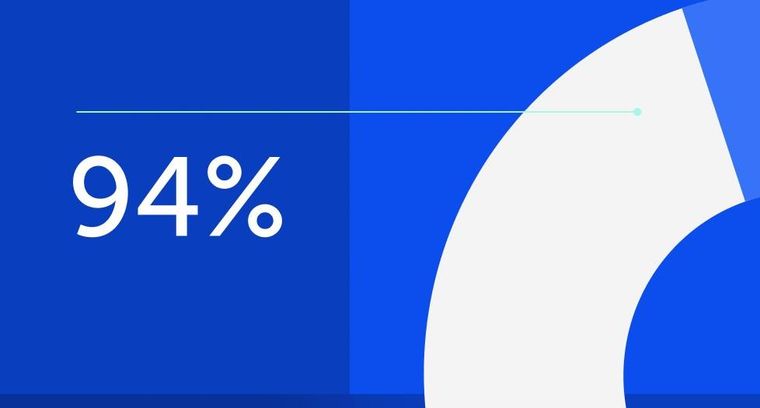
94% of researchers rate our articles as excellent or good
Learn more about the work of our research integrity team to safeguard the quality of each article we publish.
Find out more
REVIEW article
Front. Pharmacol., 04 March 2025
Sec. Drug Metabolism and Transport
Volume 16 - 2025 | https://doi.org/10.3389/fphar.2025.1557298
This article is part of the Research TopicInnovative Applications of Microalgae: Advancements in Health Care, Antioxidants, Synthetic Biology, and Functional FoodsView all articles
Microalgae are emerging as a key player in healthcare, functional foods, and sustainable biotech due to their capacity to produce bioactive compounds like β-glucans, omega-3 fatty acids, and antioxidants in an eco-friendly manner. This review comprehensively discusses the role of microalgae in healthcare and functional foods, focusing particularly on β-glucan therapeutics, drug delivery innovations, and synthetic biology applications. In healthcare, microalgae-derived compounds show immense promise for treating diseases, boosting immunity, and tackling oxidative stress. Euglena-derived paramylon, a type of β-glucan, has shown potential in various medical applications, including immunomodulation and anticancer therapy. Synthetic biology and bioprocess engineering are enhancing microalgae’s therapeutic and nutritional value, with applications in drug delivery and personalized medicine. To maximize the potential of microalgae, further research and development are needed to address scalability, regulatory alignment, and consumer acceptance, with a focus on interdisciplinary collaboration and sustainable practices to align healthcare innovation with environmental conservation.
Microalgae represent an extraordinary resource in the realm of biotechnology, offering diverse applications in health, such as functional foods and nutraceuticals; nutrition, including protein-rich feedstocks; and environmental sustainability through carbon capture and wastewater treatment. Their unique capacity for photosynthesis and production of high-value bioactive compounds, including β-glucans, pigments, and pharmaceuticals, underpins their increasing importance in modern science and industry (Nethravathy et al., 2019; Ampofo and Abbey, 2022). The therapeutic effects of microalgae bioactive compounds and their applications in biomedical research have garnered growing interest (Huang et al., 2024). Studies of bioactive compounds from microalgae were provided in Table 1.
Among these, β-glucans—natural polysaccharides known for their immunomodulatory and antioxidant properties—have garnered significant attention for their potential in functional foods, nutraceuticals, and pharmaceuticals, such as their incorporation in paramylon-enriched supplements for immune enhancement and gut health (Murphy et al., 2020; Taylor et al., 2020). Euglena-derived paramylon, a β-(1→3)-glucan, is particularly notable for its high purity, ease of extraction, and versatile applications (Gissibl et al., 2019).
Another emerging frontier is the utilization of microalgae in drug delivery systems. Microalgal cells and their derivatives, such as lipids and biopolymers, have shown promise as carriers for therapeutic agents, leveraging their biocompatibility, bioavailability, and ability to target specific tissues (Vieira et al., 2020; Huang et al., 2024). These attributes, coupled with advancements in synthetic biology, position microalgae as pivotal players in addressing global challenges in health, energy, and environmental sustainability (Fernández et al., 2021; Naduthodi et al., 2021). Microalgae in healthcare and functional food applications were summarized in Figure 1.
Synthetic biology further expands the potential of microalgae by enabling precise genetic modifications to enhance productivity and introduce novel functionalities (Naduthodi et al., 2021). For example, CRISPR-Cas9 has been used to boost lipid biosynthesis in Nannochloropsis oceanica, while TALENs have optimized pigment production pathways in Chlamydomonas reinhardtii (Liu et al., 2024; Shin et al., 2024). These modifications have resulted in higher yields and more efficient bioproduct generation. Advances in genome editing tools, such as CRISPR-Cas9 and TALENs, have catalyzed the engineering of microalgae for sustainable production of biofuels, pigments, and therapeutic proteins (Fayyaz et al., 2020; Naduthodi et al., 2021).
This review provides a comprehensive analysis of the advancements in β-glucan research, drug delivery systems and synthetic biology applications, involving microalgae. These three areas were selected because they exemplify distinct yet interconnected facets of microalgae’s potential in biotechnology. β-Glucan research highlights the foundational bioactive properties of microalgae, synthetic biology showcases the tools and strategies to enhance these properties, and drug delivery systems demonstrate their practical applications in medicine. Together, they form a cohesive narrative that bridges natural bioactivity, engineered innovation, and real-world therapeutic impact, offering a holistic view of microalgae’s promise in health and sustainability.
β-Glucans are natural polysaccharides composed of glucose monomers linked predominantly by β-(1→3), β-(1→4) and occasionally β-(1→6) glycosidic bonds. These compounds are widely distributed in nature, being found in cereals, fungi, yeast, and microalgae (Chiozzi et al., 2021; van Steenwijk et al., 2021; Lazaridou et al., 2007; Kumar et al., 2025). They have attracted significant scientific and commercial interest due to their broad range of biological activities, including immunomodulation, antioxidant effects, and potential applications in functional foods and pharmaceuticals. Among various sources, microalgae-derived β-glucans are particularly noteworthy for their unique structural properties, cost-effective production, and scalability (Murphy et al., 2023; Kumar et al., 2025). Studies of β-Glucan from microalgae were provided in Table 2.
Euglena gracilis is a unique microalga that produces a highly linear form of β-(1→3)-glucan called paramylon. This distinguishes it from other sources of β-glucans due to several specific advantages. First, Euglena biomass contains an exceptionally high proportion of paramylon, ranging from 70% to 80% of its dry weight, which is substantially higher than β-glucan yields from yeast (30%–50%) or cereals (5%–8%) (Wu et al., 2021; Lazaridou et al., 2007; Chiozzi et al., 2021). The high content of paramylon significantly enhances its economic viability for industrial applications.
Unlike other sources, where β-glucans are embedded in the cell wall and require enzymatic or chemical disruption for extraction, paramylon is stored as intracellular granules. This simplifies its extraction process, reducing processing costs and preserving the molecule’s structural integrity (Guo et al., 2020; Chiba et al., 2024). Additionally, paramylon’s linear β-(1→3) structure is believed to enhance its bioactivity, making it highly effective in modulating immune responses and exhibiting antioxidant properties (Barsanti and Gualtieri, 2019; Gao et al., 2022). Euglena β-glucan (paramylon) studies were provided in Table 3.
Euglena’s paramylon outperforms other β-glucan sources in several critical aspects, including yield, ease of extraction, and bioactivity. For instance, cereal-derived β-glucans, such as those from oats and barley, are primarily branched (β-(1→3, 1→4)) and require extensive processing that can degrade their structure and reduce bioactivity (Henrion et al., 2019; Lante et al., 2023). Similarly, yeast-derived β-glucans often contain allergenic impurities and are less effective as immunomodulators compared to the linear β-(1→3) structure of paramylon (Yamamoto et al., 2020; Kumar et al., 2025).
In terms of industrial applications, Euglena-derived β-glucan excels in functional food formulations due to its high insolubility and stability under various conditions. Its high purity and bioactivity also make it a promising candidate for pharmaceutical applications, such as immunotherapies and antioxidant treatments (Nakashima et al., 2017; Yamamoto et al., 2018; Yasuda et al., 2020; Huang et al., 2023).
Paramylon demonstrates a wide range of biological activities, supported by both in vitro and in vivo studies. It has been shown to enhance immune responses by activating macrophages, dendritic cells, and natural killer cells. For example, studies in mice and human cell cultures have reported increased cytokine production, including TNF-α and IL-6, following paramylon administration (Nakashima et al., 2017; Russo et al., 2017). Immune activation of murine RAW264.7 macrophages by sonicated and alkalized paramylon from Euglena was also reported (Guo et al., 2020). Additionally, paramylon’s prebiotic effects have been demonstrated in probiotics (Dai et al., 2022) and animal models, where it modulates gut microbiota and improves gut health (Taylor et al., 2020).
Paramylon also exhibits potent antioxidant properties, effectively scavenging reactive oxygen species (ROS) and reducing oxidative damage in various tissues. Euglena paramylon, and its aqueous extract constructed with chitosan-hyaluronic acid hydrogel facilitate cutaneous wound healing in mice without inducing excessive inflammatory response (Li et al., 2021). Animal studies have highlighted its role in protecting liver and kidney tissues from oxidative stress, as well as enhancing wound healing through angiogenesis and collagen deposition (Yasuda et al., 2018; Xie et al., 2021).
In functional foods and nutraceuticals, paramylon has been successfully incorporated into products aimed at weight management and cholesterol reduction. Its unique structural properties and bioactivity make it an ideal ingredient for developing next-generation functional foods. Moreover, its safety and efficacy in enhancing immune responses have opened avenues for its use in animal feed, particularly in aquaculture and livestock. Supplementation with paramylon in fish diets has been shown to improve growth performance and reduce susceptibility to infections (Bashir and Choi, 2017; Yamamoto et al., 2018; Hayatgheib et al., 2020).
Despite its promising potential, several challenges remain in the large-scale cultivation of Euglena and the optimization of paramylon extraction. Cost-effective and sustainable cultivation systems, such as advanced photobioreactors and nutrient recycling strategies, are essential to increase production efficiency (Fujita et al., 2008; Vogler et al., 2018). Additionally, exploring chemical modifications of paramylon to enhance its solubility and bioavailability could further expand its applications (Feuzing et al., 2022; Li et al., 2024).
Future research should prioritize large-scale clinical trials to validate the health benefits observed in preclinical studies. Such trials would provide robust evidence for its efficacy and broaden its acceptance in global markets (Ullmann and Grimm, 2021; Wang Q. et al., 2024). Moreover, interdisciplinary collaborations combining synthetic biology, metabolic engineering, and material sciences could unlock novel applications for paramylon, from drug delivery systems to bioactive packaging materials (Monfils et al., 2011; He L. et al., 2024).
Euglena-derived β-glucan, or paramylon, is a remarkable biomolecule with significant potential across diverse industries. Its high yield, ease of extraction, and versatile bioactivities make it a superior alternative to traditional β-glucan sources. By addressing current challenges and exploring innovative applications, paramylon can play a pivotal role in advancing functional foods, pharmaceuticals, and sustainable animal health solutions.
Microalgae are emerging as innovative platforms in drug delivery systems due to their unique biological properties, including biocompatibility, natural abundance, and the ability to be genetically modified. Their cellular structures, bioactive compounds, and sustainable cultivation methods make them ideal candidates for developing advanced drug delivery technologies (Geng et al., 2024; Rakhi et al., 2025).
Microalgae offer several key advantages as carriers in drug delivery systems. Firstly, their cell walls are naturally composed of biocompatible polysaccharides such as cellulose and chitin, which can be tailored to encapsulate and release pharmaceutical compounds in a controlled manner (Geng et al., 2024; Rakhi et al., 2025). Additionally, microalgae-derived lipids and proteins can be engineered to form nanoparticles or vesicles, enhancing the bioavailability of hydrophobic drugs (Dubey et al., 2023).
Moreover, microalgae possess intrinsic bioactivities, including antioxidant and anti-inflammatory properties, which can synergize with therapeutic agents. For example, carotenoids like astaxanthin and lutein have been shown to protect drug molecules from oxidative degradation, improving their stability and efficacy (Aslam et al., 2021; Pereira et al., 2024). Furthermore, their natural ability to accumulate bioactive compounds allows for simultaneous production of therapeutic agents and drug carriers, reducing manufacturing complexity (Lu et al., 2024).
One of the most promising applications of microalgae in drug delivery is their role in targeted therapies. Genetically engineered microalgae can express surface ligands that bind specifically to cancer cells or inflamed tissues. For instance, C. reinhardtii has been modified to deliver monoclonal antibodies to tumor sites, demonstrating high specificity and minimal off-target effects (Delalat et al., 2015; Dubey et al., 2023).
Another innovative approach involves the use of microalgae-derived extracellular vesicles (EVs) for drug delivery. These vesicles, secreted naturally by microalgae, can be loaded with small-molecule drugs, nucleic acids, or proteins, and have been shown to cross biological barriers such as the blood-brain barrier (Adamo et al., 2021; Adamo et al., 2024).
Microalgae have also been explored for oral drug delivery due to their resistance to gastrointestinal conditions. Encapsulation of probiotics and bioactive compounds in algal cells such as Spirulina platensis protects these agents from acidic and enzymatic degradation, ensuring their release in the intestinal tract (Zhong et al., 2021; Zhang et al., 2022). Additionally, algal-derived polysaccharides like alginate and carrageenan are widely used as matrices for oral drug formulations due to their excellent mucoadhesive and gel-forming properties (Geng et al., 2024). An oral microsphere strategy (Eug/Lut@HAMA) was developed by encapsulating Euglena (Eug) and luteolin (Lut) within methacrylated hyaluronic acid (HAMA) microspheres as a potential treatment for hyperuricemia with renal injury (Liu et al., 2025).
Microalgae-based drug delivery systems, despite their immense potential, face several challenges that must be overcome for widespread application. Firstly, the standardization of cultivation and processing is crucial due to the variability in algal biomass composition caused by environmental and cultivation conditions, which affects the consistency of drug carrier production. To address this, the development of standardized protocols and closed bioreactor systems can help mitigate these issues (Sharma et al., 2024; Kapoor et al., 2024). Secondly, the efficiency of drug loading and release within algal systems needs optimization, with encapsulation efficiency and controlled release kinetics requiring attention. Advances in bioengineering and nanotechnology could be instrumental in improving these parameters (Patra et al., 2018; Nallasamy and Natarajan, 2021). Thirdly, regulatory hurdles present a significant barrier since microalgae-based carriers are a relatively new concept, and regulatory frameworks for their approval in pharmaceutical applications are underdeveloped. Comprehensive safety assessments and clear guidelines are essential to facilitate market entry (Su et al., 2023). Lastly, the cost-effectiveness of scaling up microalgae cultivation and processing for pharmaceutical-grade applications is a concern, as it can be expensive. However, integrating drug delivery with other algal-based industries, such as biofuels and nutraceuticals, may help offset production costs (Grama et al., 2022).
The integration of synthetic biology with microalgae research holds significant promise for drug delivery. Advances in genome editing tools such as CRISPR-Cas9 enable precise engineering of algal cells to enhance drug loading capacities and targeting efficiencies (Patel et al., 2023; Ambily et al., 2024). Additionally, the development of hybrid systems combining microalgae with synthetic nanocarriers, such as liposomes or dendrimers, could further expand their applications in complex therapeutic regimens (Huang et al., 2024).
Emerging technologies such as microfluidics and AI-driven design are expected to accelerate the development of microalgae-based drug delivery systems. These tools can optimize algal cultivation, drug encapsulation, and release profiles, ensuring scalability and clinical efficacy (Liang et al., 2015; Ummalyma et al., 2020). Furthermore, interdisciplinary collaborations among biologists, engineers, and pharmaceutical scientists will be essential to translate these innovations into commercial products.
Synthetic biology integrates biology and engineering to design, construct, and modify biological systems for practical applications. Microalgae, as versatile photosynthetic organisms, provide a promising platform for synthetic biology due to their ability to produce a diverse range of bioactive compounds, high biomass yields, and potential environmental benefits. With the rapid advancement of genome editing tools and metabolic engineering techniques, synthetic biology in microalgae has entered a transformative phase (Hu et al., 2023; Jeong et al., 2023).
Modern synthetic biology in microalgae is driven by genome-editing tools like CRISPR-Cas9, TALENs, and ZFNs. For example, CRISPR-Cas9 was successfully applied in C. reinhardtii to knock out genes involved in starch synthesis, which significantly increased lipid production (Baek et al., 2016). Using TALENs technique, the gene encoding the urease enzyme in the model diatom, Phaeodactylum tricornutum, was targeted for interruption (Weyman et al., 2015). Similarly, TALENs have been employed in Nannochloropsis to enhance the expression of key genes in the fatty acid biosynthesis pathway, boosting biodiesel precursor yields (Song et al., 2024). These genome-editing tools, combined with universal cloning systems, allow researchers to precisely modify genetic pathways and introduce new functionalities (Patwari et al., 2023). A notable case involved engineering Haematococcus pluvialis to produce higher concentrations of astaxanthin, a valuable antioxidant with applications in nutraceuticals (Gu et al., 2024). High-throughput sequencing revealed low-efficacy genome editing using Cas9 RNPs electroporation and single-celled microinjection provided an alternative to deliver CRISPR reagents into E. gracilis (Chen et al., 2022a) and a synthetic biology perspective on the bioengineering tools for an industrial biotechnology platform were being built up recently (Chen et al., 2022b).
Synthetic biology has enabled the enhancement of metabolic pathways in microalgae to produce valuable compounds. In the case of fatty acids production, the engineered N. oceanica produced more eicosapentaenoic acid (EPA), a high-value ω-3 polyunsaturated fatty acids, than wild type (Liu et al., 2024). Another study applied CRISPR-Cas9 to improve the triacylglycerol pathway in Dunaliella salina, leading to higher biodiesel yields (Hu et al., 2023). Pigments and nutraceuticals have also benefited, with engineered strains producing increased levels of carotenoids and phycocyanin (Cao et al., 2023). For instance, P. tricornutum was engineered to produce fucoxanthin, a carotenoid with anti-cancer and anti-inflammatory properties, demonstrating the scalability of such innovations (Wu S. et al., 2023).
Improving photosynthetic efficiency and carbon fixation pathways has been a major focus of synthetic biology in microalgae. One approach involves reducing antenna sizes in photosystems to minimize energy loss via non-photochemical quenching (NPQ). For example, modifications in Nannochloropsis gaditana led to a 20% increase in photosynthetic efficiency (Negi et al., 2020). Additionally, engineering the Calvin-Benson-Bassham cycle in C. reinhardtii by incorporating synthetic carbon fixation pathways significantly improved carbon assimilation rates (Boisset et al., 2023). Another approach involved incorporating cyanobacterial carbon-concentrating mechanisms into Chlorella vulgaris, enhancing its ability to fix CO2 under high-light conditions (Long et al., 2016).
Biosensors have emerged as powerful tools in synthetic biology, enabling real-time monitoring of cellular and environmental parameters. These genetically encoded sensors can detect specific metabolites or environmental factors, generating measurable signals such as fluorescence or luminescence. Real-time metabolite monitoring has been achieved in genetically engineered C. vulgaris, where fluorescent biosensors tracked lipid accumulation, allowing for dynamic adjustments in cultivation conditions (Antonacci and Scognamiglio, 2020; Patwari et al., 2023).
Moreover, biosensors have been employed to optimize nitrate and phosphorus utilization in photobioreactors. For instance, nitrate-responsive biosensors in Synechococcus elongatus provided real-time data on nutrient availability, enabling precise adjustments to nutrient supply and improving nitrogen use efficiency (Hasan et al., 2024). Another innovation involved the use of biosensors for detecting reactive oxygen species (ROS), which helped monitor oxidative stress in C. reinhardtii, ensuring optimal cultivation conditions (Molen et al., 2006).
High-throughput screening enabled by biosensors has accelerated the identification of high-performing genetic variants. For example, a biosensor-assisted selection system in P. tricornutum identified strains with enhanced fucoxanthin production, achieving a 40% increase in yield (Macdonald Miller et al., 2023). Additionally, biosensors integrated with wireless systems are being explored for remote monitoring in large-scale outdoor photobioreactors, demonstrating their potential to scale microalgal cultivation (Heining et al., 2015).
Emerging biosensor technologies are revolutionizing the field of synthetic biology in microalgae by introducing advanced detection capabilities and integration with automated systems. One notable advancement is the development of multi-analyte biosensors capable of simultaneously detecting various metabolites, such as glucose, nitrate, and lipid precursors, within a single cultivation system. These biosensors leverage nanoscale materials, including graphene and gold nanoparticles, to achieve high sensitivity and specificity (Huang et al., 2021; Parkhe and Tiwari, 2024).
The application of microfluidic biosensors is another frontier. These devices integrate biosensing components into miniaturized platforms, enabling real-time monitoring of microalgae cultures with minimal sample requirements. For instance, microfluidic chips embedded with biosensors have been used to monitor pH and nutrient gradients in dynamic cultivation environments, providing unparalleled insights into cellular responses under stress conditions (Pattanayak et al., 2021).
Additionally, wearable biosensors have been developed for real-time monitoring of outdoor photobioreactor systems. These devices are equipped with wireless data transmission capabilities, allowing operators to remotely monitor critical parameters such as oxygen evolution and light absorption efficiency. Such technologies are particularly valuable for large-scale operations where manual monitoring is impractical (Xu et al., 2024).
Another promising innovation involves the integration of biosensors with CRISPR-based detection systems. These CRISPR-biosensors exploit the sequence-specific detection capabilities of CRISPR-Cas systems to identify genetic markers indicative of desired traits or stress responses. For example, CRISPR-biosensors have been employed to detect the activation of lipid biosynthesis pathways in Nannochloropsis cultures, enhancing the precision of metabolic engineering efforts (Xie et al., 2024).
These emerging technologies are poised to significantly enhance the scalability, efficiency, and precision of microalgae cultivation. By integrating these biosensors into synthetic biology workflows, researchers can achieve more robust control over metabolic pathways, optimize cultivation conditions, and expand the industrial applicability of microalgae-derived products.
Despite these advancements, several challenges remain in scaling synthetic biology applications for microalgae. Photobioreactor design and operation present a major hurdle, as scaling up these systems requires maintaining consistent light exposure, temperature, and nutrient distribution. Current large-scale systems often struggle with suboptimal light penetration and uneven CO2 distribution, which reduces overall productivity. To overcome these issues, advancements in photobioreactor design, such as modular systems with adaptive lighting technologies, are necessary (Huang et al., 2017; Patwari et al., 2023). Additionally, high production costs associated with genetic engineering, cultivation, and downstream processing challenge the economic feasibility of synthetic biology applications. While techniques like CRISPR-Cas9 are effective, they remain costly for industrial-scale deployment, making the development of cost-effective genome-editing tools and high-throughput screening methods crucial (Sarkar et al., 2023).
The genomic complexity and resource limitations of many microalgae species also hinder the optimization of metabolic pathways. Limited genomic resources, including incomplete genome annotations and poorly understood regulatory networks, are a significant barrier that can be overcome with improved genomic databases and functional annotation pipelines (Stavridou et al., 2024). Regulatory and biosafety concerns arise when deploying genetically modified microalgae in open environments for biofuel or environmental applications. Developing stricter international guidelines and risk assessment protocols is essential to ensure safe and sustainable use (Henley et al., 2013).
The scalability of cultivation systems is another challenge, as the transition from laboratory to industrial-scale cultivation introduces variability in growth rates, biomass yields, and metabolic performance. Advanced photobioreactor designs and innovative cultivation strategies are needed to ensure consistent performance across different scales (Dharmaraja et al., 2023). Lastly, public acceptance and market integration are critical, as consumer skepticism regarding genetically modified organisms (GMOs) may slow the adoption of synthetic biology-derived products. Public awareness campaigns that highlight the environmental and economic benefits of these innovations will be crucial to gaining market acceptance (Hwang and Nam, 2021).
Addressing these challenges requires interdisciplinary collaborations among biologists, engineers, and computational scientists. Future innovations will likely focus on more efficient genetic tools, enhanced metabolic engineering techniques, and robust systems for scaling production.
Microalgae-based innovations, despite their immense potential, face a range of challenges across technical, economic, consumer acceptance and regulatory dimensions that must be addressed to achieve large-scale adoption and practical application. Fortunately, solutions to these barriers are emerging, paving the way for scalable and sustainable applications.
The technical challenges and solutions in microalgae-based innovations are multifaceted. Cultivation constraints are a significant hurdle, as large-scale cultivation of microalgae is complex. Open-pond systems, while cost-effective, are prone to contamination and environmental variability. In contrast, closed photobioreactors offer better control but come with significant capital and operational costs (Jerney and Spilling, 2020; Novoveská et al., 2023). Solutions to these constraints include the development of advanced modular photobioreactors with adaptive lighting systems, which optimize light distribution and reduce energy consumption. Additionally, integrating CO2 capture technologies with cultivation systems can enhance resource utilization efficiency (Gupta et al., 2015; Skifa et al., 2025). Genetic stability is another challenge, as genetic modifications in engineered microalgae often face stability issues across multiple cultivation cycles. To overcome this, researchers are focusing on stable genome editing techniques, such as base editing and epigenetic modifications, to minimize off-target effects while preserving productivity. Advanced bioinformatics tools are also being used to predict and mitigate genetic instability (Jeong et al., 2023; Onn et al., 2024). Lastly, in the context of drug delivery systems, improving the encapsulation efficiency and controlled release profiles of microalgae-based carriers is technically demanding. Nanotechnology-based drug encapsulation and controlled-release systems, coupled with machine learning algorithms for optimizing release kinetics, are being developed to enhance drug delivery efficiency (Kang et al., 2024; Wang X. et al., 2024).
High production costs are a significant hurdle, especially for microalgae-derived products like β-glucans and engineered therapeutic compounds. Efforts to address these challenges include the integration of co-products, such as biofuels and animal feed, to improve overall economic viability. Additionally, leveraging public-private partnerships can provide funding and reduce the economic risks associated with large-scale production (Wang X. et al., 2024; Skifa et al., 2025). Market integration is another challenge. Competing with established agricultural and chemical synthesis methods remains challenging. Developing strong marketing strategies highlighting the environmental benefits and health advantages of microalgae products can help penetrate competitive markets. Certification schemes and eco-labels emphasizing sustainability are being explored to attract eco-conscious consumers (Ramadas et al., 2021; Zhong et al., 2023).
The commercialization of microalgae in healthcare and functional foods has gained momentum due to their nutritional benefits and potential to address global health challenges. However, consumer acceptance remains a critical factor in determining their market success. Key elements influencing consumer acceptance include taste, cost-effectiveness, and public perception, each of which plays a pivotal role in shaping purchasing decisions and long-term adoption.
Taste is one of the most significant barriers to consumer acceptance of microalgae-based products. Microalgae, such as Spirulina and Chlorella, often have distinct flavors that can be perceived as unpleasant, such as earthy, fishy, or bitter notes (Becker, 2007). These sensory characteristics can deter consumers. To overcome this challenge, manufacturers are increasingly investing in flavor-masking technologies and incorporating microalgae into familiar food formats, such as snacks, beverages, and baked goods. For example, Spirulina has been successfully integrated into smoothies and energy bars, where its flavor is less pronounced (Caporgno and Mathys, 2018). Cost-effectiveness is another critical factor influencing consumer acceptance. Microalgae cultivation and processing can be expensive due to the need for controlled environments, specialized equipment, and high-quality inputs. These costs are often passed on to consumers, resulting in higher retail prices compared to conventional functional foods (Çelekli et al., 2024). To address this, advancements in cultivation technologies, such as photobioreactors and waste-based nutrient sources, are being explored to reduce production costs and improve affordability (Taparia et al., 2016). Public perception of microalgae-based products also plays a crucial role in their commercial success. Despite their nutritional benefits, microalgae are often perceived as unconventional or unappealing, particularly in Western markets where they are not traditionally consumed (Plaza et al., 2008). Misconceptions about safety, sustainability, and efficacy can further hinder consumer acceptance. Effective communication strategies, including clear labeling, educational campaigns, and endorsements from trusted health authorities, are essential to build trust and awareness. For instance, highlighting the environmental sustainability of microalgae cultivation, such as their low carbon footprint and ability to grow in non-arable land, can appeal to environmentally conscious consumers (Borowitzka, 2013).
The utilization of microalgae in therapeutics and functional foods has gained significant attention due to their rich nutritional profiles and potential health benefits. However, the regulatory landscape for these products varies considerably across different regions, posing unique challenges for market entry and compliance. In the United States, microalgae-based products are regulated by the Food and Drug Administration (FDA) under the categories of dietary supplements, food additives, or drugs, depending on their intended use. For instance, Spirulina and Chlorella are generally recognized as safe for use in foods, but any health claims must be substantiated by rigorous clinical trials (Hosny et al., 2025). The FDA’s stringent requirements for safety and efficacy data can be a significant hurdle, particularly for novel microalgae strains or extracts. In the European Union (EU), microalgae products fall under the jurisdiction of the European Food Safety Authority (EFSA). Health claims related to microalgae must be approved under the EU Health Claims Regulation, which demands robust scientific evidence, often involving human intervention studies (Bigliardi and Galati, 2013). This process can be time-consuming and costly, particularly for small and medium-sized enterprises. In Asia, particularly in countries like China and Japan, the regulatory environment is somewhat more accommodating but still complex (Patel et al., 2008). The approval process for new functional foods can be lengthy, requiring extensive documentation and testing. Japan, on the other hand, has a well-established system for functional foods under the Foods with Function Claims (FFC) system, which allows for a more streamlined approval process. However, the specific requirements for microalgae-derived products can still be challenging to navigate, particularly regarding the substantiation of health benefits. In developing regions, such as parts of Africa and South America, the regulatory frameworks for microalgae-based products are often less developed. This can lead to inconsistencies in enforcement and a lack of clarity for manufacturers. However, the absence of stringent regulations can also provide opportunities for rapid market entry, albeit with potential risks related to product quality and safety (Salehipour-Bavarsad et al., 2024).
Regulatory challenges in the microalgae biotechnology sector require thoughtful solutions. The approval processes for microalgae-based products are still evolving. Harmonizing international regulatory standards and creating streamlined safety assessment protocols for genetically modified strains can reduce barriers to market entry. Collaborations between regulatory bodies and researchers are vital to accelerate approval processes (Herrmann et al., 2021; Onn et al., 2024). Additionally, public perception plays a significant role, as consumer skepticism toward genetically modified organisms (GMOs) can hinder acceptance. Public outreach campaigns and transparent labeling practices are being implemented to improve consumer trust. Demonstrating clear safety profiles through large-scale clinical studies is also key to alleviating skepticism (Ramadas et al., 2021; Kang et al., 2024). By addressing these challenges through technological advancements, economic strategies, and regulatory alignment, the field of microalgae biotechnology can unlock its full potential for transformative impact.
The integration of microalgae into biotechnology represents a transformative opportunity to address global challenges in health, nutrition, and sustainability. Recent advancements in microalgae research have highlighted three critical areas for future development: technological innovations, sustainability approaches, and collaborative networks. These interconnected strategies are key to overcoming current limitations and unlocking the full potential of microalgae-based applications.
Technological advancements are reshaping the scalability and precision of microalgae cultivation. The development of advanced photobioreactors, equipped with adaptive lighting systems and modular designs, has significantly improved light utilization efficiency, reducing energy consumption and operational costs. For instance, modular photobioreactors used in large-scale C. vulgaris production demonstrated a 30% increase in biomass yield under controlled light conditions compared to conventional systems (Penloglou et al., 2024). Moreover, genome-editing tools, such as CRISPR-Cas9 and TALENs, are enabling precise metabolic modifications in microalgae (Jeon et al., 2017). A recent study on N. oceanica reported a 40% enhancement in lipid productivity through targeted pathway optimization (Liu et al., 2024). These technologies not only enhance production efficiency but also broaden the spectrum of valuable compounds derived from microalgae, including biofuels, nutraceuticals, and therapeutic proteins.
Integrating microalgae cultivation with environmental sustainability initiatives offers dual benefits: economic feasibility and ecological impact. Microalgae-based carbon capture systems are being developed to mitigate industrial CO2 emissions while simultaneously promoting biomass growth. For example, a pilot project in Germany combined microalgae bioreactors with cement factory emissions, achieving a 50% reduction in carbon output while producing valuable algal biomass for biofuel production (He Z. et al., 2024). Additionally, using microalgae for wastewater treatment has proven effective in recovering nutrients and reducing contaminants. S. obliquus cultivation in municipal wastewater not only reduced nitrogen and phosphorus levels by over 80% but also generated algal biomass rich in proteins and lipids (de Morais et al., 2023). These integrated approaches underscore the economic and environmental potential of microalgae as a sustainable resource.
The rapid adoption and innovation in microalgae biotechnology depend on strong partnerships between academia, industry, and regulatory bodies. Collaborative networks facilitate knowledge exchange, resource sharing, and streamlined commercialization pathways. For instance, public-private partnerships in Asia have accelerated the development of genetically modified microalgae for aquaculture feed, addressing regional food security challenges (Ahmad and Ashraf, 2024). Establishing international regulatory standards through such collaborations will further reduce barriers to market entry and promote global acceptance of microalgae-based innovations.
Microalgae-based innovations in β-glucan production, drug delivery systems and synthetic biology applications hold transformative potential. These advancements not only promise revolutionary changes in health, nutrition, and environmental sustainability but also set a precedent for biotechnological solutions to global challenges.
The utilization of β-glucans underscores microalgae’s inherent bioactivity, with applications ranging from immune enhancement to metabolic health. These natural compounds, particularly paramylon from Euglena, showcase the potential to bridge functional foods and therapeutic innovations. Synthetic biology has further amplified microalgae’s role in biotechnology by providing precise tools to enhance productivity and expand applications. Tools like CRISPR-Cas9 and TALENs have opened avenues for producing high-value compounds such as biofuels, pigments, and pharmaceuticals, while reducing costs and improving scalability.
Environmental sustainability remains a cornerstone of microalgae’s appeal. Integrating carbon capture and wastewater treatment with microalgae cultivation demonstrates dual benefits: reducing environmental pollutants and generating valuable biomass. Successful case studies, such as nutrient recovery in wastewater or CO2 mitigation in industrial emissions, exemplify microalgae’s potential for circular economy initiatives.
Finally, collaborative networks between academia, industry, and regulatory bodies are crucial. These partnerships accelerate innovation, streamline commercialization, and enhance consumer trust through transparent communication. For instance, international consortia have developed cost-efficient methods for microalgal production, making it feasible for widespread applications in nutraceuticals and beyond.
By addressing technical, economic, and regulatory challenges through targeted solutions, microalgae biotechnology stands at the forefront of sustainable and impactful innovation. This comprehensive integration of bioactive research, engineering, and practical applications positions microalgae as a critical contributor to future global sustainability and health advancements.
CL: Conceptualization, Investigation, Supervision, Writing–original draft, Writing–review and editing. MD: Investigation, Writing–original draft, Writing–review and editing. YH: Investigation, Writing–original draft. WS: Investigation, Writing–original draft. ZC: Supervision, Writing–review and editing. QL: Supervision, Writing–review and editing. HZ: Supervision, Writing–review and editing. LZ: Supervision, Writing–review and editing. SL: Supervision, Writing–review and editing. JW: Funding acquisition, Methodology, Project administration, Resources, Supervision, Writing–original draft, Writing–review and editing.
The author(s) declare that financial support was received for the research, authorship, and/or publication of this article. This project is supported by the Shenzhen Science and Technology Program (KCXST20221021111206015), the National Key R&D Program of China (2021YFA0910800), and the National Natural Science Foundation of China (41876188).
The authors gratefully acknowledge the supports from the Instrumental Analysis Center of Shenzhen University (Xili Campus).
The authors declare that the research was conducted in the absence of any commercial or financial relationships that could be construed as a potential conflict of interest.
The author(s) declared that they were an editorial board member of Frontiers, at the time of submission. This had no impact on the peer review process and the final decision.
The author(s) declare that Generative AI was used in the creation of this manuscript. The authors acknowledge the use of ChatGPT, version 4, a generative AI technology developed by OpenAI, in the preparation of this manuscript for language only.
All claims expressed in this article are solely those of the authors and do not necessarily represent those of their affiliated organizations, or those of the publisher, the editors and the reviewers. Any product that may be evaluated in this article, or claim that may be made by its manufacturer, is not guaranteed or endorsed by the publisher.
Adamo, G., Fierli, D., Romancino, D. P., Picciotto, S., Barone, M. E., Aranyos, A., et al. (2021). Nanoalgosomes: introducing extracellular vesicles produced by microalgae. J. Extracell. Vesicles 10 (6), e12081. doi:10.1002/jev2.12081
Adamo, G., Santonicola, P., Picciotto, S., Gargano, P., Nicosia, A., Longo, V., et al. (2024). Extracellular vesicles from the microalga Tetraselmis chuii are biocompatible and exhibit unique bone tropism along with antioxidant and anti-inflammatory properties. Commun. Biol. 7 (1), 941. doi:10.1038/s42003-024-06612-9
Ahmad, A., and Ashraf, S. S. (2024). Harnessing microalgae: innovations for achieving UN sustainable development goals and climate resilience. J. Water Process Eng. 68, 106506. doi:10.1016/j.jwpe.2024.106506
Ambati, R. R., Phang, S. M., Ravi, S., and Aswathanarayana, R. G. (2014). Astaxanthin: sources, extraction, stability, biological activities and its commercial applications--a review. Mar. Drugs 12 (1), 128–152. doi:10.3390/md12010128
Ambily, B., Limna Mol, V. P., Sini, H., and Nevin, K. G. (2024). CRISPR-based microalgal genome editing and the potential for sustainable aquaculture: a comprehensive review. J. Appl. Phycol. doi:10.1007/s10811-024-03376-x
Ampofo, J., and Abbey, L. (2022). Microalgae: bioactive composition, health benefits, safety and prospects as potential high-value ingredients for the functional food industry. Foods 11 (12), 1744. doi:10.3390/foods11121744
Antonacci, A., and Scognamiglio, V. (2020). Biotechnological advances in the design of algae-based biosensors. Trends Biotechnol. 38 (3), 334–347. doi:10.1016/j.tibtech.2019.10.005
Aoe, S., Kawano, T., Naito, J., Nishida, N., and Takahashi, M. (2023). Effects of paramylon-rich Euglena gracilis EOD-1 powder on visceral fat obesity in moderately obese Japanese adults: a randomized, double-blind, placebo-controlled, parallel-group trial. Food Sci. Nutr. 11 (2), 953–962. doi:10.1002/fsn3.3130
Aoe, S., Yamanaka, C., and Mio, K. (2021). Microarray analysis of paramylon, isolated from Euglena gracilis EOD-1, and its effects on lipid metabolism in the ileum and liver in diet-induced obese mice. Nutrients 13 (10), 3406. doi:10.3390/nu13103406
Aslam, S., Ahmad, M., and Riaz, M. (2021). “Stability of carotenoids,” in Carotenoids: structure and function in the human body. Editors M. Zia-Ul-Haq, S. Dewanjee, and M. Riaz (Springer International Publishing), 251–315. doi:10.1007/978-3-030-46459-2_8
Baek, K., Kim, D. H., Jeong, J., Sim, S. J., Melis, A., Kim, J.-S., et al. (2016). DNA-free two-gene knockout in Chlamydomonas reinhardtii via CRISPR-Cas9 ribonucleoproteins. Sci. Rep. 6 (1), 30620. doi:10.1038/srep30620
Barclay, W. R., Meager, K. M., and Abril, J. R. (1994). Heterotrophic production of long chain omega-3 fatty acids utilizing algae and algae-like microorganisms. J. Appl. Phycol. 6 (2), 123–129. doi:10.1007/BF02186066
Barsanti, L., and Gualtieri, P. (2019). Paramylon, a potent immunomodulator from WZSL mutant of Euglena gracilis. Molecules 24 (17), 3114. doi:10.3390/molecules24173114
Bashir, K. M. I., and Choi, J. S. (2017). Clinical and physiological perspectives of β-glucans: the past, present, and future. Int. J. Mol. Sci. 18 (9), 1906. doi:10.3390/ijms18091906
Becker, E. W. (2007). Micro-algae as a source of protein. Biotechnol. Adv. 25 (2), 207–210. doi:10.1016/j.biotechadv.2006.11.002
Ben-Amotz, A., and Levy, Y. (1996). Bioavailability of a natural isomer mixture compared with synthetic all-trans beta-carotene in human serum. Am. J. Clin. Nutr. 63 (5), 729–734. doi:10.1093/ajcn/63.5.729
Bigliardi, B., and Galati, F. (2013). Innovation trends in the food industry: the case of functional foods. Trends Food Sci. Tech., 31(2), 118–129. doi:10.1016/j.tifs.2013.03.006
Boisset, N. D., Favoino, G., Meloni, M., Jomat, L., Cassier-Chauvat, C., Zaffagnini, M., et al. (2023). Phosphoribulokinase abundance is not limiting the Calvin-Benson-Bassham cycle in Chlamydomonas reinhardtii. Front. Plant Sci. 14, 1230723. doi:10.3389/fpls.2023.1230723
Borowitzka, M. A. (2013). High-value products from microalgae—their development and commercialisation. J. Appl. Phycol. 25 (3), 743–756. doi:10.1007/s10811-013-9983-9
Cao, K., Cui, Y., Sun, F., Zhang, H., Fan, J., Ge, B., et al. (2023). Metabolic engineering and synthetic biology strategies for producing high-value natural pigments in Microalgae. Biotechnol. Adv. 68, 108236. doi:10.1016/j.biotechadv.2023.108236
Caporgno, M. P., and Mathys, A. (2018). Trends in microalgae incorporation into innovative food products with potential health benefits. Front. Nutr. 5, 58. doi:10.3389/fnut.2018.00058
Çelekli, A., Özbal, B., and Bozkurt, H. (2024). Challenges in functional food products with the incorporation of some microalgae. Foods 13 (5), 725. doi:10.3390/foods13050725
Chen, Z., Zhu, J., Chen, Z., Du, M., Yao, R., Fu, W., et al. (2022a). High-throughput sequencing revealed low-efficacy genome editing using Cas9 RNPs electroporation and single-celled microinjection provided an alternative to deliver CRISPR reagents into Euglena gracilis. Plant Biotechnol. J. 20 (11), 2048–2050. doi:10.1111/pbi.13915
Chen, Z., Zhu, J., Du, M., Chen, Z., Liu, Q., Zhu, H., et al. (2022b). A synthetic biology perspective on the bioengineering tools for an industrial microalga: Euglena gracilis. Front. Bioeng. Biotechnol. 10, 882391. doi:10.3389/fbioe.2022.882391
Chiba, H., Manabe, N., Naito, J., Nishida, N., Ohno, N., and Yamaguchi, Y. (2024). A convenient assay for soluble Dectin-1 lectin domain binding to insoluble β-glucans. Carbohydr. Res. 536, 109041. doi:10.1016/j.carres.2024.109041
Chiozzi, V., Eliopoulos, C., Markou, G., Arapoglou, D., Agriopoulou, S., El Enshasy, H. A., et al. (2021). Biotechnological addition of β-glucans from cereals, mushrooms and yeasts in foods and animal feed. Processes 9 (11), 1889. doi:10.3390/pr9111889
Cui, Y., Zhu, L., Li, Y., Jiang, S., Sun, Q., Xie, E., et al. (2021). Structure of a laminarin-type β-(1→3)-glucan from brown algae Sargassum henslowianum and its potential on regulating gut microbiota. Carbohydr. Polym. 255, 117389. doi:10.1016/j.carbpol.2020.117389
Dai, J., He, J., Chen, Z., Qin, H., Du, M., Lei, A., et al. (2022). Euglena gracilis promotes lactobacillus growth and antioxidants accumulation as a potential next-generation prebiotic. Front. Nutr. 9, 864565. doi:10.3389/fnut.2022.864565
Delalat, B., Sheppard, V. C., Rasi Ghaemi, S., Rao, S., Prestidge, C. A., McPhee, G., et al. (2015). Targeted drug delivery using genetically engineered diatom biosilica. Nat. Commun. 6 (1), 8791. doi:10.1038/ncomms9791
de Morais, E. G., Sampaio, I. C. F., Gonzalez-Flo, E., Ferrer, I., Uggetti, E., and García, J. (2023). Microalgae harvesting for wastewater treatment and resources recovery: a review. N. Biotechnol. 78, 84–94. doi:10.1016/j.nbt.2023.10.002
Dharmaraja, J., Shobana, S., Huy, M., Vatland, A. K., Ashokkumar, V., and Kumar, G. (2023). “Chapter 2 - design and scale-up of photobioreactors,” in Current developments in biotechnology and bioengineering. Editors R. Sirohi, A. Pandey, S. Sim, J.-S. Chang, and D.-J. Lee (Elsevier), 11–32. doi:10.1016/B978-0-323-99911-3.00010-5
Dubey, K. K., Kumar, A., Baldia, A., Rajput, D., Kateriya, S., Singh, R., et al. (2023). Biomanufacturing of glycosylated antibodies: challenges, solutions, and future prospects. Biotechnol. Adv. 69, 108267. doi:10.1016/j.biotechadv.2023.108267
Fayyaz, M., Chew, K. W., Show, P. L., Ling, T. C., Ng, I. S., and Chang, J.-S. (2020). Genetic engineering of microalgae for enhanced biorefinery capabilities. Biotechnol. Adv. 43, 107554. doi:10.1016/j.biotechadv.2020.107554
Fernández, F. G. A., Reis, A., Wijffels, R. H., Barbosa, M., Verdelho, V., and Llamas, B. (2021). The role of microalgae in the bioeconomy. New Biotechnol. 61, 99–107. doi:10.1016/j.nbt.2020.11.011
Feuzing, F., Mbakidi, J. P., Marchal, L., Bouquillon, S., and Leroy, E. (2022). A review of paramylon processing routes from microalga biomass to non-derivatized and chemically modified products. Carbohydr. Polym. 288, 119181. doi:10.1016/j.carbpol.2022.119181
Frick, K., Ebbing, T., Yeh, Y.-C., Schmid-Staiger, U., and Tovar, G. E. M. (2023). Beta-glucan production of Phaeodactylum tricornutum, Monodopsis subterranea and Cylindrotheca fusiformis during nitrogen depletion. J. Appl. Phycol. 35 (6), 2607–2618. doi:10.1007/s10811-023-03026-8
Fujita, T., Aoyagi, H., Ogbonna, J. C., and Tanaka, H. (2008). Effect of mixed organic substrate on alpha-tocopherol production by Euglena gracilis in photoheterotrophic culture. Appl. Microbiol. Biotechnol. 79 (3), 371–378. doi:10.1007/s00253-008-1443-0
Gao, L., Zhao, X., Liu, M., and Zhao, X. (2022). Characterization and antibacterial activities of carboxymethylated paramylon from Euglena gracilis. Polym. (Basel) 14 (15), 3022. doi:10.3390/polym14153022
Geng, H., Chen, M., Guo, C., Wang, W., and Chen, D. (2024). Marine polysaccharides: biological activities and applications in drug delivery systems. Carbohydr. Res. 538, 109071. doi:10.1016/j.carres.2024.109071
Gissibl, A., Sun, A., Care, A., Nevalainen, H., and Sunna, A. (2019). Bioproducts from Euglena gracilis: synthesis and applications. Front. Bioeng. Biotechnol. 7, 108. doi:10.3389/fbioe.2019.00108
Grama, S. B., Liu, Z., and Li, J. (2022). Emerging trends in genetic engineering of microalgae for commercial applications. Mar. Drugs 20 (5), 285. doi:10.3390/md20050285
Gu, Y., Teo, M. Y. M., In, L. L. A., Shimizu, K., Chae, K.-J., Ngoc Thu Tran, T., et al. (2024). Genetic engineering of Haematococcus pluvialis microalgae for the enhancement of astaxanthin production: a review. Biocatal. Agric. Biotechnol. 60, 103298. doi:10.1016/j.bcab.2024.103298
Guo, Q., Bi, D., Wu, M., Yu, B., Hu, L., Liu, C., et al. (2020). Immune activation of murine RAW264.7 macrophages by sonicated and alkalized paramylon from Euglena gracilis. BMC Microbiol. 20 (1), 171. doi:10.1186/s12866-020-01782-y
Gupta, P. L., Lee, S. M., and Choi, H. J. (2015). A mini review: photobioreactors for large scale algal cultivation. World J. Microbiol. Biotechnol. 31 (9), 1409–1417. doi:10.1007/s11274-015-1892-4
Guzmán, S., Gato, A., and Calleja, J. M. (2001). Antiinflammatory, analgesic and free radical scavenging activities of the marine microalgae Chlorella stigmatophora and Phaeodactylum tricornutum. Phytother. Res. 15 (3), 224–230. doi:10.1002/ptr.715
Hasan, R., Kasera, N., Beck, A. E., and Hall, S. G. (2024). Potential of Synechococcus elongatus UTEX 2973 as a feedstock for sugar production during mixed aquaculture and swine wastewater bioremediation. Heliyon 10 (3), e24646. doi:10.1016/j.heliyon.2024.e24646
Hayatgheib, N., Moreau, E., Calvez, S., Lepelletier, D., and Pouliquen, H. (2020). A review of functional feeds and the control of Aeromonas infections in freshwater fish. Aquac. Int. 28 (3), 1083–1123. doi:10.1007/s10499-020-00514-3
He, L., Zhu, Z., and Qi, C. (2024a). β-Glucan-A promising immunocyte-targeting drug delivery vehicle: superiority, applications and future prospects. Carbohydr. Polym. 339, 122252. doi:10.1016/j.carbpol.2024.122252
He, Z., Wang, J., and Li, Y. (2024b). Recent advances in microalgae-driven carbon capture, utilization, and storage: strain engineering through adaptive laboratory evolution and microbiome optimization. Green Carbon. doi:10.1016/j.greenca.2024.10.001
Heining, M., Sutor, A., Stute, S. C., Lindenberger, C. P., and Buchholz, R. (2015). Internal illumination of photobioreactors via wireless light emitters: a proof of concept. J. Appl. Phycol. 27 (1), 59–66. doi:10.1007/s10811-014-0290-x
Henley, W. J., Litaker, R. W., Novoveská, L., Duke, C. S., Quemada, H. D., and Sayre, R. T. (2013). Initial risk assessment of genetically modified (GM) microalgae for commodity-scale biofuel cultivation. Algal Res. 2 (1), 66–77. doi:10.1016/j.algal.2012.11.001
Henrion, M., Francey, C., Lê, K. A., and Lamothe, L. (2019). Cereal B-glucans: the impact of processing and how it affects physiological responses. Nutrients 11 (8), 1729. doi:10.3390/nu11081729
Herrmann, I. K., Wood, M. J. A., and Fuhrmann, G. (2021). Extracellular vesicles as a next-generation drug delivery platform. Nat. Nanotechnol. 16 (7), 748–759. doi:10.1038/s41565-021-00931-2
Hosny, S., Elshobary, M. E., and El-Sheekh, M. M. (2025). Unleashing the power of microalgae: a pioneering path to sustainability and achieving the sustainable development goals. Environ. Sci. Pollut. Res. Int. doi:10.1007/s11356-025-35885-8
Hu, J., Wang, D., Chen, H., and Wang, Q. (2023). Advances in genetic engineering in improving photosynthesis and microalgal productivity. Int. J. Mol. Sci. 24 (3), 1898. doi:10.3390/ijms24031898
Huang, H., Lang, Y., and Zhou, M. (2024). A comprehensive review on medical applications of microalgae. Algal Res. 80, 103504. doi:10.1016/j.algal.2024.103504
Huang, Q., Jiang, F., Wang, L., and Yang, C. (2017). Design of photobioreactors for mass cultivation of photosynthetic organisms. Engineering 3 (3), 318–329. doi:10.1016/J.ENG.2017.03.020
Huang, X., Wen, Y., Chen, Y., Liu, Y., and Zhao, C. (2023). Structural characterization of Euglena gracilis polysaccharide and its in vitro hypoglycemic effects by alleviating insulin resistance. Int. J. Biol. Macromol. 236, 123984. doi:10.1016/j.ijbiomac.2023.123984
Huang, X., Zhu, Y., and Kianfar, E. (2021). Nano Biosensors: properties, applications and electrochemical techniques. J. Mater Res. Technol. 12, 1649–1672. doi:10.1016/j.jmrt.2021.03.048
Hwang, H., and Nam, S. J. (2021). The influence of consumers' knowledge on their responses to genetically modified foods. Gm. Crops Food 12 (1), 146–157. doi:10.1080/21645698.2020.1840911
Hyrslova, I., Krausova, G., Mrvikova, I., Stankova, B., Branyik, T., Malinska, H., et al. (2022). Functional properties of Dunaliella salina and its positive effect on probiotics. Mar. Drugs 20 (12), 781. doi:10.3390/md20120781
Ieiri, H., Kameda, N., Naito, J., Kawano, T., Nishida, N., Takahashi, M., et al. (2021). Paramylon extracted from Euglena gracilis EOD-1 augmented the expression of SIRT1. Cytotechnology 73 (5), 755–759. doi:10.1007/s10616-021-00494-z
Ishibashi, K. I., Onaka, N., Nishida, N., Takahashi, M., Adachi, Y., and Ohno, N. (2022). Dectin-1 reactivity to paramylon derived from Euglena gracilis EOD-1. Biol. Pharm. Bull. 45 (9), 1394–1397. doi:10.1248/bpb.b22-00247
Jeon, S., Lim, J.-M., Lee, H.-G., Shin, S.-E., Kang, N. K., Park, Y.-I., et al. (2017). Current status and perspectives of genome editing technology for microalgae. Biotechnol. Biofuels 10 (1), 267. doi:10.1186/s13068-017-0957-z
Jeong, B.-r., Jang, J., and Jin, E. (2023). Genome engineering via gene editing technologies in microalgae. Bioresour. Technol. 373, 128701. doi:10.1016/j.biortech.2023.128701
Jerney, J., and Spilling, K. (2020). Large scale cultivation of microalgae: open and closed systems. Methods Mol. Biol. 1980, 1–8. doi:10.1007/7651_2018_130
Jo, S. H., Jo, K. A., Park, S. Y., and Kim, J. Y. (2024). Unveiling immunomodulatory effects of Euglena gracilis in immunosuppressed mice: transcriptome and pathway analysis. J. Microbiol. Biotechnol. 34 (4), 880–890. doi:10.4014/jmb.2401.01006
Kadam, S. U., Tiwari, B. K., and O'Donnell, C. P. (2015). Extraction, structure and biofunctional activities of laminarin from brown algae. Int. J. Food Sci. Technol. 50 (1), 24–31. doi:10.1111/ijfs.12692
Kang, W., Xu, Z., Lu, H., Liu, S., Li, J., Ding, C., et al. (2024). Advances in biomimetic nanomaterial delivery systems: harnessing nature's inspiration for targeted drug delivery. J. Mater Chem. B 12 (29), 7001–7019. doi:10.1039/D4TB00565A
Kapoor, D. U., Kukkar, M. R., Gaur, M., Prajapati, B. G., Suttiruengwong, S., and Sriamornsak, P. (2024). Algae as third-generation materials: exploring the emerging role in pharmaceutical applications. Mater Today Sustain. 27, 100935. doi:10.1016/j.mtsust.2024.100935
Khozin-Goldberg, I., Iskandarov, U., and Cohen, Z. (2011). LC-PUFA from photosynthetic microalgae: occurrence, biosynthesis, and prospects in biotechnology. Appl. Microbiol. Biotechnol. 91 (4), 905–915. doi:10.1007/s00253-011-3441-x
Kim, K., Ehrlich, A., Perng, V., Chase, J. A., Raybould, H., Li, X. D., et al. (2019). Algae-derived β-glucan enhanced gut health and immune responses of weaned pigs experimentally infected with a pathogenic E. coli. Anim. Feed Sci. Technol. 248, 114–125. doi:10.1016/j.anifeedsci.2018.12.004
Kim, S. K., and Van Ta, Q. (2012). Bioactive sterols from marine resources and their potential benefits for human health. Adv. Food Nutr. Res. 65, 261–268. doi:10.1016/b978-0-12-416003-3.00017-2
Koizumi, N., Sakagami, H., Utsumi, A., Fujinaga, S., Takeda, M., Asano, K., et al. (1993). Anti-HIV (human immunodeficiency virus) activity of sulfated paramylon. Antivir. Res. 21 (1), 1–14. doi:10.1016/0166-3542(93)90063-o
Kumar, V., Bhoyar, M. S., Mohanty, C. S., Chauhan, P. S., Toppo, K., and Ratha, S. K. (2025). Untapping the potential of algae for β-glucan production: a review of biological properties, strategies for enhanced production and future perspectives. Carbohydr. Polym. 348, 122895. doi:10.1016/j.carbpol.2024.122895
Lante, A., Canazza, E., and Tessari, P. (2023). Beta-glucans of cereals: functional and technological properties. Nutrients 15 (9), 2124. doi:10.3390/nu15092124
Lazaridou, A., Biliaderis, C., and Izydorczyk, M. (2007). “Cereal Β-glucans: structures, physical properties and physiological functions,” in Book: functional food carbohydrates, 1–72.
Lei, H., Yu, X., and Fan, D. (2024). Nanocomposite hydrogel for real-time wound status monitoring and comprehensive treatment. Adv. Sci. (Weinh) 11, e2405924. doi:10.1002/advs.202405924
Lei, H., Zhao, J., Li, H., and Fan, D. (2022). Paramylon hydrogel: a bioactive polysaccharides hydrogel that scavenges ROS and promotes angiogenesis for wound repair. Carbohydr. Polym. 289, 119467. doi:10.1016/j.carbpol.2022.119467
Li, H., Wang, K., Tan, M., Zhu, B., and Wang, H. (2024). Carboxymethylation of paramylon derived from Euglena gracilis and its hypoglycemic mechanism in diabetic mice. Int. J. Biol. Macromol. 278 (Pt 4), 134891. doi:10.1016/j.ijbiomac.2024.134891
Li, J., Zheng, Z., Du, M., Chen, J., Zhu, H., Hu, Z., et al. (2021). Euglena gracilis and its aqueous extract constructed with chitosan-hyaluronic acid hydrogel facilitate cutaneous wound healing in mice without inducing excessive inflammatory response. Front. Bioeng. Biotechnol. 9, 713840. doi:10.3389/fbioe.2021.713840
Liang, Y., Kashdan, T., Sterner, C., Dombrowski, L., Petrick, I., Kröger, M., et al. (2015). “Chapter 2 - algal biorefineries,” in Industrial biorefineries and white biotechnology. Editors A. Pandey, R. Höfer, M. Taherzadeh, K. M. Nampoothiri, and C. Larroche (Elsevier), 35–90. doi:10.1016/B978-0-444-63453-5.00002-1
Liu, M., Zheng, J., Yu, L., Shao, S., Zhou, W., and Liu, J. (2024). Engineering Nannochloropsis oceanica for concurrent production of canthaxanthin and eicosapentaenoic acid. Bioresour. Technol. 413, 131525. doi:10.1016/j.biortech.2024.131525
Liu, X., Dong, J., Cui, J., Zheng, Y., Hu, H., Wang, R., et al. (2025). Microalgae-based drug delivery microspheres for treatment of hyperuricemia with renal injury. Nano Today 61, 102607. doi:10.1016/j.nantod.2024.102607
Long, B. M., Rae, B. D., Rolland, V., Förster, B., and Price, G. D. (2016). Cyanobacterial CO2-concentrating mechanism components: function and prospects for plant metabolic engineering. Curr. Opin. Plant Biol. 31, 1–8. doi:10.1016/j.pbi.2016.03.002
Lu, X., Zhao, W., Wang, J., He, Y., Yang, S., and Sun, H. (2024). A comprehensive review on the heterotrophic production of bioactive compounds by microalgae. World J. Microbiol. Biotechnol. 40 (7), 210. doi:10.1007/s11274-024-03892-5
Macdonald Miller, S., Abbriano, R. M., Herdean, A., Banati, R., Ralph, P. J., and Pernice, M. (2023). Random mutagenesis of Phaeodactylum tricornutum using ultraviolet, chemical, and X-ray irradiation demonstrates the need for temporal analysis of phenotype stability. Sci. Rep. 13 (1), 22385. doi:10.1038/s41598-023-45899-2
Merchant, R. E., and Andre, C. A. (2001). A review of recent clinical trials of the nutritional supplement Chlorella pyrenoidosa in the treatment of fibromyalgia, hypertension, and ulcerative colitis. Altern. Ther. Health Med. 7 (3), 79–91.
Molen, T. A., Rosso, D., Piercy, S., and Maxwell, D. P. (2006). Characterization of the alternative oxidase of Chlamydomonas reinhardtii in response to oxidative stress and a shift in nitrogen source. Physiol. Plant 127 (1), 74–86. doi:10.1111/j.1399-3054.2006.00643.x
Monfils, A. K., Triemer, R. E., and Bellairs, E. F. (2011). Characterization of paramylon morphological diversity in photosynthetic euglenoids (Euglenales, Euglenophyta). Phycologia 50 (2), 156–169. doi:10.2216/09-112.1
Murphy, E. J., Rezoagli, E., Collins, C., Saha, S. K., Major, I., and Murray, P. (2023). Sustainable production and pharmaceutical applications of β-glucan from microbial sources. Microbiol. Res. 274, 127424. doi:10.1016/j.micres.2023.127424
Murphy, E. J., Rezoagli, E., Major, I., Rowan, N. J., and Laffey, J. G. (2020). β-Glucan metabolic and immunomodulatory properties and potential for clinical application. J. Fungi (Basel) 6 (4), 356. doi:10.3390/jof6040356
Myklestad, S. M., and Granum, E. (2009). “Chapter 4.2 - biology of (1,3)-β-glucans and related glucans in Protozoans and chromistans,” in Chemistry, biochemistry, and biology of 1-3 beta glucans and related polysaccharides. Editors A. Bacic, G. B. Fincher, and B. A. Stone (Academic Press), 353–385. doi:10.1016/B978-0-12-373971-1.00010-8
Naduthodi, M. I. S., Claassens, N. J., D’Adamo, S., van der Oost, J., and Barbosa, M. J. (2021). Synthetic biology approaches to enhance microalgal productivity. Trends Biotechnol. 39 (10), 1019–1036. doi:10.1016/j.tibtech.2020.12.010
Nagayama, Y., Isoo, N., Nakashima, A., Suzuki, K., Yamano, M., Nariyama, T., et al. (2020). Renoprotective effects of paramylon, a β-1,3-D-Glucan isolated from Euglena gracilis Z in a rodent model of chronic kidney disease. PLoS One 15 (8), e0237086. doi:10.1371/journal.pone.0237086
Nakashima, A., Sasaki, K., Sasaki, D., Yasuda, K., Suzuki, K., and Kondo, A. (2021). The alga Euglena gracilis stimulates Faecalibacterium in the gut and contributes to increased defecation. Sci. Rep. 11 (1), 1074. doi:10.1038/s41598-020-80306-0
Nakashima, A., Sugimoto, R., Suzuki, K., Shirakata, Y., Hashiguchi, T., Yoshida, C., et al. (2019). Anti-fibrotic activity of Euglena gracilis and paramylon in a mouse model of non-alcoholic steatohepatitis. Food Sci. Nutr. 7 (1), 139–147. doi:10.1002/fsn3.828
Nakashima, A., Suzuki, K., Asayama, Y., Konno, M., Saito, K., Yamazaki, N., et al. (2017). Oral administration of Euglena gracilis Z and its carbohydrate storage substance provides survival protection against influenza virus infection in mice. Biochem. Biophys. Res. Commun. 494 (1-2), 379–383. doi:10.1016/j.bbrc.2017.09.167
Nallasamy, P., and Natarajan, S. (2021). “Algal nanotechnology: scope and limitations,” in Bioprospecting algae for nanosized materials. Editors D. Thangadurai, J. Sangeetha, and R. Prasad (Springer International Publishing), 3–22. doi:10.1007/978-3-030-81557-8_1
Negi, S., Perrine, Z., Friedland, N., Kumar, A., Tokutsu, R., Minagawa, J., et al. (2020). Light regulation of light-harvesting antenna size substantially enhances photosynthetic efficiency and biomass yield in green algae. Plant J. 103 (2), 584–603. doi:10.1111/tpj.14751
Nethravathy, M. U., Mehar, J. G., Mudliar, S. N., and Shekh, A. Y. (2019). Recent advances in microalgal bioactives for food, feed, and healthcare products: commercial potential, market space, and sustainability. Compr. Rev. Food Sci. Food Saf. 18 (6), 1882–1897. doi:10.1111/1541-4337.12500
Novoveská, L., Nielsen, S. L., Eroldoğan, O. T., Haznedaroglu, B. Z., Rinkevich, B., Fazi, S., et al. (2023). Overview and challenges of large-scale cultivation of photosynthetic microalgae and cyanobacteria. Mar. Drugs 21 (8), 445. doi:10.3390/md21080445
Okouchi, R., E, S., Yamamoto, K., Ota, T., Seki, K., Imai, M., et al. (2019). Simultaneous intake of Euglena gracilis and vegetables exerts synergistic anti-obesity and anti-inflammatory effects by modulating the gut microbiota in diet-induced obese mice. Nutrients 11 (1), 204. doi:10.3390/nu11010204
Onn, S. M., Koh, G. J., Yap, W. H., Teoh, M.-L., Low, C.-F., and Goh, B.-H. (2024). Recent advances in genetic engineering of microalgae: bioengineering strategies, regulatory challenges and future perspectives. J. Appl. Phycol. doi:10.1007/s10811-024-03367-y
Parkhe, V. S., and Tiwari, A. P. (2024). Gold nanoparticles-based biosensors: pioneering solutions for bacterial and viral pathogen detection-a comprehensive review. World J. Microbiol. Biotechnol. 40 (9), 269. doi:10.1007/s11274-024-04072-1
Patel, D., Dufour, Y., and Domigan, N. (2008). Functional food and nutraceutical registration processes in Japan and China: a diffusion of innovation perspective. J. Pharm. Pharm. Sci. 11 (4), 1–11. doi:10.18433/j32s3n
Patel, V. K., Das, A., Kumari, R., and Kajla, S. (2023). Recent progress and challenges in CRISPR-Cas9 engineered algae and cyanobacteria. Algal Res. 71, 103068. doi:10.1016/j.algal.2023.103068
Patra, J. K., Das, G., Fraceto, L. F., Campos, E. V. R., Rodriguez-Torres, M. d. P., Acosta-Torres, L. S., et al. (2018). Nano based drug delivery systems: recent developments and future prospects. J. Nanobiotechnol 16 (1), 71. doi:10.1186/s12951-018-0392-8
Pattanayak, P., Singh, S. K., Gulati, M., Vishwas, S., Kapoor, B., Chellappan, D. K., et al. (2021). Microfluidic chips: recent advances, critical strategies in design, applications and future perspectives. Microfluid Nanofluid 25 (12), 99. doi:10.1007/s10404-021-02502-2
Patwari, P., Pruckner, F., and Fabris, M. (2023). Biosensors in microalgae: a roadmap for new opportunities in synthetic biology and biotechnology. Biotechnol. Adv. 68, 108221. doi:10.1016/j.biotechadv.2023.108221
Peng, J., Yuan, J. P., Wu, C. F., and Wang, J. H. (2011). Fucoxanthin, a marine carotenoid present in brown seaweeds and diatoms: metabolism and bioactivities relevant to human health. Mar. Drugs 9 (10), 1806–1828. doi:10.3390/md9101806
Penloglou, G., Pavlou, A., and Kiparissides, C. (2024). Recent advancements in photo-bioreactors for microalgae cultivation: a brief overview. Processes 12 (6), 1104. doi:10.3390/pr12061104
Pereira, L., Cotas, J., and Valado, A. (2024). Antioxidants from microalgae and their potential impact on human well-being. Explor Drug Sci. 2 (3), 292–321. doi:10.37349/eds.2024.00048
Phillips, F. C., Jensen, G. S., Showman, L., Tonda, R., Horst, G., and Levine, R. (2019). Particulate and solubilized β-glucan and non-β-glucan fractions of Euglena gracilis induce pro-and anti-inflammatory innate immune cell responses and exhibit antioxidant properties. J. Inflamm. Res. 12, 49–64. doi:10.2147/jir.S191824
Plaza, M., Cifuentes, A., and Ibáñez, E. (2008). In the search of new functional food ingredients from algae. Trends Food Sci. Technol. 19 (1), 31–39. doi:10.1016/j.tifs.2007.07.012
Rakhi, S. F., Reza, A. H. M. M., Davies, B., Wang, J., Qin, J., and Tang, Y. (2025). Improvement of growth and lipid accumulation in microalgae with aggregation-induced emission-based nanomaterials towards sustainable lipid production. Nanoscale 17, 1308–1316. doi:10.1039/D4NR02361G
Ramadas, S., Nyika, J., Yadav, S., Mackolil, J., Prashat G, R., Workie, E., et al. (2021). Consumer Percept. Prefer. towards Genet. Modified (GM) Foods Bibliometric Evid. Policy Imperatives 2021. doi:10.2139/ssrn.3882657
Raposo, M. F., de Morais, R. M., and Bernardo de Morais, A. M. (2013). Bioactivity and applications of sulphated polysaccharides from marine microalgae. Mar. Drugs 11 (1), 233–252. doi:10.3390/md11010233
Reyes Suárez, E., Bugden, S. M., Kai, F. B., Kralovec, J. A., Noseda, M. D., Barrow, C. J., et al. (2008). First isolation and structural determination of cyclic β-(1→2)-glucans from an alga, Chlorella pyrenoidosa. Carbohydr. Res. 343 (15), 2623–2633. doi:10.1016/j.carres.2008.07.009
Romay, C., González, R., Ledón, N., Remirez, D., and Rimbau, V. (2003). C-phycocyanin: a biliprotein with antioxidant, anti-inflammatory and neuroprotective effects. Curr. Protein Pept. Sci. 4 (3), 207–216. doi:10.2174/1389203033487216
Russo, R., Barsanti, L., Evangelista, V., Frassanito, A. M., Longo, V., Pucci, L., et al. (2017). Euglena gracilis paramylon activates human lymphocytes by upregulating pro-inflammatory factors. Food Sci. Nutr. 5 (2), 205–214. doi:10.1002/fsn3.383
Ryan, C., Cao, S., Sekiguchi, M., Haraguchi, A., Murata, A., Nakashima, A., et al. (2023). Euglena gracilis-derived β-glucan paramylon entrains the peripheral circadian clocks in mice. Front. Nutr. 10, 1113118. doi:10.3389/fnut.2023.1113118
Sadovskaya, I., Souissi, A., Souissi, S., Grard, T., Lencel, P., Greene, C. M., et al. (2014). Chemical structure and biological activity of a highly branched (1→3,1→6)-β-d-glucan from Isochrysis galbana. Carbohydr. Polym. 111, 139–148. doi:10.1016/j.carbpol.2014.04.077
Salehipour-Bavarsad, F., Nematollahi, M. A., Pistocchi, R., and Pezzolesi, L. (2024). Algal food safety: possible contaminations, challenges of harmonized quality assessments, and suggested recommendations for the nascent industry of microalgae-based products. Algal Res. 81, 103579. doi:10.1016/j.algal.2024.103579
Salmeán, A. A., Duffieux, D., Harholt, J., Qin, F., Michel, G., Czjzek, M., et al. (2017). Insoluble (1 → 3), (1 → 4)-β-D-glucan is a component of cell walls in brown algae (Phaeophyceae) and is masked by alginates in tissues. Sci. Rep. 7 (1), 2880. doi:10.1038/s41598-017-03081-5
Sánchez, J. F., Fernández-Sevilla, J. M., Acién, F. G., Cerón, M. C., Pérez-Parra, J., and Molina-Grima, E. (2008). Biomass and lutein productivity of Scenedesmus almeriensis: influence of irradiance, dilution rate and temperature. Appl. Microbiol. Biotechnol. 79 (5), 719–729. doi:10.1007/s00253-008-1494-2
Sarkar, S., Yadav, M., and Kumar, A. (2023). “CRISPR/Cas9 system: an advanced approach for the improvement of industrially important microorganisms,” in Industrial microbiology and biotechnology: emerging concepts in microbial technology. Editor P. Verma (Singapore: Springer Nature), 69–97. doi:10.1007/978-981-99-2816-3_4
Scartazza, A., Picciarelli, P., Mariotti, L., Curadi, M., Barsanti, L., and Gualtieri, P. (2017). The role of Euglena gracilis paramylon in modulating xylem hormone levels, photosynthesis and water-use efficiency in Solanum lycopersicum L. Physiol. Plant 161 (4), 486–501. doi:10.1111/ppl.12611
Schulze, C., Wetzel, M., Reinhardt, J., Schmidt, M., Felten, L., and Mundt, S. (2016). Screening of microalgae for primary metabolites including β-glucans and the influence of nitrate starvation and irradiance on β-glucan production. J. Appl. Phycol. 28 (5), 2719–2725. doi:10.1007/s10811-016-0812-9
Sharma, R., Solanki, P., Chaudhary, M., Gupta, N., and Kaur, P. (2024). Unveiling the potential of microalgae for bioplastic production from wastewater – current trends, innovations, and future prospects. Biotechnol. Sustain. Mater. 1 (1), 10. doi:10.1186/s44316-024-00010-1
Shibakami, M., Shibata, K., Akashi, A., Onaka, N., Takezaki, J., Tsubouchi, G., et al. (2018). Creation of straight-chain cationic polysaccharide-based bile salt sequestrants made from euglenoid β-1,3-glucan as potential antidiabetic agents. Pharm. Res. 36 (1), 23. doi:10.1007/s11095-018-2553-8
Shimada, R., Fujita, M., Yuasa, M., Sawamura, H., Watanabe, T., Nakashima, A., et al. (2016). Oral administration of green algae, Euglena gracilis, inhibits hyperglycemia in OLETF rats, a model of spontaneous type 2 diabetes. Food Funct. 7 (11), 4655–4659. doi:10.1039/c6fo00606j
Shin, S. E., Koh, H. G., Park, K., Park, S. H., Chang, Y. K., and Kang, N. K. (2024). Increasing lipid production in Chlamydomonas reinhardtii through genetic introduction for the overexpression of glyceraldehyde-3-phosphate dehydrogenase. Front. Bioeng. Biotechnol. 12, 1396127. doi:10.3389/fbioe.2024.1396127
Skifa, I., Chauchat, N., Cocquet, P.-H., and Guer, Y. L. (2025). Microalgae cultivation in raceway ponds: advances, challenges, and hydrodynamic considerations. EFB Bioeconomy J. 5, 100073. doi:10.1016/j.bioeco.2024.100073
Skov, J., Kania, P. W., Holten-Andersen, L., Fouz, B., and Buchmann, K. (2012). Immunomodulatory effects of dietary β-1,3-glucan from Euglena gracilis in rainbow trout (Oncorhynchus mykiss) immersion vaccinated against Yersinia ruckeri. Fish. Shellfish Immunol. 33 (1), 111–120. doi:10.1016/j.fsi.2012.04.009
Song, Y., Shin, H., Sianipar, H. G. J., Park, J. Y., Lee, M., Hah, J., et al. (2022). Oral administration of Euglena gracilis paramylon ameliorates chemotherapy-induced leukocytopenia and gut dysbiosis in mice. Int. J. Biol. Macromol. 211, 47–56. doi:10.1016/j.ijbiomac.2022.04.168
Song, Y., Wang, F., Chen, L., and Zhang, W. (2024). Engineering fatty acid biosynthesis in microalgae: recent progress and perspectives. Mar. Drugs 22 (5), 216. doi:10.3390/md22050216
Stavridou, E., Karapetsi, L., Nteve, G. M., Tsintzou, G., Chatzikonstantinou, M., Tsaousi, M., et al. (2024). Landscape of microalgae omics and metabolic engineering research for strain improvement: an overview. Aquaculture 587, 740803. doi:10.1016/j.aquaculture.2024.740803
Størseth, T. R., Kirkvold, S., Skjermo, J., and Reitan, K. I. (2006). A branched β-d-(1→3,1→6)-glucan from the marine diatom Chaetoceros debilis (Bacillariophyceae) characterized by NMR. Carbohydr. Res. 341 (12), 2108–2114. doi:10.1016/j.carres.2006.05.005
Su, M., Bastiaens, L., Verspreet, J., and Hayes, M. (2023). Applications of microalgae in foods, pharma and feeds and their use as fertilizers and biostimulants: legislation and regulatory aspects for consideration. Foods 12 (20), 3878. doi:10.3390/foods12203878
Sugiyama, A., Hata, S., Suzuki, K., Yoshida, E., Nakano, R., Mitra, S., et al. (2010). Oral administration of paramylon, a beta-1,3-D-glucan isolated from Euglena gracilis Z inhibits development of atopic dermatitis-like skin lesions in NC/Nga mice. J. Vet. Med. Sci. 72 (6), 755–763. doi:10.1292/jvms.09-0526
Suzuki, K., Nakashima, A., Igarashi, M., Saito, K., Konno, M., Yamazaki, N., et al. (2018). Euglena gracilis Z and its carbohydrate storage substance relieve arthritis symptoms by modulating Th17 immunity. PLoS One 13 (2), e0191462. doi:10.1371/journal.pone.0191462
Taparia, T., Mvss, M., Mehrotra, R., Shukla, P., and Mehrotra, S. (2016). Developments and challenges in biodiesel production from microalgae: a review. Biotechnol. Appl. Biochem. 63 (5), 715–726. doi:10.1002/bab.1412
Taylor, H. B., Gudi, R., Brown, R., and Vasu, C. (2020). Dynamics of structural and functional changes in gut microbiota during treatment with a microalgal β-glucan, paramylon and the impact on gut inflammation. Nutrients 12 (8), 2193. doi:10.3390/nu12082193
Tonon, T., Harvey, D., Larson, T. R., and Graham, I. A. (2002). Long chain polyunsaturated fatty acid production and partitioning to triacylglycerols in four microalgae. Phytochemistry 61 (1), 15–24. doi:10.1016/s0031-9422(02)00201-7
Ullmann, J., and Grimm, D. (2021). Algae and their potential for a future bioeconomy, landless food production, and the socio-economic impact of an algae industry. Org. Agr 11 (2), 261–267. doi:10.1007/s13165-020-00337-9
Ummalyma, S. B., Sahoo, D., and Pandey, A. (2020). “Chapter 12 - microalgal biorefineries for industrial products,” in Microalgae cultivation for biofuels production. Editor A. Yousuf (Academic Press), 187–195. doi:10.1016/B978-0-12-817536-1.00012-6
Uthaiah, N. M., Venkataramareddy, S. R., and Sheikh, A. Y. (2025). Oleaginous microalgae Nannochloropsis sp. as a potential source of EPA and bioactive metabolites: Bottlenecks in the downstream processing and unravelling the challenges in its food, feed and therapeutic application. Algal Res. 86, 103897. doi:10.1016/j.algal.2025.103897
van Steenwijk, H. P., Bast, A., and de Boer, A. (2021). Immunomodulating effects of fungal beta-glucans: from traditional use to medicine. Nutrients 13 (4), 1333. doi:10.3390/nu13041333
Vieira, M. V., Pastrana, L. M., and Fuciños, P. (2020). Microalgae encapsulation systems for food, pharmaceutical and cosmetics applications. Mar. Drugs 18 (12), 644. doi:10.3390/md18120644
Vogler, B. W., Brannum, J., Chung, J. W., Seger, M., and Posewitz, M. C. (2018). Characterization of the Nannochloropsis gaditana storage carbohydrate: a 1,3-beta glucan with limited 1,6-branching. Algal Res. 36, 152–158. doi:10.1016/j.algal.2018.10.011
Wang, H., Zhang, Y., Chen, L., Cheng, W., and Liu, T. (2018). Combined production of fucoxanthin and EPA from two diatom strains Phaeodactylum tricornutum and Cylindrotheca fusiformis cultures. Bioprocess Biosyst. Eng. 41 (7), 1061–1071. doi:10.1007/s00449-018-1935-y
Wang, Q., Jiang, H., Zhang, H., Lu, W., Wang, X., Xu, W., et al. (2024a). β-Glucan-conjugated anti-PD-L1 antibody enhances antitumor efficacy in preclinical mouse models. Carbohydr. Polym. 324, 121564. doi:10.1016/j.carbpol.2023.121564
Wang, X., Ma, S., and Kong, F. (2024b). Microalgae biotechnology: methods and applications. Bioengineering 11 (10), 965. doi:10.3390/bioengineering11100965
Watanabe, T., Shimada, R., Matsuyama, A., Yuasa, M., Sawamura, H., Yoshida, E., et al. (2013). Antitumor activity of the β-glucan paramylon from Euglena against preneoplastic colonic aberrant crypt foci in mice. Food Funct. 4 (11), 1685–1690. doi:10.1039/c3fo60256g
Weyman, P. D., Beeri, K., Lefebvre, S. C., Rivera, J., McCarthy, J. K., Heuberger, A. L., et al. (2015). Inactivation of Phaeodactylum tricornutum urease gene using transcription activator-like effector nuclease-based targeted mutagenesis. Plant Biotechnol. J. 13 (4), 460–470. doi:10.1111/pbi.12254
Wu, M., Qin, H., Deng, J., Liu, Y., Lei, A., Zhu, H., et al. (2021). A new pilot-scale fermentation mode enhances Euglena gracilis biomass and paramylon (β-1,3-glucan) production. J. Clean. Prod. 321, 128996. doi:10.1016/j.jclepro.2021.128996
Wu, S., Gu, W., Jia, S., Xie, X., Wang, L., and Wang, G. (2023b). Optimizing fucoxanthin production from Phaeodactylum tricornutum: impact of harvesting methods on culture medium reusability. Algal Res. 74, 103150. doi:10.1016/j.algal.2023.103150
Wu, Z., Yang, Y., Li, J., Bossier, P., Wei, X., Guo, Z., et al. (2023a). β-Glucans in particulate and solubilized forms elicit varied immunomodulatory and apoptosis effects in teleost macrophages in a dosedependent manner. Front. Immunol. 14, 1243358. doi:10.3389/fimmu.2023.1243358
Xie, S., Yue, Y., and Yang, F. (2024). Recent advances in CRISPR/Cas system-based biosensors for the detection of foodborne pathogenic microorganisms. Micromachines 15 (11), 1329. doi:10.3390/mi15111329
Xie, Y., Li, J., Qin, H., Wang, Q., Chen, Z., Liu, C., et al. (2021). Paramylon from Euglena gracilis prevents lipopolysaccharide-induced acute liver injury. Front. Immunol. 12, 797096. doi:10.3389/fimmu.2021.797096
Xu, Z., Hao, Y., Luo, A., and Jiang, Y. (2024). Technologies and applications in wireless biosensors for real-time health monitoring. Med-X 2 (1), 24. doi:10.1007/s44258-024-00041-3
Yamamoto, F. Y., Castillo, S., and Gatlin III, D. M. (2020). Immunomodulatory effects of β-glucans derived from Euglena gracilis or Saccharomyces cerevisiae for hybrid striped bass (Morone chrysops × M. saxatilis). Aquac. Res., 51(3), 1211–1221. doi:10.1111/are.14472
Yamamoto, F. Y., Sutili, F. J., Hume, M., and Gatlin, D. M. (2018). The effect of β-1,3-glucan derived from Euglena gracilis (Algamune(™)) on the innate immunological responses of Nile tilapia (Oreochromis niloticus L.). J. Fish. Dis. 41 (10), 1579–1588. doi:10.1111/jfd.12871
Yang, H., Choi, K., Kim, K. J., Park, S. Y., Jeon, J. Y., Kim, B. G., et al. (2022). Immunoenhancing effects of Euglena gracilis on a cyclophosphamide-induced immunosuppressive mouse model. J. Microbiol. Biotechnol. 32 (2), 228–237. doi:10.4014/jmb.2112.12035
Yasuda, K., Nakashima, A., Murata, A., Suzuki, K., and Adachi, T. (2020). Euglena gracilis and β-glucan paramylon induce Ca2+ signaling in intestinal tract epithelial, immune, and neural cells. Nutrients 12 (8), 2293. doi:10.3390/nu12082293
Yasuda, K., Ogushi, M., Nakashima, A., Nakano, Y., and Suzuki, K. (2018). Accelerated wound healing on the skin using a film dressing with β-glucan paramylon. Vivo 32 (4), 799–805. doi:10.21873/invivo.11310
Zhang, F., Li, Z., Duan, Y., Abbas, A., Mundaca-Uribe, R., Yin, L., et al. (2022). Gastrointestinal tract drug delivery using algae motors embedded in a degradable capsule. Sci. Robot. 7 (70), eabo4160. doi:10.1126/scirobotics.abo4160
Zhong, D., Zhang, D., Chen, W., He, J., Ren, C., Zhang, X., et al. (2021). Orally deliverable strategy based on microalgal biomass for intestinal disease treatment. Sci. Adv. 7 (48), eabi9265. doi:10.1126/sciadv.abi9265
Keywords: microalgae, health care, functional foods, β-glucan, drug delivery, synthetic biology
Citation: Li C, Du M, Han Y, Sun W, Chen Z, Liu Q, Zhu H, Zhao L, Li S and Wang J (2025) Microalgae in health care and functional foods: β-glucan applications, innovations in drug delivery and synthetic biology. Front. Pharmacol. 16:1557298. doi: 10.3389/fphar.2025.1557298
Received: 08 January 2025; Accepted: 11 February 2025;
Published: 04 March 2025.
Edited by:
Jinyao Li, Xinjiang University, ChinaReviewed by:
Blanca Hernandez-Ledesma, Spanish National Research Council (CSIC), SpainCopyright © 2025 Li, Du, Han, Sun, Chen, Liu, Zhu, Zhao, Li and Wang. This is an open-access article distributed under the terms of the Creative Commons Attribution License (CC BY). The use, distribution or reproduction in other forums is permitted, provided the original author(s) and the copyright owner(s) are credited and that the original publication in this journal is cited, in accordance with accepted academic practice. No use, distribution or reproduction is permitted which does not comply with these terms.
*Correspondence: Shuangfei Li, c2ZsaUBzenUuZWR1LmNu; Jiangxin Wang, anh3YW5nQHN6dS5lZHUuY24=
Disclaimer: All claims expressed in this article are solely those of the authors and do not necessarily represent those of their affiliated organizations, or those of the publisher, the editors and the reviewers. Any product that may be evaluated in this article or claim that may be made by its manufacturer is not guaranteed or endorsed by the publisher.
Research integrity at Frontiers
Learn more about the work of our research integrity team to safeguard the quality of each article we publish.