebo, I., Dierssen-Sotos, T., de Pedro, M., Perez-Gomez, B., Castano-Vinyals, G., Fernández-Villa, T., et al. (2018). Epidemiology of non-steroidal anti-inflammatory drugs consumption in Spain. The MCC-Spain study. BMC public health 18, 1134. doi:10.1186/s12889-018-6019-z
Gustafson, J. E., Candelaria, P. V., Fisher, S. A., Goodridge, J. P., Lichocik, T. M., McWilliams, T., et al. (1999). Growth in the presence of salicylate increases fluoroquinolone resistance in Staphylococcus aureus. Antimicrob. Agents Chemother. 43, 990–992. doi:10.1128/AAC.43.4.990
Hamed, M. M., El-Dean, A. M., Abdel-Mohsen, S. A., and Tolba, M. S. (2021). New diclofenac derivatives as anti-microbial, anti-inflammatory agents: design, synthesis, biological screening, and molecular docking study. Russ. J. Bioorg Chem. 47, 208–220. doi:10.1134/S1068162021010088
Hannan, T. J., Totsika, M., Mansfield, K. J., Moore, K. H., Schembri, M. A., and Hultgren, S. J. (2012). Host-pathogen checkpoints and population bottlenecks in persistent and intracellular uropathogenic Escherichia coli bladder infection. FEMS Microbiol. Rev. 36, 616–648. doi:10.1111/j.1574-6976.2012.00339.x
Harbut, M. B., Vilcheze, C., Luo, X., Hensler, M. E., Guo, H., Yang, B., et al. (2015). Auranofin exerts broad-spectrum bactericidal activities by targeting thiol-redox homeostasis. Proc. Natl. Acad. Sci. U. S. A. 112, 4453–4458. doi:10.1073/pnas.1504022112
Hogan, A., Heppert, V. G., and Suda, A. J. (2013). Osteomyelitis. Arch. Orthop. Trauma Surg. 133, 1183–1196. doi:10.1007/s00402-013-1785-7
Hooton, T. M. (2012). Clinical practice. Uncomplicated urinary tract infection. N. Engl. J. Med. 366, 1028–1037. doi:10.1056/NEJMcp1104429
Horseman, M., and Bowman, J. D. (2013). Is community-acquired methicillin-resistant Staphylococcus aureus coverage needed for cellulitis? Infect. Dis. Ther. 2, 175–185. doi:10.1007/s40121-013-0019-1
Howes, L. G. (2007). Selective COX-2 inhibitors, NSAIDs and cardiovascular events - is celecoxib the safest choice? Ther. Clin. Risk Manag. 3, 831–845.
Humphries, R. M., Pollett, S., and Sakoulas, G. A. (2013). A current perspective on daptomycin for the clinical microbiologist. Clin. Microbiol. Rev. 26, 759–780. doi:10.1128/CMR.00030-13
Ito, K., Nishida, Y., Hamada, S., Shimizu, K., Sakai, T., Ohkawara, B., et al. (2022). Efficacy of auranofin as an inhibitor of desmoid progression. Sci. Rep. 12, 11918. doi:10.1038/s41598-022-15756-9
Ivanyi, J., and Zumla, A. (2013). Nonsteroidal anti-inflammatory drugs for adjunctive tuberculosis treatment. J. Infect. Dis. 208, 185–188. doi:10.1093/infdis/jit153
Kalinka, J., Hachmeister, M., Geraci, J., Sordelli, D., Hansen, U., Niemann, S., et al. (2014). Staphylococcus aureus isolates from chronic osteomyelitis are characterized by high host cell invasion and intracellular adaptation, but still induce inflammation. Int. J. Med. Microbiol. 304, 1038–1049. doi:10.1016/j.ijmm.2014.07.013
Kamb, A., Wee, S., and Lengauer, C. (2007). Why is cancer discovery so difficult? Nat. Rev. Drug Discov. 6, 115–120. doi:10.1038/nrd2155
Kandiah, N., Pai, M. C., Senanarong, V., Looi, I., Ampil, E., Park, K. W., et al. (2017). Rivastigmine: the advantages of dual inhibition of acetylcholinesterase and butyrylcholinesterase and its role in subcortical vascular dementia and Parkinson's disease dementia. Clin. Interv. Aging 12, 697–707. doi:10.2147/CIA.S129145
Karin, M., Lawrence, T., and Nizet, V. (2006). Innate immunity gone awry: linking microbial infections to chronic inflammation and cancer. Cell 124, 823–835. doi:10.1016/j.cell.2006.02.016
Kavanagh, N., Ryan, E. J., Widaa, A., Sexton, G., Fennell, J., O'Rourke, S., et al. (2018). Staphylococcal osteomyelitis: disease progression, treatment challenges, and future directions. Clin. Microbiol. Rev. 31, 000844-17. doi:10.1128/CMR.00084-17
Khazaeinia, T., and Jamali, F. (2000). Evaluation of gastrointestinal toxicity of ibuprofen using surrogate markers in rats: effect of formulation and route of administration. Clin. Exp. Rheumatol. 18, 187–192.
Kishore, N., Raja, M. D., Kumar, C. S., Dhanalekshmi, U., and Srinivasan, R. (2016). Lipid carriers for delivery of celecoxib: in vitro, in vivo assessment of nanomedicine in rheumatoid arthritis. Eur. J. Lipid Sci. Technol. 118, 949–958. doi:10.1002/ejlt.201400658
Ko, K., Lee, W. K., Oh, C. Y., Lee, S. H., Cho, S. T., Bang, W. J., et al. (2018). Is a combination of antibiotics and non-steroidal anti-inflammatory drugs more beneficial than antibiotic monotherapy for the treatment of female acute uncomplicated cystitis? A randomised controlled pilot study. Urol. J. 15, 365–369. doi:10.22037/uj.v0i0.3716
Kolinsky, D. C., and Liang, S. Y. (2018). Musculoskeletal infections in the emergency department. Emerg. Med. Clin. North Am. 36, 751–766. doi:10.1016/j.emc.2018.06.006
Kowalski, M. L., and Stevenson, D. D. (2013). Classification of reactions to non-steroidal anti-inflammatory drugs. Allergy Clin. North Am. 33, 135–145. doi:10.1016/j.iac.2012.10.008
Krasselt, M., Baerwald, C., Petros, S., and Seifert, O. (2021). Mortality of sepsis in patients with rheumatoid arthritis: a single-center retrospective analysis and comparison with a control group. J. Intensive Care Med. 36, 766–774. doi:10.1177/0885066620917588
Kroesen, V. M., Groschel, M. I., Martinson, N., Zumla, A., Maeurer, M., van der Werf, T. S., et al. (2017). Non-steroidal anti-inflammatory drugs as host-directed therapy for tuberculosis: a systematic review. Front. Immunol. 8, 772. doi:10.3389/fimmu.2017.00772
Kroesen, V. M., Rodriguez-Martinez, P., Garcia, E., Rosales, Y., Diaz, J., Martin-Cespedes, M., et al. (2018). A beneficial effect of low-dose aspirin in a murine model of active tuberculosis. Front. Immunol. 9, 798. doi:10.3389/fimmu.2018.00798
Kronenberg, A., Butikofer, L., Odutayo, A., Muhlemann, K., da Costa, B. R., Battaglia, M., et al. (2017). Symptomatic treatment of uncomplicated lower urinary tract infections in the ambulatory setting: randomised, double-blind trial. BMJ 359, j4784. doi:10.1136/bmj.j4784
Kucukguzel, S. G., and Senkardes, S. (2015). Recent advances in bioactive pyrazoles. Eur. J. Med. Chem. 97, 786–815. doi:10.1016/j.ejmech.2014.11.059
Kudva, A., Kamath, A. T., Dhara, V., and Ravindranath, V. (2019). Chronic recurrent osteomyelitis: a surgeon's enigma. J. Oral Pathol. Med. 48, 180–184. doi:10.1111/jop.12814
Lapi, F., Azoulay, L., Yin, H., Nessim, S. J., and Suissa, S. (2013). Concurrent use of diuretics, angiotensin converting enzyme inhibitors, and angiotensin receptor blockers with non-steroidal anti-inflammatory drugs and risk of acute kidney injury: nested case-control study. BMJ 346, e8525. doi:10.1136/bmj.e8525
Laudy, A. E. (2018). Non-antibiotics, efflux pumps and drug resistance of Gram-negative rods. Pol. J. Microbiol. 67, 129–135. doi:10.21307/pjm-2018-017
Lawn, S. D., and Zumla, A. I. (2011). Tuberculosis. Lancet 378, 57–72. doi:10.1016/S0140-6736(10)62173-3
Le Berre, R., Faure, K., Nguyen, S., Pierre, M., Ader, F., and Guery, B. (2006). Quorum sensing: une nouvelle cible thérapeutique pour Pseudomonas aeruginosa [quorum sensing: a new clinical target for Pseudomonas aeruginosa?]. Med. Mal. Infect. 36, 349–357. doi:10.1016/j.medmal.2006.01.008
Levison, M. E., and Kaye, D. (2013). Treatment of complicated urinary tract infections with an emphasis on drug-resistant gram-negative uropathogens. Curr. Infect. Dis. Rep. 15, 109–115. doi:10.1007/s11908-013-0315-7
Lew, D. P., and Waldvogel, F. A. (2004). Osteomyelitis. Lancet 364, 369–379. doi:10.1016/S0140-6736(04)16727-5
Li, J. T., Li, Z. S., Zhan, X. B., Cui, Z. M., and Nie, S. N. (2003). Experimental study of the safety of the selective COX-2 inhibitor, celecoxib, for gastric mucosa. Chin. J. Dig. Dis. 4, 53–56. doi:10.1046/j.1443-9573.2003.t01-1-00115.x
Liao, X., Yang, F., Li, H., So, P. K., Yao, Z., Xia, W., et al. (2017). Targeting the thioredoxin reductase-thioredoxin system from Staphylococcus aureus by silver ions. Inorg. Chem. 56, 14823–14830. doi:10.1021/acs.inorgchem.7b01904
Lichtenberger, P., and Hooton, T. M. (2008). Complicated urinary tract infections. Curr. Infect. Dis. Rep. 10, 499–504. doi:10.1007/s11908-008-0081-0
Lima, A. L., Oliveira, P. R., Carvalho, V. C., Cimerman, S., and Savio, E. (2014). Recommendations for the treatment of osteomyelitis. Braz J. Infect. Dis. 18, 526–534. doi:10.1016/j.bjid.2013.12.005
Liu, Q. Y., Han, F., Pan, L. P., Jia, H. Y., Li, Q., and Zhang, Z. D. (2018). Inflammation responses in patients with pulmonary tuberculosis in an intensive care unit. Exp. Ther. Med. 15, 2719–2726. doi:10.3892/etm.2018.5775
Mahalmani, V., Prakash, A., and Medhi, B. (2023). Do alternatives to animal experimentation replace preclinical research? Indian J. Pharmacol. 55, 71–75. doi:10.4103/ijp.ijp_223_23
Mahmoud, E. S., Baharoon, S. A., Alsafi, E., and Al-Jahdaly, H. (2016). Acute respiratory distress syndrome complicating community-acquired pneumonia secondary to Mycobacterium tuberculosis in a tertiary care centre in Saudi Arabia. Saudi Med. J. 37, 973–978. doi:10.15537/smj.2016.9.15183
March-Vila, E., Pinzi, L., Sturm, N., Tinivella, A., Engkvist, O., Chen, H., et al. (2017). On the integration of in silico drug design methods for drug repurposing. Front. Pharmacol. 8, 298. doi:10.3389/fphar.2017.00298
Martic-Kehl, M. I., Schibli, R., and Schubiger, P. A. (2012). Can animal data predict human outcome? problems and pitfalls of translational animal research. Eur. J. Nucl. Med. Mol. Imaging 39, 1492–1496. doi:10.1007/s00259-012-2175-z
Melnyk, A. I., Meckes, N., Zyczynski, H. M., Grosse, P. J., Guirguis, M., and Bradley, M. S. (2024). Antibiotic utilization and symptom improvement in a retrospective cohort of women with urinary tract infection symptoms. Int. Urogynecol J. 35, 355–361. doi:10.1007/s00192-023-05676-1
Mendelsohn, A. (2022). New dual-action glaucoma medication promising for glaucoma patients. Available online at: https://glaucoma.org/articles/new-dual-action-glaucoma-medication-promising-for-glaucoma-patients (Accessed February 26, 2025).
Millecam, J., van Bergen, T., Schauvliege, S., Antonissen, G., Martens, A., Chiers, K., et al. (2019). Developmental pharmacokinetics and safety of ibuprofen and its enantiomers in the conventional pig as potential pediatric animal model. Front. Pharmacol. 10, 505. doi:10.3389/fphar.2019.00505
Mingh, J. (2007). xPharm: the comprehensive pharmacology reference. Elsevier, 1–6. doi:10.1016/B978-008055232-3.61268-1
Mohammad, H., Abutaleb, N. S., Dieterly, A. M., Lyle, L. T., and Seleem, M. N. (2021). Investigating auranofin for the treatment of infected diabetic pressure ulcers in mice and dermal toxicity in pigs. Sci. Rep. 11, 10935. doi:10.1038/s41598-021-90360-x
National Institute for Health and Care Excellence (2018). Urinary tract infection (lower): antimicrobial prescribing. Available online at: https://www.nice.org.uk/guidance/ng109/resources/urinary-tract-infection-lower-antimicrobial-prescribing-pdf-66141546350533 (Accessed October 23, 2024).
National Institute for Health and Care Excellence (2022). Resource impact report: romosozumab for treating severe osteoporosis (TA791). Available online at: https://www.nice.org.uk/guidance/ta791/resources/resource-impact-report-pdf-11075818141 (Accessed February 18, 2025).
Nguon, S., Novy, P., and Kokoska, L. (2013). Potentiation of the in vitro anti-staphylococcal effect of oxacillin and tetracycline by the anti-inflammatory drug diacetyl rhein. Chemotherapy 59, 447–452. doi:10.1159/000363730
Nielubowicz, G. R., and Mobley, H. L. (2010). Host-pathogen interactions in urinary tract infection. Nat. Rev. Urol. 7, 430–441. doi:10.1038/nrurol.2010.101
Nugrahani, I., Herawati, D., and Wibowo, M. S. (2023). The benefits and challenges of antibiotics–non-steroidal anti-inflammatory drugs non-covalent reaction. Molecules 28, 3672. doi:10.3390/molecules28093672
Ohshita, Y., Hiramatsu, K., and Yokota, T. (1990). A point mutation in norA gene is responsible for quinolone resistance in Staphylococcus aureus. Biochem. Biophys. Res. Commun. 172, 1028–1034. doi:10.1016/0006-291x(90)91549-8
Okpala, O. E., Rondevaldova, J., Osei-Owusu, H., Kudera, T., Kokoskova, and T., and Kokoska, L. (2024). Susceptibility of Staphylococcus aureus to anti-inflammatory drugs with a focus on the combinatory effect of celecoxib with oxacillin in vitro. Molecules 29, 3665. doi:10.3390/molecules29153665
Oliveira, I. M., Borges, A., Borges, F., and Simoes, M. (2019). Repurposing ibuprofen to control Staphylococcus aureus biofilms. Eur. J. Med. Chem. 166, 197–205. doi:10.1016/j.ejmech.2019.01.046
Ornelas, A., Zacharias-Millward, N., Menter, D. G., Davis, J. S., Lichtenberger, L., Hawke, D., et al. (2017). Beyond COX-1: the effects of aspirin on platelet biology and potential mechanisms of chemoprevention. Cancer Metastasis Rev. 36, 289–303. doi:10.1007/s10555-017-9675-z
Ozturk, I., Eraç, Y., Ballar Kırmızibayrak, P., and Ermertcan, S. (2021). Non-steroidal anti-inflammatory drugs alter antibiotic susceptibility and expression of virulence-related genes and protein A of Staphylococcus aureus. Turk J. Med. Sci. 51, 835–847. doi:10.3906/sag-2003-60
Page, A. T., Clifford, R. M., Potter, K., Schwartz, D., and Etherton-Beer, C. D. (2016). The feasibility and effect of deprescribing in older adults on mortality and health: a systematic review and meta-analysis. Br. J. Clin. Pharmacol. 82, 583–623. doi:10.1111/bcp.12975
Pallio, G., Bitto, A., Pizzino, G., Galfo, F., Irrera, N., Minutoli, L., et al. (2016). Use of a balanced dual cyclooxygenase-1/2 and 5-lypoxygenase inhibitor in experimental colitis. Eur. J. Pharmacol. 789, 152–162. doi:10.1016/j.ejphar.2016.07.033
Patyar, S., Prakash, A., and Medhi, B. (2011). Dual inhibition: a novel promising pharmacological approach for different disease conditions. J. Pharm. Pharmacol. 63, 459–471. doi:10.1111/j.2042-7158.2010.01236.x
Pavelka, K., Bruyere, O., Cooper, C., Kanis, J. A., Leeb, B. F., Maheu, E., et al. (2016). Diacerein: benefits, risks and place in the management of osteoarthritis. An opinion-based report from the ESCEO. Drugs Aging 33, 75–85. doi:10.1007/s40266-016-0347-4
Pereira, P. A., Trindade, B. C., Secatto, A., Nicolete, R., Peres-Buzalaf, C., Ramos, S. G., et al. (2013). Celecoxib improves host defense through prostaglandin inhibition during Histoplasma capsulatum infection. Mediat. Inflamm. 2013, 950981. doi:10.1155/2013/950981
Pina-Vaz, C., Sansonetty, F., Rodrigues, A. G., Martinez-DE-Oliveira, J., Fonseca, A. F., and Mardh, P. A. (2000). Antifungal activity of ibuprofen alone and in combination with fluconazole against Candida species. J. Med. Microbiol. 49, 831–840. doi:10.1099/0022-1317-49-9-831
Pinzi, L., Bisi, N., and Rastelli, G. (2024). How drug repurposing can advance drug discovery: challenges and opportunities. Front. Drug Discov. 4, 1460100. doi:10.3389/fddsv.2024.1460100
Powell, L. C., Pritchard, M. F., Ferguson, E. L., Powell, K. A., Patel, S. U., Rye, P. D., et al. (2018). Targeted disruption of the extracellular polymeric network of Pseudomonas aeruginosa biofilms by alginate oligosaccharides. NPJ Biofilms Microbiomes 4, 13. doi:10.1038/s41522-018-0056-3
Quadros Barse, L., Ulfig, A., Varatnitskaya, M., Vázquez-Hernández, M., Yoo, J., Imann, A. M., et al. (2024). Comparison of the mechanism of antimicrobial action of the gold(I) compound auranofin in Gram-positive and Gram-negative bacteria. Microbiol. Spectr. 12, e0013824. doi:10.1128/spectrum.00138-24
Ratia, C., Soengas, R. G., and Soto, S. M. (2022). Gold-derived molecules as new antimicrobial agents. Front. Microbiol. 13, 846959. doi:10.3389/fmicb.2022.846959
Rayaprolu, B. M., Strawser, J. J., and Anyarambhatla, G. (2018). Excipients in parenteral formulations: selection considerations and effective utilization with small molecules and biologics. Drug Dev. Ind. Pharm. 44, 1565–1571. doi:10.1080/03639045.2018.1483392
Reeve, E., Gnjidic, D., Long, J., and Hilmer, S. (2015). A systematic review of the emerging definition of 'deprescribing' with network analysis: implications for future research and clinical practice. Br. J. Clin. Pharmacol. 80, 1254–1268. doi:10.1111/bcp.12732
Richeldi, L., Ewer, K., Losi, M., Hansell, D. M., Roversi, P., Fabbri, L. M., et al. (2004). Early diagnosis of subclinical multidrug-resistant tuberculosis. Ann. Intern Med. 140, 709–713. doi:10.7326/0003-4819-140-9-200405040-00010
Sachdeva, A., Nambiar, A., Rai, B. P., Veeratterapillay, R., and Harding, C. (2021). Non-steroidal anti-inflammatory drugs for treating symptomatic uncomplicated urinary tract infections in non-pregnant adult women. Cochrane Database Syst. Rev. 2021, CD014762. doi:10.1002/14651858.CD014762
Sasindran, S. J., and Torrelles, J. B. (2011). Mycobacterium tuberculosis infection and inflammation: what is beneficial for the host and for the bacterium? Front. Microbiol. 2, 2. doi:10.3389/fmicb.2011.00002
Scottish Intercollegiate Guidelines Network (2020). Management of suspected bacterial lower urinary tract infection in adult women. Edinburgh: SIGN. Available online at: https://www.sign.ac.uk/media/1766/sign-160-uti-0-1_web-version.pdf (Accessed October 23, 2024).
Sedlacek, M., Gemery, J. M., Cheung, A. L., Bayer, A. S., and Remillard, B. D. (2007). Aspirin treatment is associated with a significantly decreased risk of Staphylococcus aureus bacteremia in hemodialysis patients with tunneled catheters. Am. J. Kidney Dis. 49, 401–408. doi:10.1053/j.ajkd.2006.12.014
Seong, Y. J., Alhashimi, M., Mayhoub, A., Mohammad, H., and Seleem, M. N. (2020). Repurposing fenamic acid drugs to combat multidrug-resistant Neisseria gonorrhoeae. Antimicrob Agents Chemother. Antimicrob. Agents Chemother. 64, e02206. doi:10.1128/AAC.02206-19
Shah, P. N., Marshall-Batty, K. R., Smolen, J. A., Tagaev, J. A., Chen, Q., Rodesney, C. A., et al. (2018). Antimicrobial activity of ibuprofen against cystic fibrosis-associated gram-negative pathogens. Antimicrob. Agents Chemother. 62, e01574. doi:10.1128/AAC.01574-17
Shirin, H., Moss, S. F., Kancherla, S., Kancherla, K., Holt, P. R., Weinstein, I. B., et al. (2006). Non-steroidal anti-inflammatory drugs have bacteriostatic and bactericidal activity against Helicobacter pylori. J. Gastroenterol. Hepatol. 21, 1388–1393. doi:10.1111/j.1440-1746.2006.04194.x
Silverstein, F. E., Faich, G., Goldstein, J. L., Simon, L. S., Pincus, T., Whelton, A., et al. (2000). Gastrointestinal toxicity with celecoxib vs nonsteroidal anti-inflammatory drugs for osteoarthritis and rheumatoid arthritis: the CLASS study: a randomized controlled trial. Celecoxib Long-term Arthritis Safety Study. JAMA 284, 1247–1255. doi:10.1001/jama.284.10.1247
Singh, J. A., and Yu, S. (2018). Septic arthritis in emergency departments in the us: a national study of health care utilization and time trends. Arthritis Care Res. Hob. 70, 320–326. doi:10.1002/acr.23270
Slomovitz, B. M., Broaddus, R. R., Schmandt, R., Wu, W., Oh, J. C., Ramondetta, L. M., et al. (2004). Expression of imatinib mesylate-targeted kinases in endometrial carcinoma. Gynecol. Oncol. 95, 32–36. doi:10.1016/j.ygyno.2004.06.052
Smith, M., Roberts, M., and Al-Kassas, R. (2022). Implantable drug delivery systems for the treatment of osteomyelitis. Drug Dev. Ind. Pharm. 48, 511–527. doi:10.1080/03639045.2022.2135729
Song, J. H., Ko, K. S., Suh, J. Y., Oh, W. S., Kang, C. I., Chung, D. R., et al. (2008). Clinical implications of vancomycin-resistant Enterococcus faecium (VRE) with VanD phenotype and vanA genotype. J. Antimicrob. Chemother. 61, 838–844. doi:10.1093/jac/dkn025
Song, Y., Baer, M., Srinivasan, R., Lima, J., Yarranton, G., Bebbington, C., et al. (2012). PcrV antibody-antibiotic combination improves survival in Pseudomonas aeruginosa-infected mice. Eur. J. Clin. Microbiol. Infect. Dis. 31, 1837–1845. doi:10.1007/s10096-011-1509-2
Sood, S., Yadav, A., and Shrivastava, R. (2016). Mycobacterium aurum is unable to survive Mycobacterium tuberculosis latency associated stress conditions: implications as non-suitable model organism. Indian J. Microbiol. 56, 198–204. doi:10.1007/s12088-016-0564-x
Sozer, S., Diniz, G., and Lermioglu, F. (2011). Effects of celecoxib in young rats: histopathological changes in tissues and alterations of oxidative stress/antioxidant defense system. Arch. Pharm. Res. 34, 253–259. doi:10.1007/s12272-011-0211-3
Spitaleri, A., Ghodousi, A., Miotto, P., and Cirillo, D. M. (2019). Whole genome sequencing in Mycobacterium tuberculosis. ann transl med. Ann. Transl. Med. 7, S197. doi:10.21037/atm.2019.07.28
Stahl, S. M., Pradko, J. F., Haight, B. R., Modell, J. G., Rockett, C. B., and Learned-Coughlin, S. (2004). A review of the neuropharmacology of bupropion, a dual norepinephrine and dopamine reuptake inhibitor. Prim. Care Companion J. Clin. Psychiatry 6, 159–166. doi:10.4088/pcc.v06n0403
Stanbury, R. M., and Graham, E. M. (1998). Systemic corticosteroid therapy-side effects and their management. Br. J. Ophthalmol. 82, 704–708. doi:10.1136/bjo.82.6.704
Steinman, M. A., Landefeld, C. S., Rosenthal, G. E., Berthenthal, D., Sen, S., and Kaboli, P. J. (2006). Polypharmacy and prescribing quality in older people. J. Am. Geriatr. Soc. 54, 1516–1523. doi:10.1111/j.1532-5415.2006.00889.x
Svensson, E. M., Murray, S., Karlsson, M. O., and Dooley, K. E. (2015). Rifampicin and rifapentine significantly reduce concentrations of bedaquiline, a new anti-TB drug. J. Antimicrob. Chemother. 70, 1106–1114. doi:10.1093/jac/dku504
Tabacco, G., and Bilezikian, J. P. (2019). Osteoanabolic and dual-action drugs. Br. J. Clin. Pharmacol. 85, 1084–1094. doi:10.1111/bcp.13766
Tabatabaeifar, F., Isaei, E., Kalantar-Neyestanaki, D., and Morones-Ramírez, J. R. (2022). Antimicrobial and antibiofilm effects of combinatorial treatment formulations of anti-inflammatory drugs-common antibiotics against pathogenic bacteria. Pharmaceutics 15, 4. doi:10.3390/pharmaceutics15010004
Tahan, T. T., Gabardo, B. M. A., and Rossoni, A. M. O. (2020). Tuberculosis in childhood and adolescence: a view from different perspectives. J. Pediatr. Rio J. 96 (1), 99–110. doi:10.1016/j.jped.2019.11.002
Takahashi, O., and Hiraga, K. (1985). Differences in the haemorrhagic toxicity of aspirin between rats and mice. Acta Pharmacol. Toxicol. (Copenh) 56, 6–13. doi:10.1111/j.1600-0773.1985.tb01246.x
Tarkowski, A. (2006). Infection and musculoskeletal conditions: infectious arthritis. Best. Pract. Res. Clin. Rheumatol. 20, 1029–1044. doi:10.1016/j.berh.2006.08.001
Taylor, R. S., Fotopoulos, G., and Maibach, H. (2011). Safety profile of topical diclofenac: a meta-analysis of blinded, randomized, controlled trials in musculoskeletal conditions. Curr. Med. Res. Opin. 27, 605–622. doi:10.1185/03007995.2010.550606
Thangamani, S., Maland, M., Mohammad, H., Pascuzzi, P. E., Avramova, L., Koehler, C. M., et al. (2017). Repurposing approach identifies auranofin with broad spectrum antifungal activity that targets Mia40-Erv1 pathway. Front. Cell Infect. Microbiol. 7, 4. doi:10.3389/fcimb.2017.00004
Thangamani, S., Mohammad, H., Abushahba, M. F., Sobreira, T. J., Hedrick, V. E., Paul, L. N., et al. (2016a). Antibacterial activity and mechanism of action of auranofin against multi-drug-resistant bacterial pathogens. Sci. Rep. 6, 22571. doi:10.1038/srep22571
Thangamani, S., Mohammad, H., Abushahba, M. F., Sobreira, T. J., and Seleem, M. N. (2016b). Repurposing auranofin for the treatment of cutaneous staphylococcal infections. Int. J. Antimicrob. Agents 47, 195–201. doi:10.1016/j.ijantimicag.2015.12.016
Thangamani, S., Younis, W., and Seleem, M. N. (2015). Repurposing celecoxib as a topical antimicrobial agent. Front. Microbiol. 6, 750. doi:10.3389/fmicb.2015.00750
Tong, S. Y. C., Davis, J. S., Eichenberger, E., Holland, T. L., and Fowler, V. G. (2015). Staphylococcus aureus infections: epidemiology, pathophysiology, clinical manifestations, and management. Clin. Microbiol. Rev. 28, 603–661. doi:10.1128/CMR.00134-14
Urish, K. L., and Cassat, J. E. (2020). Staphylococcus aureus osteomyelitis: bone, bugs, and surgery. Infect. Immun. 88, 009322-19. doi:10.1128/IAI.00932-19
Van Norman, G. A. (2019). Limitations of animal studies for predicting toxicity in clinical trials: is it time to rethink our current approach? JACC Basic Transl. Sci. 4, 845–854. doi:10.1016/j.jacbts.2019.10.008
Vargason, A. M., Anselmo, A. C., and Mitragotri, S. (2021). The evolution of commercial drug delivery technologies. Nat. Biomed. Eng. 5, 951–967. doi:10.1038/s41551-021-00698-w
Verma, T., Bhaskarla, C., Sadhir, I., Sreedharan, S., and Nandi, D. (2018). Non-steroidal anti-inflammatory drugs, acetaminophen and ibuprofen, induce phenotypic antibiotic resistance in Escherichia coli: roles of marA and acrB. FEMS Microbiol. Lett. 365, doi:10.1093/femsle/fny251
Vik, I., Bollestad, M., Grude, N., Bærheim, A., Damsgaard, E., Neumark, T., et al. (2018). Ibuprofen versus pivmecillinam for uncomplicated urinary tract infection in women-A double-blind, randomized non-inferiority trial. PLoS Med. 15, e1002569. doi:10.1371/journal.pmed.1002569
Vilaplana, C., Marzo, E., Tapia, G., Diaz, J., Garcia, V., and Cardona, P. J. . (2013). Ibuprofen therapy resulted in significantly decreased tissue bacillary loads and increased survival in a new murine experimental model of active tuberculosis. J. Infect. Dis. 208, 199–202. doi:10.1093/infdis/jit152
Walter, N., Baertl, S., Alt, V., and Rupp, M. (2021). What is the burden of osteomyelitis in Germany? An analysis of inpatient data from 2008 through 2018. BMC Infect. Dis. 21, 550. doi:10.1186/s12879-021-06274-6
Whittle, B. J. (2000). COX-1 and COX-2 products in the gut: therapeutic impact of COX-2 inhibitors. Gut 47, 320–325. doi:10.1136/gut.47.3.320
Wu, H., Kouladjian O'Donnell, L., Fujita, K., Masnoon, N., and Hilmer, S. N. (2021). Deprescribing in the older patient: a narrative review of challenges and solutions. Int. J. Gen. Med. 14, 3793–3807. doi:10.2147/IJGM.S253177
Yao, C., and Narumiya, S. (2019). Prostaglandin-cytokine crosstalk in chronic inflammation. Br. J. Pharmacol. 176, 337–354. doi:10.1111/bph.14530
Yin, Z., Wang, Y., Whittell, L. R., Jergic, S., Liu, M., Harry, E., et al. (2014). DNA replication is the target for the antibacterial effects of nonsteroidal anti-inflammatory drugs. Chem. Biol. 21, 481–487. doi:10.1016/j.chembiol.2014.02.009
Zhang, H., Liu, S., Yue, J., Sun, S., Lv, Q., Jian, S., et al. (2019). In vitro, antimicrobial activity of diacerein on 76 isolates of gram-positive cocci from bacterial keratitis patients and in vivo study of diacerein eye drops on Staphylococcus aureus keratitis in mice. Antimicrob. Agents Chemother. 63, 018744-18–e1918. doi:10.1128/AAC.01874-18
Zhang, L., Lu, Y. M., and Dong, X. Y. (2004). Effects and mechanism of the selective COX-2 inhibitor, celecoxib, on rat colitis induced by trinitrobenzene sulfonic acid. Chin. J. Dig. Dis. 5, 110–114. doi:10.1111/j.1443-9573.2004.00164.x
Zhang, S., Qu, X., Tang, H., Wang, Y., Yang, H., Yuan, W., et al. (2021). Diclofenac resensitises methicillin-resistant Staphylococcus aureus to β-Lactams and prevents implant infections. Adv. Sci. (Weinh) 8, 2100681. doi:10.1002/advs.202100681
Keywords: bacterial infection, cellulitis, cystitis, dual-action drugs, inflammation, osteomyelitis, septic arthritis, single-drug therapy
Citation: Okpala OE, Rondevaldova J and Kokoska L (2025) Anti-inflammatory drugs as potential antimicrobial agents: a review. Front. Pharmacol. 16:1557333. doi: 10.3389/fphar.2025.1557333
Received: 08 January 2025; Accepted: 13 March 2025;
Published: 08 April 2025.
Edited by:
Francesca Oliviero, University of Padua, ItalyReviewed by:
Chee Loong Teo, Agri Season Sdn Bhd, MalaysiaMaria Lucila Hernández Macedo, Tiradentes University, Brazil
Copyright © 2025 Okpala, Rondevaldova and Kokoska. This is an open-access article distributed under the terms of the Creative Commons Attribution License (CC BY). The use, distribution or reproduction in other forums is permitted, provided the original author(s) and the copyright owner(s) are credited and that the original publication in this journal is cited, in accordance with accepted academic practice. No use, distribution or reproduction is permitted which does not comply with these terms.
*Correspondence: Ladislav Kokoska, a29rb3NrYUBmdHouY3p1LmN6