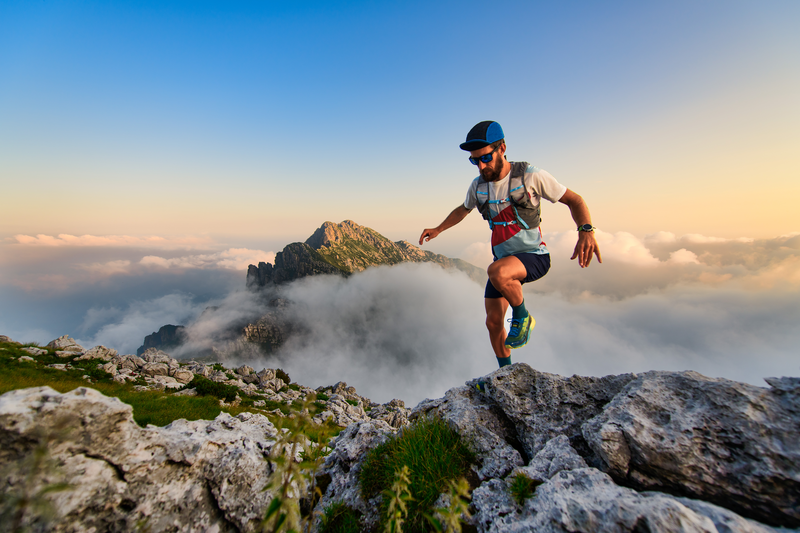
95% of researchers rate our articles as excellent or good
Learn more about the work of our research integrity team to safeguard the quality of each article we publish.
Find out more
REVIEW article
Front. Pharmacol. , 01 April 2025
Sec. Ethnopharmacology
Volume 16 - 2025 | https://doi.org/10.3389/fphar.2025.1557136
Typha angustifolia L. is a perennial marsh botanical drugs belonging to the genus Typha of the family Typhaceae, boasts a medicinal legacy spanning over 1900 years in China. Within traditional medicine, it is often used to treat a variety of bleeding disorders and gynecological diseases. Typha angustifolia contains various active components and metabolites including flavonoids, steroids, phenylpropanoids and organic acids. Over 94 compounds have been isolated and identified from T. angustifolia, demonstrating significant pharmacological activities such as anti-inflammatory, analgesic, anti-platelet aggregation, anti-atherosclerosis and anti-oxidation. In modern clinical practice, T. angustifolia is extensively utilized in treating dysmenorrhea, irregular menstruation, trauma bleeding, soft tissue contusion, hematochezia, hematuria and abnormal uterine bleeding. Typha angustifolia has a wide range of biological activities, making it a valuable resource for discovering potential drug candidates and developing new botanical supplements. This paper provides a comprehensive review of the research status of T. angustifolia, encompassing its botany, traditional uses, phytochemistry, pharmacological activity, and quality control, with the objective of enhancing our understanding of the application value and bioavailability of this traditional medicinal plant and offering a reference point for further research in this field.
Typha angustifolia L., a renowned traditional herbal medicine, is derived from a perennial marsh plant belonging to the genus Typha of the family Typhaceae. This plant thrives in warm, humid swamps and shallow waters and is widely distributed in central and northeastern China, as well as in northern Asia, Europe, and North America (State Administration of TCM of the PR China, 1999). In ancient China, the yellow male inflorescences on the top of T. angustifolia were harvested in summer, dried, rolled, and sifted into powder for medicinal use, which has a history of more than 1900 years. Traditional ethnic medicine attributes hemostatic and diuretic properties to T. angustifolia, which finds widespread use in treating many diseases such as hematemesis, hemoptysis, traumatic bleeding, dysmenorrhea, thoracic and abdominal pain, swelling, urinary retention, hematochezia, and hematuria (Chinese pharmacopoeia committee, 2020; Nanjing University of TCM, 2006).
Typha angustifolia contains abundant bioactive metabolites, with 92 chemical metabolites currently isolated and identified, including flavonoids, steroids, alkaloids, organic acids, and volatile oils. Among them, flavonoids are considered the most representative active metabolites. Numerous in vivo and in vitro studies have demonstrated the wide range of biological activities exhibited by these compounds, including anti-inflammatory, analgesic, anti-platelet aggregation, anti-atherosclerosis, and anti-oxidation. In modern clinical practice, T. angustifolia is utilized in the treatment of benign prostatic hyperplasia, hyperlipidemia, and fundus vascular lesions (Li and Xu, 2018; Qin et al., 2017; Zhu, 2002). It is noteworthy that, as a marsh plant, T. angustifolia also plays a role in improving environmental water quality (Grebenshchykova et al., 2020; Nabuyanda et al., 2019; Reyes et al., 2013). It has a beneficial purification effect on heavy metal pollution and eutrophication and holds certain application value in urban sewage treatment (Zheng, 2018).
Typha angustifolia possesses high medicinal value and a broad spectrum of biological activities, presenting abundant resources for the discovery of promising drug candidates and the development of new plant supplements. However, current research on the active metabolites and mechanisms of T. angustifolia is insufficient, with a lack of mechanism-based pharmacological activity evaluation and in vitro and in vivo studies. Additionally, a systematic and effective quality control system has not been established, leading to the underutilization of its resources. Therefore, this paper reviews the research on T. angustifolia from various perspectives, including botany, traditional uses, phytochemistry, pharmacological activity, and quality control, by summarizing and discussing the research status, application prospects, and limitations, aims to provide theoretical support and guidance for the comprehensive development and utilization of this important medicinal plant resource.
Following PRISMA guidelines for rigor and reproducibility, we conducted a systematic literature search with “T. angustifolia” as the central keyword, expanding it iteratively to include synonymous terms, related pharmacological applications, and therapeutic outcomes. Boolean operators were used to combine search strings focusing on both plant identity and research scope. We examined a wide range of academic sources from international databases such as PubMed, ScienceDirect, Web of Science, CNKI and Baidu Scholar, and supplemented these with manual searches of the Chinese Pharmacopoeia and historical texts. All publications in peer reviewed journals until December 2024 were considered.
Typha angustifolia is a perennial botanical drugs, typically reaching a height of 1.5–3 m. The rhizomes of the plant are milky yellow in color. The stem is erect and stout in the aboveground portion, gradually tapering as it ascends. The leaves are strap-shaped, measuring 40–70 cm in length and 0.4–0.9 cm in width. They possess a smooth and hairless surface, and the upper part of the leaves is flat, while the lower part’s abdomen is slightly concave. The back of the leaves gradually rises and protrudes outward, resulting in a semicircular transverse section, the base of the sheath expands and grows around the part of the stem. The inflorescences are typically spaced around 2.5–6.9 cm apart, with the male inflorescences measuring approximately 2.7–9.2 cm in length. The central axis of the inflorescences is white, curved, and covered with soft hairs. From the base of the inflorescences, there are 1-3 bracteoles slightly wider than the leaves. The lower part consists of the female flowers, measuring about 15–30 cm in length, with a bract also growing slightly wider than the leaf. The male flower is usually composed of three stamens, with anthers about 2 mm long and containing two chambers, and the overall shape is striped, with distinct nearly spherical pollen grains. The filaments are short, and the flower is united at the base into a short stalk, measuring about 2–3 mm in length. The stigma is spoon-shaped, curved outward, about 1.3–1.8 mm in length. The ovary is fusiform, exhibiting brown spots on the surface, and approximately 1 mm in length. The stalk at the root of the ovary is thinner, about 5 mm in length. The sterile female ovary is 1–1.2 mm long, conical in shape, with the uppermost portion yellowish brown and short-pointed, displaying undeveloped stigmas; the base of the ovary is frequently white, adorned with filamentous hairs that extend upward, slightly shorter than the stigma. The fruit type is a nut, the shape is oval, measuring approximately 1.5 mm in length. The pericarp has long brown spots, and the seeds are dark brown, about 1–1.2 mm in length. The florescence of T. angustifolia is from June to August, and the fruiting period is from August to September (Zhang, 1998; State Administration of TCM of the PR China, 1999; Nanjing University of TCM, 2006). The whole plant and inflorescence are shown in Figure 1.
Typha angustifolia, known as “Pu Huang” in Chinese, refers to an botanical drug renowned for its styptic and blood-stasis properties. Traditional Chinese Medicine extensively utilizes T. angustifolia in the treatment of dysmenorrhea, irregular menstruation, abdominal pain, trauma bleeding, soft tissue contusion, hematochezia, hematuria, and functional uterine bleeding (Nanjing University of TCM, 2006). Beyond its clinical applications, T. angustifolia also serves as a vital aquatic economic plant. Its leaf bases and rhizome apices are edible vegetables, while its stems and leaves are utilized for weaving mats and other crafts, as well as in paper manufacturing, the female flower can be employed as filling for pillow cores and cushions (Zhao and Xu, 2007).
The first documented of T. angustifolia was in the Shen Nong Ben Cao Jing, the earliest Chinese treatise on materia medica which over 1,900 years ago (Sun, 1963). It describes T. angustifolia as a Chinese herbal medicine known for its diuretic, analgesic, and hemostatic effects. In the Tang Dynasty, the herbal book Yao Xing Lun records that T. angustifolia had a prominent hemostatic effect and could be used to treat a variety of bleeding diseases, including abnormal uterine bleeding, nasal mucosal bleeding and hematuria (Zhen, 2006). In the Classic Materia Medica, Ri Hua Zi Ben Cao in the Song Dynasty, records that T. angustifolia can be used to treat epigastric pain, dysmenorrhea, irregular menstruation, uroschesisn and hematochezia (Ri, 2005). Ben Cao Gang Mu, a botanical monograph of the Ming Dynasty, records that T. angustifolia had a good analgesic effect, which could be used to relieve various types of pathologic pain, such as chest pain, heartache, stomachache and bellyache (Li, 1957). The same period’s Materia Medica Ben Cao Jing Shu also had a similar record, and it was believed that this analgesic effect of T. angustifolia might be related to its influence on the blood circulation system (Miao, 2011). The traditional uses of T. angustifolia is more detailed in the Materia Medica classics of the Qing Dynasty. It is recorded in Ben Cao Chong Yuan that T. angustifolia can be used to treat a variety of hemorrhagic diseases such as hematuria, traumatic bleeding and digestive tract bleeding (Zhang, 1983). Notably, T. angustifolia is effective in alleviating pain symptoms commonly associated with bleeding. Ben Cao Bei Yao was the first to suggest that the therapeutic effect of T. angustifolia could be enhanced through high-temperature roasting, and it also reported that T. angustifolia can be used to treat soft tissue contusion, menorrhagia, spermatogenesis and urinary retention (Wang, 2005). In addition, T. angustifolia also plays an important role in the treatment of some digestive diseases, such as flatulence, digestive dysfunction and enteritis. This is confirmed by the relevant records in Ben Cao Cong Xin and Ben Cao Bian Du (Wu, 2013; Zhang, 1936). The ancient Classic Medica books on T. angustifolia and their traditional uses are shown in Table 1.
Moreover, T. angustifolia is also a crucial component in over 10 listed traditional Chinese medicine prescription preparations, including Jinggu Dieda pills, Fuke Zhixue Ling, Mo Luo dan and Hexue Mingmu Pian. These formulations are utilized in treating conditions such as soft tissue contusion, functional uterine bleeding, chronic atrophic gastritis, and fundus vascular diseases (Li and Xu, 2018; Qi et al., 2022; Xue et al., 2023). All the formulated preparation of Traditional Chinese Medicine containing T. angustifolia and their clinical applications are shown in Table 2.
Table 2. Clinical application of formulated preparation of Traditional Chinese Medicine of Typha angustifolia.
Typha angustifolia contains a rich array of chemical metabolites, with 94 compounds having been isolated and identified thus far. These compounds include flavonoids (1–16), steroids (17–34), phenylpropanoids (35–43), Organic acid (44–57), volatile oil (58–79) and other compounds (80–94). Phytochemical studies indicate that the most active metabolites are found in the pollen of T. angustifolia, with flavonoids being the predominant constituent. The names, categories and corresponding structures of these compounds are summarized in Table 3 and Figures 2–9.
Flavonoid compounds are abundant in T. angustifolia, and are considered as the basis of many pharmacological activities such as anti-inflammation, analgesia and anti-hyperlipidemic. At present, a total of 16 flavonoids (1–16) have been isolated and identified from T. angustifolia, including Isorhamnetin (1), Kaempferol (2), Quercetin (3), Naringenin (4), Quercetin-3-O-rutinose (5), 3.3′-Dimethylquercetin (6), Cacticin (7), Isorhamnetin-3-O-(2G-α-rhamnosyl)-rutioside (8), Quercetin-3-O-(2G-α-L-rhamnosyl)-rutioside (9), Isorhamnetin-3-O-neohesperidin (10), Narcissin (11), Kaempferol-3-O-neohesperidoside (12), Quercetin-3-O-neohesperidoside (13), Picatechin (14), Catechin (15) and Typhaneoside (16). Moreover, the compounds sorhamnetin-3-O-neohesperidin (10) and Typhaneoside (16) have also been reported to have significant antioxidant activities and promote the proliferation of human umbilical vein endothelial cells in vitro (Chen et al., 2017). These compounds and their corresponding structures are shown in Table 3 and Figure 2.
A total of 18 steroid compounds (17–34) were isolated from T. angustifolia, most of which were cyclopentane-polyhydrophenanthrene as the structural framework. Monaco et al. (1990) isolated several novel sterols with ergostane skeleton from T. angustifolia, and the compounds 7β-hydroxysitosterol (26) and 7α-hydroxysitosterol (27) were isolated from this plant for the first time. These compounds are β-Sitosterol (17), Daucosterol (18), Sitosteryl palmitate (19), β-sitostenone (20), Stigmastane-3,6-dione (21), Daucosterol 6′-O-palmitate (22), Peroxyergosterol (23), Isofucosterol (24), 7-oxositosterol (25), 7β-hydroxysitosterol (26), 7α-hydroxysitosterol (27), Stigmastan-3-6-dione (28), Stigmast-4-en-6β-ol-3-one (29), Stigmast-4-en-6α-ol-3-one (30), 3β-hydroxystigmast-5-en-7-one (31), 6α-hydroxystigmast-4-en-3-one (32), Stigmast-5-ene-3β,7α-diol (33) and Stigmast-5- ene-3β,7β-diol (34). These compounds and their corresponding structures are shown in Table 3 and Figures 3, 4.
The phenylpropanoids in T. angustifolia are mainly divided into coumarins and lignin, which exhibit distinct structural characteristics. Coumarins are characterized by a benzene ring fused to an alpha-pyrone ring, while lignin compounds typically consist of phenylpropane units linked by various types of bonds, forming a complex polymer structure. At present, nine compounds (35–43) have been isolated, which are respectively aulownin (35), 9-n-butyl-guaiacylglycerol (36), 9-n-butyl-isoguaiacylglycerol (37), 1-O-p-coumaroylglycerol (38), 1-O-di-p-coumaroylglycerol (39), Syringaresinol (40), Pinoresinol (41), Threo-2,3-bis-(4-hydroxy-3-methoxyphenyl)-3-methoxypropanol (42) and Erythro-2,3-bis(4-hydroxy-3-methoxyphenyl)-3-butoxypro pan-1-ol (43). These compounds and their corresponding structures are shown in Table 3 and Figure 5.
At present, fourteen organic acid compounds (44–57) were isolated from T. angustifolia, predominantly consisting of unsaturated organic acids and long-chain fatty acids. These compounds are Palmitic Acid (44), Cerotinic acid (45), Octadecanoic acid (46), Vanillic acid (47), 4-Hydroxycinnamic acid (48), Ferulic acid (49), 4-Acetoxy-3-methoxycinnamic acid (50), Lauric acid (51), Typhic acid (52), Arachidonic acid (53), Pyruvic Acid (54), Citric Acid (55), Nicotinic acid (56) and Protocatechuic acid (57). These compounds, such as Ferulic acid (49) and Citric acid (55), have shown promising anti-oxidant and anti-inflammatory properties in recent studies, and also contribute to the diverse chemical composition of T. angustifolia, offering potential pharmaceutical applications in various health conditions (Liu et al., 2022; Wu et al., 2019). These compounds and their corresponding structures are shown in Table 3 and Figure 6.
The volatile oil content in T. angustifolia is relatively abundant. Currently, 23 compounds were isolated and identified from T. angustifolia, including Phenethyl senecioate (58), Tetrahydro-2-(12-pentadecynyloxy)-2H-pyran (59), Methyl benzoate (60), Methyl hydrocinnamate (61), 2, 4-di-tert-butylphenol (62), Methyl vanillate (63), Methyl hexadecanoate (64), 1,1′-bicyclohexy (65), Dimethyl adipate (66), Octanoic acid (67), Dimethyl azelate (68), Dimethyl sebacate (69), Dimethyl undecanedioate (70), Methyl 9,10-dihydroxyoctadecanoate (71), Methyl vaccinate (72), 10-Octadecenoic acid (73), bis(2-ethylhexyl) phthalate (74), Diethyl phthalate (75), Dibutyl phthalate (76), Diisobutyl phthalate (77), Dimethyl phthalate (78) and Phenyl phthalate (79). The volatile oil metabolite is crucial for evaluating T. angustifolia quality. Biological activity studies indicate that many of the aforementioned metabolites exhibit strong biological activity. For example, Dimethyl phthalate (78) is commonly used in mosquito repellent production due to its exceptional adhesion and water resistance (Li et al., 2006). These compounds and their corresponding structures are shown in Table 3 and Figure 7.
Tao et al. (2010) first reported two new cerebrosides in T. angustifolia, 1-O-(β-D-glucopyranosyloxy)-(2S,3S,4R, 8Z)-2-[(2′R)-2′-ydroxytricosanoylamino]-8-nonadecene-3,4-diol (80) and 1-O-(β-D-glucopyranosyloxy)-(2S,3R,4E, 8Z)-2-[(2′R)-2′-hydroxynonadecanoylamino]-4.13 -nonadecene-3-diol (81), and found that they could inhibited vascular smooth muscle cell proliferation in a dose-dependent manner. Additionally, T. angustifolia also contains some aliphatic hydrocarbons and alkaloids, such as 6,21-Nonacosanediol (82), 6,10-Nonacosanediol (83), 6,8-Nonacosanediol (84), 1-Hexacosanol (85), Hexadecanol (86), Zarzissine (87), Choline (88), Allantoin (89), Adenine (90), Hypoxanthine (91), Uracil (92), 2.4(3H, 5H)-Pyrimidinedione (93), 2,3- Dihydroxypropyl hexadecanoate (94). These compounds and their corresponding structures are shown in Table 3 and Figure 8.
Given its extensive utilization in ethnopharmacological contexts, T. angustifolia has garnered considerable attention from researchers. Over recent decades, investigations into the pharmacological activities of T. angustifolia have progressively unveiled its diverse therapeutic potentials, including but not limited to anti-inflammatory, analgesic, anti-platelet aggregation, anti-atherosclerosis and anti-oxidative activities. Researchers have also carried out some studies based on different in vivo and in vitro biological models to confirm these pharmacological activities. Nonetheless, it is imperative to note that these studies have not elucidated the relationship between traditional medicinal usage and pharmacological efficacy comprehensively. Moreover, the association between bioactive metabolites and in vivo mechanisms remains ambiguous, with scant attention paid to pharmacokinetic parameters and bioavailability. Detailed pharmacological activities and biological studies are shown in Table 4 and Figures 9, 10.
The total flavonoids of T. angustifolia exhibit inhibitory effects on the expression of IL-6 mRNA and the secretion of protein in C2C12 skeletal muscle cells cultured with palmitic acid. This inhibition contributes to the alleviation of skeletal muscle inflammation and the amelioration of insulin resistance within the skeletal muscle, implying a potential mechanism linked to the regulation of glucose transport efficiency and the suppression of the NF-κB pathway (Lou et al., 2008b). Further investigations into the anti-inflammatory properties of T. angustifolia have revealed that its total flavonoids can diminish the levels of IL-6 and TNF-α in the serum of type 2 diabetic rats induced by a high-fat diet and low-dose Streptozocin (Feng et al., 2014a). Additionally, they downregulate the content of SOCS-3 by modulating the JAK/STAT pathway, thus mitigating chronic inflammation within the circulatory system. Typha angustifolia also exhibited an inhibitory effect on the PI3K/AKT/mTOR pathway in vitro. This effect is significant as it reduces inflammation by inducing macrophage autophagy and inhibiting the secretion of the inflammatory factor IL-10 (Wang D. et al., 2017).
Typha angustifolia can inhibit the damage of vascular endothelial cells induced by Ox-LDL, reduce the intercellular adhesion and inhibit the further occurrence of inflammatory reaction by down-regulating the expression of IL-8 mRNA (Wang and Li, 2012). Liu et al. (2021) reported that T. angustifolia can suppress the inflammatory response by inhibiting the expression of PARP1 and MAPK, consequently reducing lymphocyte infiltration and enhancing motor function in an acute spinal cord injury rat model. Zhang et al. (2013) used the combination of immunogenic bovine serum albumin, CCl4 and LPS to induce a rat model of nephritis hematuria, and found that T. angustifolia could significantly reduce the number of malformed red blood cells in the urine of rats, the contents of urinary protein and creatinine, and the value of serum urea nitrogen. It can increase the Endogenous creatinine clearance rate of serum total protein and endogenous creatinine clearance rate, and has a certain protective effect on renal function. Another study also reported that T. angustifolia can effectively inhibit the expression of iNOS and COX-2 in LPS-induced RAW264.7 cells, and then downregulate the content levels of inflammatory factors IL-1β, IL-6 and TNF-α (Chen et al., 2021). Analysis of the active metabolites within T. angustifolia extracts indicated that organic acids and flavonoids may constitute its primary anti-inflammatory substance foundation. It is worth mentioning that T. angustifolia can also protect the blood-retinal barrier function by inhibiting pro-inflammatory cytokines such as TNF-α and ICAM-1. This action reduces the excessive release of NO, thereby alleviating retinal inflammation and exerting a protective effect on retinal microangiopathy caused by diabetes (Yang et al., 2021).
Researchers observed the analgesic effect of T. angustifolia by plate method and writhing body method. They found that T. angustifolia significantly prolonged the number of foot lickings and pain latency in mice, exerting a notable inhibitory effect on pain induced by chemical and physical stimuli. Interestingly, the analgesic effect of T. angustifolia surpassed that of diclofenac sodium in the positive drug group (Ge et al., 2002). Zhang et al. (2017)’s study also confirmed this point, and further explored the effect of T. angustifolia on the regulation of pain perception in rats by using pressure plate pain measurement method, and found that the analgesic activity of T. angustifolia was dose-dependent, and based on the solubility of active metabolites in different organic solvents, it was speculated that flavonoids might be the main active metabolites of T. angustifolia for analgesia. In addition, T. angustifolia also exhibited an inhibitory effect on inflammatory toe pain induced by exogenous substances, significantly prolonging the paw retraction latency in inflamed rats (Fu et al., 2017). In a study utilizing an LC-MS combined effect-directed fractionation strategy, two active metabolites, Isorhamnetin-3-O-neohesperidin (10) and Typhaneoside (16), were isolated and identified from T. angustifolia. Both compounds demonstrated significant reductions in the number of body twists induced by acetic acid in mice, with inhibition rates of 54% and 51.8%, respectively (Zeng et al., 2019).
Typha angustifolia can inhibits platelet aggregation induced by ADP, arachidonic acid, collagen, and other inducers in vitro, slowing down the aggregation rate and depolymerizing the irreversible platelet aggregation, and this inhibitory effect is dose-dependent (Feng and Liu, 1999). Typha angustifolia can reduce the prothrombin time, thrombin time, activated partial thromboplastin time, and serum fibrinogen content in rats (Ma et al., 2010). Additionally, further animal experiments revealed that T. angustifolia inhibits arteriovenous anastomosis thrombosis formation in rats, decreases the rate of arterial thromboembolism induced by electrical stimulation, and exhibits a certain protective effect on endothelial cells (Zhao and Zhu, 2011). One study observed the effects of T. angustifolia on hemorheology indexes in rats and found that it could significantly reduce whole blood viscosity, enhance red blood cell deformations, and decrease aggregation without significantly affecting hematocrit in each dose group, indicating an improvement in blood circulation (Kong et al., 2011). Yang and Li (2012) reported similar research results, further confirming that the total flavonoids of T. angustifolia are active metabolites against platelet aggregation. These flavonoids improved the elevation of rabbit whole blood viscosity induced by a combination of adrenaline and ice bath, reduced the maximum platelet aggregation rate, and shortened the maximum agglutination time.
In addition to its influence on hemorheology, T. angustifolia can also reduce the content of TXB2 and 6-keto-PGF1α in the plasma of blood stasis model rats, thus improving the platelet aggregation rate and inhibiting thrombosis (Shen et al., 2015). He et al. (2014) reported that T. angustifolia significantly inhibits the formation of thromboxemia in rats and has a protective effect on vascular endothelial cells. Building on this foundation, the anti-platelet aggregation activities of different organic solvent extracts of T. angustifolia were systematically screened, leading to the preliminary confirmation that the active metabolites are enriched in the ethyl acetate extract of T. angustifolia. Furthermore, various analysis methods based on network construction and genomics have also been employed to study the anti-platelet aggregation activity of T. angustifolia. For instance, Wang X. et al. (2017) evaluated T. angustifolia‘s anti-platelet aggregation activity using a high-throughput platelet aggregation experimental system in vitro. They discovered that T. angustifolia could decrease the level of calcium ions in platelets, potentially inhibiting platelet aggregation by activating the PI3K/Akt/eNOS pathway.
Typha angustifolia effectively reduces the formation and development of atherosclerotic plaques and diminishes the lesion area through various mechanisms, including the reduction of blood lipid levels, lipid accumulation, and promotion of endothelial cell proliferation. Its anti-atherosclerotic activity primarily involved lipid reduction and endothelial cell protection.
Typha angustifolia can promote the synthesis of prostacyclin (PGI2), reduce the production of lipid peroxides by vascular endothelial cells, reduce the levels of serum TC, LDL-C and TC/HDL-C by inhibiting intestinal absorption of exogenous cholesterol, and inhibit the increase of TXB2 content (Qiang et al., 1987; Zhang et al., 1987). In a variety of animal models, T. angustifolia showed significant activity in lowering blood lipids. Tao and Li (2004) reported that T. angustifolia could reduce the levels of total cholesterol, triglycerides, and low-density lipoprotein in the serum of a hyperlipidemia rabbit model. While the levels of TC and TG in the plasma of the hyperlipidemia quail model decreased, the levels of endothelin and nitric oxide were not significantly affected. By enhancing the function of macrophages, T. angustifolia could reduce the hyperlipidemia induced by high-fat diet, but had no effect on the elevation of ALT caused by high-fat (Reng et al., 1989; Zhou et al., 2006). Jiang and Huang (2009) induced hyperlipidemia model in rats by intraperitoneal injection of vitamin D and high-fat diet, and found that T. angustifolia could significantly reduce the content levels of TC, TG, LDL-C and NO in the serum of rats. By down-regulating the expression of PERK-CHOP pathway, T. angustifolia also can inhibit endoplasmic reticulum stress and reduce cell apoptosis of aortic endothelial cells in atherosclerotic mice, and reduce the average area of atherosclerotic lesions (Mo, 2019).
Additionally, it has also been observed that T. angustifolia can prevent excessive accumulation of intracellular cholesterol and regulate the balance of total cholesterol in the body by up-regulating the expression of density lipoprotein receptor mRNA (Jiang et al., 2008). An in vitro study reported that total flavonoids of T. angustifolia can reduce the secretion of free fatty acids in 3T3-L1 adipocytes and regulate the lipid metabolism of the cells (He et al., 2006a). Further studies found that the lipid-lowering mechanism of bulrushes involved down-regulating the expression of major transcription factors in the fat formation pathway, which could inhibit the transport, uptake, and synthesis of lipids by reducing the levels of lipid metabolizing enzymes FABP4, LPL and FAS (He et al., 2008). Moreover, Typhaneoside (16), one of the active metabolites of T. angustifolia, can dose-dependently improve non-alcoholic fatty liver disease induced by a high-fat diet in mice. This improvement occurs through the activation of FXR expression, leading to a reduction in lipid accumulation in the liver and white adipose tissue. This demonstrates the multifaceted lipid-lowering properties of T. angustifolia and its active metabolites, presenting potential therapeutic avenues for combating dyslipidemia-related conditions (Zheng et al., 2023).
Typha angustifolia has demonstrated a significant protective effect on endothelial cells in various in vivo animal experimental models. It enhances prostaglandin synthesis in arterial endothelial cells of spontaneously hypertensive rats, reduces the concentration of intracellular free calcium ions, improves transport efficiency, and preserves endothelial cell function, thus significantly preventing the formation of atherosclerotic plaques (Qiang et al., 1987). Zhou et al. (2006) reported similar findings, indicating that T. angustifolia could notably inhibit the formation of atherosclerotic lesions in dyslipidemic quail. Additionally, it also inhibits the migration of macrophages and fibroblasts into the subintima, reduces the formation of lipostriated arteriosclerosis plaques in rabbit atherosclerotic models, and mitigates risk factors associated with endothelial cell injury (Tao and Li, 2004).
Typha angustifolia can significantly reduce the plasma vWF content, increase the plasma NO content, enhance the endothelum-dependent relaxation response induced by acetylcholine and norepinephrine induced contraction response of thoracic aorta. It has obvious protective effect on vascular endothelial injury caused by hyperlipidemia. This underscores its pronounced protective effect against vascular endothelial injury caused by hyperlipidemia (Zhang et al., 2003). Additionally, T. angustifolia inhibits the upregulated expression of ICAM-1 and MCP-1 in human umbilical vein endothelial cells induced by oxidized low-density lipoprotein, leading to reduced LDH release, enhanced endothelial cell proliferation, and protection against inflammation-induced vascular endothelial injury (Wang and Huang, 2010). Further investigation into its active compounds revealed that total flavonoids are among the primary active substances of T. angustifolia. These flavonoids exhibit protective effects against endothelial cell damage induced by hypoxia, improving the activity of human umbilical vein endothelial cells under hypoxic conditions. Moreover, they inhibit the production of the endogenous vasoactive factor, human endothelin-1 (ET-1), and regulate the balance of NO/ET-1 (Lin et al., 2011). By activating the autophagy pathway of macrophages, total flavonoids of T. angustifolia can reduce the degradation of extracellular matrix and inhibit the formation and development of plaque, suggesting that the mechanism may be related to the inhibition of the activation of PI3K/AKT/mTOR pathway (Wang D. et al., 2017).
Typha angustifolia can enhance the glucose uptake and transport capacity of 3T3-L1 adipocytes in a dose-dependent manner (He et al., 2006b). Lou et al. (2008a) reported that T. angustifolia improves palmitic acid-induced insulin resistance in C2C12 skeletal muscle cells. It increases cellular glucose utilization efficiency and uptake capacity by upregulating glucose transporter (GLUT-4) expression. It can also upregulate the expression of Scr protein and promote the formation of its signal complex, and increase the glucose consumption level of skeletal muscle cells (Liu et al., 2014). Feng et al. (2012a) reported similar results, and further confirmed in vitro experiments that total flavonoids of T. angustifolia can improve skeletal muscle insulin resistance by increasing the expression of β-arrestin-2 protein in cells. Fu et al. (2010) reported that total flavonoids from T. angustifolia can enhance the expression of PPARγ and C/EBP-α by promoting the proliferation and differentiation of adipocytes, thus antagonizing the insulin resistance induced by fatty acids. Further studies showed that T. angustifolia can also improve fatty acid-induced islet beta cell damage, which may be related to the reduction of endoplasmic reticulum stress and the reduction of production of oxidative stress products (Feng et al., 2015).
Wang et al. (2003) conducted an investigation using a rat model of cerebral ischemia-reperfusion injury to assess the protective effects of T. angustifolia on brain tissue. The findings revealed that T. angustifolia significantly elevated the activities of LDH and SOD in brain tissue while inhibiting lipid peroxidation damage. Similarly, Jiang and Huang (2009) also corroborated these results in a rat model of atherosclerosis, demonstrating the antioxidant activity of T. angustifolia. This activity notably reduced the content of MDA in rat serum and suppressed the production of oxygen free radicals within the non-enzymatic system. Chen et al. (2006) reported that co-culturing T. angustifolia with rat nerve cells markedly boosted the activities of GSH-Px and SOD within the cells. This led to a reduction in MDA content and mitigated brain tissue lipid peroxidation damage induced by mercury, and it also significantly enhanced the antioxidant capacity of nerve cells following mercury injury. Wang and Li (2010) confirmed through in vitro experiments using cultured human umbilical vein endothelial cells that T. angustifolia can decrease LDH and MDA activities, increase SOD activity, and rectify endothelial cell dysfunction induced by oxidized low-density lipoprotein. Furthermore, a comprehensive study evaluated the antioxidant activity of various extracts of T. angustifolia. It was found that both ethanol extract and water extract had significant free radical scavenging ability and antioxidant activity, which could significantly reduce the content of MDA and NO in endothelial cells under oxidative stress, and increase the content of SOD (Chen et al., 2017).
Qin et al. (2017) reported that T. angustifolia can inhibit prostatic hyperplasia induced by testosterone propionate and reduce serum testosterone levels. In a mouse model of prostatic hyperplasia, Zhu et al. (2018) confirmed that T. angustifolia reduces the expression of Ki67 and CD34 antigens in prostate tissue, inhibiting pathological hyperplasia of prostate cells and reducing the aggregation of vascular endothelial cells. Typha angustifolia can enhance muscle tension, improve contractility, and prolong the contractility of isolated uterine smooth muscle in late pregnancy rats. This effect can be inhibited by prostaglandin synthetase inhibitors and calcium channel blockers, suggesting that the effect of T. angustifolia on isolated uterine smooth muscle involves various regulatory pathways (Lai et al., 2016). Additionally, T. angustifolia significantly inhibits the calcification of human vascular smooth muscle cells induced by ouabain. Its mechanism involves the inhibition of the NKA-α1 subunit and the blocking of the osteopontin signaling pathway (Lou et al., 2017).
The water extract of T. angustifolia inhibits the growth of Lewis lung cancer tumors in mice, increases serum levels of IL-2 and TNF-α, arrests tumor cells in the G1 phase, and induces tumor cell apoptosis (Chen et al., 2008). Additionally, tetrazole salt assay results demonstrate that T. angustifolia improves spleen lymphocyte proliferation in tumor-bearing mice (Li et al., 2011). Typha angustifolia exhibits a protective effect on retinal tissue lesions caused by hyperglycemia by down-regulating the expression of VEGF and Ang-1 and improving fasting blood glucose levels (Yang et al., 2021). Several studies have reported that T. angustifolia also mitigates brain tissue injury caused by ischemic reperfusion, protecting neuronal function through the regulation of proteins such as HSP-70, Caspase-3, Parkin, and Cyclin-E (Xia et al., 2008; Xia et al., 2009; Yuan and Xia, 2014). This inhibition of neuronal apoptosis and impact on mitochondrial autophagy suggest potential treatment avenues for ischemic encephalopathy and the development of novel therapeutics.
In the 2020 edition of the Chinese Pharmacopoeia, the quality evaluation method for Typha purpurea was standardized, with isorhamnetin-3-O-neohesperidin and T. angustifolia serving as the index metabolites for determining its content. However, the current quality evaluation methods lack comprehensiveness, relying solely on two chemical metabolites as quality evaluation indices weakens the correlation with drug activity. Furthermore, specific identification methods and quantitative standards are lacking, it is necessary to further establish a scientific and reasonable quality evaluation model based on modern technology to guide the production, circulation, and use of T. angustifolia more effectively.
At present, HPLC, HPCE, LC-MS/MS, GC-MS and other high-efficiency chromatography-mass spectrometry tools are often used in the quality control research of T. angustifolia for qualitative and quantitative analysis of active metabolites. Wen et al. (2006) established the HPLC method for the determination of total flavonoids content in T. angustifolia for the first time, and ensured a good linear relationship between its index metabolites, with good repeatability and high recovery rate. Ma et al. (2014) optimized the extraction process of total flavonoids from T. angustifolia, elution and separation of total flavonoids with macroporous resin, and further established a stable and simple purification process. Zhang et al. (2019) established a method for simultaneous determination of Isorhamnetin-3-O-neohesperidin (10) and Typhaneoside (16) in T. angustifolia. Compared with the traditional method, the peak time is shorter and the two metabolites can be effectively separated. Ge and Jia (2011) established an LC-MS/PAD method for the detection of Auramine O in T. angustifolia, which can effectively distinguish whether the botanical drugs have been illegally stained.
Fingerprints, multivariate statistical analysis and pharmacokinetics of traditional Chinese medicine are also widely used in the process of identification and separation of index metabolites. For the first time, Lu et al. (2018) tested the content of index metabolites in T. angustifolia at different mature stages, and the results showed that the active metabolites were the highest in T. angustifolia picked in the half flowering stage. Based on the UPLC method, Tang et al. (2022) established the traditional Chinese medicine fingerprint of T. angustifolia and the content determination method of eight main metabolites. A total of 15 common peaks were identified in the UPLC fingerprint, and the similarity of different batches of medicinal materials was greater than 0.900. Li et al. (2015) simultaneously determined the total content of five main index metabolites in 16 batches of T. angustifolia decoction pieces by HPLC, and established a quality characterization and correlation analysis method based on this.
A variety of emerging technologies play an important role in the quality control research of T. angustifolia and have made great progress in recent years, such as in vitro and in vivo quantitative studies through the combination of multiple chromatographic technologies, analysis of the enrichment mode of active substances based on metabolomics, and prediction of active substances through the establishment of network pharmacological models. In order to establish a scientific and sound quality evaluation system, we still need to conduct in-depth analysis of chemical information and pharmacodynamic results with statistical differences on the basis of existing research, and find chemical substances with high correlation with pharmacological activity to ensure the safety and effectiveness of clinical use.
Typha angustifolia has a long history of application and is widely used the treatment of dysmenorrhea, irregular menstruation, abdominal pain, trauma bleeding, soft tissue contusion, hematochezia, hematuria, and other hemorrhagic diseases. It also serves as a resource-rich aquatic cash crop, finding extensive applications in medicine, weaving, papermaking, and the food industry. With its high medicinal value, economic potential, and bioavailability, it holds significant importance. Currently, we have reviewed the botany, traditional uses, phytochemistry, pharmacological activities, and quality control of T. angustifolia. The paper then delves into future research directions and focal points, considering the following aspects:
Numerous studies have confirmed that flavonoids serve as the foundation for many pharmacological activities, including anti-inflammatory, analgesic, and lipid-lowering effects. Given their promising biological activity, the quest for novel flavonoid compounds from T. angustifolia and exploring the correlation between their structural characteristics and efficacy represent significant research endeavors and future focuses. However, current studies primarily focus on discovering and isolating new active metabolites, often remaining at the stage of crude extract analysis. Reports on potential biological activities of new compounds are scarce. In addition, it is essential that future studies address current methodological limitations by adopting standardized bioassay protocols to rigorously validate the pharmacological efficacy of these compounds. Thus, conducting bioactivity-driven structure-activity studies on newly isolated compounds is crucial. This approach can elucidate the internal relationship between active metabolites and pharmacological activities, guiding future research directions on T. angustifolia‘s active metabolites, and advancing the research and development of innovative drugs.
Systematic research into the pharmacological activity of T. angustifolia is crucial for unlocking its medicinal potential. This medicinal plant exhibits various pharmacological activities, including anti-inflammatory, analgesic, anti-platelet aggregation, anti-atherosclerosis, and anti-oxidation properties. However, existing studies have not delved deeply into the specific action links and mechanisms of T. angustifolia in vivo, nor have they thoroughly explored its relationship with disease targets. The focus of traditional plant research is the rapid and accurate discovery of pharmacological effector substances. However, the current study of the pharmacological active metabolites of T. angustifolia primarily revolves around extracts, particularly total flavonoid extracts, with a notable absence of in-depth exploration into monomer compounds. Moreover, future studies should critically address these limitations by integrating advanced in vivo models and mechanistic analyses to validate pharmacological effects and elucidate disease target interactions. Therefore, it is necessary to further study the correlation between the pharmacological activities and chemical metabolites of T. angustifolia by combining related techniques such as pharmacokinetics and network pharmacology. This will lead to a more comprehensive understanding of T. angustifolia‘s medicinal properties and facilitate the development of novel therapeutic interventions.
Typha angustifolia has traditionally been utilized in treating hemorrhagic diseases, particularly those stemming from local thrombosis and impaired blood microcirculation. This is maybe attributed to its anti-platelet aggregation activity, indicating potential for development as a novel antithrombotic drug. However, existing studies predominantly focus on T. angustifolia‘s effect on blood coagulation-related indices, with limited exploration into its specific mechanism influencing blood coagulation function. Future studies should critically evaluate these mechanisms using comprehensive in vitro and in vivo models to rigorously validate its antithrombotic potential. This underscores the need for robust clinical research validation and evidence to support traditional uses of T. angustifolia. Hence, we need to pay attention to the role of traditional Chinese medicine theory in guiding the use and development of drugs. In order to better develop and utilize the medicinal resources of T. angustifolia, it is necessary to establish a close relationship with traditional medical knowledge, and further strengthen the existing pharmacological activity research on this basis.
The quality control index of T. angustifolia is still relatively simple, the identification of active metabolites is blind, and there is no comprehensive quality evaluation system. In the future research, based on the existing active metabolite data, combined with a variety of emerging technologies, in-depth analysis of chemical information and pharmacodynamics results with statistical differences should be carried out, further digging related quality markers and improving the quality evaluation system of T. angustifolia. In assessing the quality of medicinal materials comprehensively, it's essential to emphasize clinical relevance in terms of safety and effectiveness. Establishing an overall quality evaluation method should prioritize active metabolite content. Notably, T. angustifolia contains numerous volatile metabolites, which may lose their activity due to external factors like light, humidity, temperature, and oxidation. Hence, this requires us to further develop quality control methods that can monitor the production and content changes of extracts over time based on the fingerprint of existing compounds or fractions, so as to improve the stability of the quality of medicinal materials.
In conclusion, T. angustifolia is a traditional medicinal plant with considerable development potential and research value. It is our hope that this paper can serve as a valuable reference for all researchers interested in T. angustifolia.
JL: Conceptualization, Investigation, Visualization, Writing – original draft, Writing – review and editing. ZC: Investigation, Software, Writing – original draft, Writing – review and editing. ST: Conceptualization, Methodology, Writing – review and editing. XS: Conceptualization, Formal Analysis, Investigation, Supervision, Validation, Writing – original draft. BS: Formal Analysis, Project administration, Validation, Writing – review and editing. XZ: Data curation, Funding acquisition, Resources, Writing – review and editing. YY: Data curation, Funding acquisition, Methodology, Resources, Writing – review and editing. XW: Data curation, Formal Analysis, Funding acquisition, Project administration, Resources, Supervision, Validation, Writing – original draft, Writing – review and editing.
The author(s) declare that financial support was received for the research and/or publication of this article. This work was supported by the National Natural Science Foundation of China (No. 82074327).
We thank the Science and Innovation Center of the College of Basic Medicine at Chengdu University of Traditional Chinese Medicine for their technical support.
The authors declare that the research was conducted in the absence of any commercial or financial relationships that could be construed as a potential conflict of interest.
The author(s) declare that no Generative AI was used in the creation of this manuscript.
All claims expressed in this article are solely those of the authors and do not necessarily represent those of their affiliated organizations, or those of the publisher, the editors and the reviewers. Any product that may be evaluated in this article, or claim that may be made by its manufacturer, is not guaranteed or endorsed by the publisher.
AKT, protein kinase B; ALT, glutamic-pyruvic transaminase; Ang-1, Angiopoietins-2; APTT, activated partial thromboplastin time; Caspase-3, cysteine protease protein-3; CHOP, CCAAT/enhancer binding protein homologous protein; COX-2, cyclooxygenase-2; ET-1, endothelin-1; FABP4, fatty acid-binding protein; FAS, fatty acid synthase; FIB, fibrinogen; FXR, farnesoid X receptor; GSH-Px, glutathione peroxidase; ICAM-1, intercellular cell adhesion molecule-1; JAK, Janus Kinase; LDH, lactate dehydrogenase; LDL-C, low-density lipoprotein cholesterol; LPL, lipoprotein lipase; MAPK, mitogen-activated protein kinase; MCP-1, monocyte chemoattractant protein-1; MDA, malonaldehyde; mTOR, mammalian target of rapamycin; PARP1, Poly ADP-ribose polymerase-1; PERK, protein kinase R-like endoplasmic reticulum kinase; PGI2, prostaglandin-2; PI3K, phosphatidylin-ositol-3-kinase; PT, prothrombin time; STAT, signal transducerand activator of transcription; TC, total cholesterol; TG, triglyceride; TT, thrombin clotting time; TXB2, thromboxane; VEGF, vascular endothelial growth factor; vW, von Willebrand factor.
Chen, C., Miu, J., Gu, Q., and Zheng, W. (2006). Effect of Typha angustifolia L. extract on the antioxidant capacity of primary cultured nerve cells in mercury-damaged SD rats. Pharm. J. Chin. PLA. 22, 3. doi:10.3969/j.issn.1008-9926.2006.05.001
Chen, C., Miu, J., Li, J., and Zheng, W. (2008a). Inhibition of Typha angustifolia L. aqueous extract on Lewis lung cancer in mice. Pharm. J. Chin. PLA. 24, 4. doi:10.279/CNKI:SUN:JFJN.0.2008-03-003
Chen, J., Hao, W., Feng, X., Song, K., Zhang, T., and Liu, C. (2019). Prediction and analysis of chemical constituents, pharmacological action and Q-marker of Typha angustifolia. Chin. Tradit. Herb. Drugs 50, 12–19. doi:10.7501/j.cnki.0253-2670.2019.9.446
Chen, P., Cao, Y., Bao, B., Zhang, L., and Ding, A. (2017). Antioxidant capacity of Typha angustifolia L. extracts and two active flavonoids. Pharm. Biol. 55, 1283–1288. doi:10.1080/13880209.2017.1300818
Chen, P., Yan, H., and Zhang, L. (2008). Study on chemical composition of Typha angustifolia. Strait. Pharm. J. 20, 64–66. doi:10.1156/j.cnki.163-9043.2016.02.17
Chen, T., Chen, C., Huang, Y., Baskaran, R., Tsai, J. J. P., and Hu, R. (2021). Ethanolic extract of Typha angustifolia L. modulates lipopolysaccharide-induced inflammatory response through inducible nitric oxide synthase/cyclooxygenase-2 signaling in RAW 264.7 macrophage. J. Tradit. Chin. Med. 41, 836–844. doi:10.19852/j.cnki.jtcm.2021.06.002
Chen, Y. (1990). The active ingredient from Typha angustifolia L. pollen. Chin. Tradit. Herb. Drugs 21, 2–5. doi:10.7501/j.issn.0253-2670.1990.2.017
Chen, Y., Li, F., and Tao, W. (2015). The natural chemical constituents of Typha angustifolia. Nat. Prod. Res. Dev. 27, 9–13. doi:10.16333/j.1001-6880.2015.09.009
Chinese Pharmacopoeia Committee (2020). Pharmacopoeia of the people’s Republic of China (volume I). Beijing: China Pharmaceutical Science and Technology Press.
Feng, X., Cao, S., Ji, S., and Zhou, Y. (2014a). Study on chemical constituents of analgesic active parts of Typha angustifolia L. (Part 2). Chin. Arch. Tradit. Chin. Med. 32, 3–6. doi:10.7885/j.cnki.10.0.2014-05-089
Feng, X., Chen, Q., and Liang, X. (2014). Effects of total flavonoids from Typha angustifolia L. on inflammatory factors and insulin sensitivity in type 2 diabetic rats. J. Guangzhou Univ. Tradit. Chin. Med. 31, 936–943. doi:10.13359/j.cnki.gzxbtcm.2014.06.019
Feng, X., and Liu, F. (1999). Effects of Typha angustifolia organic acids on rabbit platelet aggregation. J. Chin. Nat. 6, 48–49. doi:10.19621/j.cnki.11-3555/r.1999.06.082
Feng, X., Liu, J., and Liang, N. (2015). The intervention effect of total flavonoids from Typha angustifolia L. on palmitic acid-induced damage to INS-1 pancreatic islet β cells. J. Guangzhou Univ. Chin. Med. 32, 899–902. doi:10.13359/j.cnki.gzxbtcm.2015.05.026
Feng, X., Liu, Y., Wang, T., Chen, Y., Chen, W., Ying, J., et al. (2012a). Effects of total flavonoids from Typha angustifolia L. on the expression of β-arrestin-2 gene and protein in C2C12 skeletal muscle cells. Chin. J. Tradit. Chin. Med. Pharm. 27, 4. doi:10.1170/j.cnki.10.2012-09-017
Feng, X., Zeng, G., Tan, J., Li, X., Wang, Y., and Zhou, Y. (2012b). Study on chemical constituents of analgesic active parts of Typha angustifolia L. South Pharm. 10, 201–204. doi:10.3969/j.issn.1672-2981.2012.03.013
Fu, L., Zhao, T., Dong, F., Zhou, Q., and Wang, Y. (2017). Study on the effect of Typha angustifolia L. solution on pain regulation in rats with inflammatory pain. Heilongjiang J. Anim. Sci. Vet. Med. 10, 32–35. doi:10.195270/2017-19-062
Fu, X., He, Y., Chen, W., Ying, J., and Wang, W. (2010). Effects of total flavonoids from Typha angustifolia L. on the proliferation, differentiation and related gene expression of 3T3-L1 preadipocytes. J. Med. Postgrad. 23, 456–459. doi:10.16571/j.cnki.1008-8199.2010.05.002
Gao, Q., and Tian, J. (2001). Research progress of Typha angustifolia. J. Neimeng Gu Tradit. Chin. Med. 10, 81–83. doi:10.16040/j.cnki.cn15-1101.2001.s1.103
Ge, F., Kuang, H., Wang, S., and Zhou, Z. (2002). Experimental study on the analgesic effect of Typha angustifolia. J. Xianning Univ. Sci. 2, 117–118. doi:10.16751/j.cnki.2095-4646.2002.02.019
Ge, H., and Jia, T. (2011). Determination of auramine O in illegally stained Chinese herbs Typha angustifolia and Scutellaria baicalensis decoction slices by LC-MS/PAD. Liaoning J. Tradit. Chin. Med. 38, 1616–1618. doi:10.13192/j.ljtcm.2011.08.147.gekq.068
Grebenshchykova, Z., Frédette, C., Chazarenc, F., Comeau, Y., and Brisson, J. (2020). Establishment and potential use of woody species in treatment wetlands. Int. J. Phytoremediation 22, 295–304. doi:10.1080/15226514.2019.1658712
Guo, J., Ma, L., and Liu, F. (2019). Study on chemical components in Typha angustifolia L. leaves. J. Shenyang Pharm. Univ. 36, 874–877. doi:10.14066/j.cnki.cn21-1349/r.2019.10.004
He, G., Zhou, W., Wang, L., Li, Y., Qiang, J., and Chen, Y. (2014). Screening of effective anti-thrombotic parts of Typha angustifolia. Chin. J. Exp. Tradit. Med. 20, 138–141. doi:10.13422/j.cnki.syfjx.2014100138
He, Y., Wang, W., Chen, W., and Ying, J. (2008). Effects of total flavonoids from Typha angustifolia L. on the expression of peroxisome proliferator-activated receptor family mRNA genes in 3T3-L1 adipocytes. J. Chin. Integr. Med. 6, 16–19. doi:10.15273/j.cnki.02.2008-09-016
He, Y., Wang, W., Chen, W., Ying, J., He, C., Ma, Y., et al. (2006a). Effects of total flavonoids from Typha angustifolia L. on glucose and lipid metabolism in 3T3-L1 adipocytes. J. Chin. Integr. Med. 4, 593–595. doi:10.3736/jcim20060610
He, Y., Wang, W., Chen, W., Ying, J., He, C., Ma, Y., et al. (2006b). Effects of total flavonoids from Typha angustifolia L. on glucose and lipid metabolism in 3T3-L1 adipocytes. J. Integr. Med. 4, 593–595. doi:10.3736/jcim20060610
Jiang, L., and Huang, W. (2009). Experimental study on the effect of Typha angustifolia L. on rats with atherosclerosis induced by hyperlipidemia. J. Emerg. Tradit. Chin. Med. 18, 770–773. doi:10.3969/j.issn.1004-745X.2009.05.055
Jiang, L., Tang, X., Wang, Y., and Huang, W. (2008). Effects of Typha angustifolia L. on liver low-density lipoprotein receptor gene in atherosclerotic rats. Laser J. 29, 95. doi:10.3969/j.issn.0253-2743.2008.06.051
Kong, X., Chen, P., Zhang, L., Shan, M., Cao, Y., Su, S., et al. (2011). Effects of Typha angustifolia L. charcoal on blood rheology and coagulation time in rats with blood stasis. Chin. J. Exp. Tradit. Med. 17, 129–132. doi:10.13422/j.cnki.syfjx.2011.06.036
Lai, X., Xu, Z., and Yuan, B. (2016). Effect of Typha angustifolia L. decoction on the contractile activity of isolated uterine smooth muscle in rats in late pregnancy. China Pharm. 27, 3. doi:10.6039/j.issn.1001-0408.2016.01.18
Li, G., Xu, S., Zhao, L., Lu, G., Sun, D., Wang, Y., et al. (2015). Study on the correlation analysis of Typha angustifolia L. quality characterization based on the quality evaluation model of drug system. J. Beijing Univ. Tradit. Chin. Med. 38, 393–410. doi:10.3969/j.issn.1006-2157.2015.06.007
Li, J., Chen, C., and Li, W. (2011). Effect of alcohol extracts of Typha angustifolia L. on immune function in C57BL/6 tumor-bearing mice. J. Anhui Agric. Sci. 39, 3. doi:10.3969/j.issn.0517-6611.2011.18.033
Li, J., Zhu, W., and Zhou, H. (2006). Catalytic synthesis of dimethyl phthalate. Appl. Chem. Ind. 2, 122–124. doi:10.16581/j.cnki.issn1671-3206.2006.02.016
Li, Y., and Xu, S. (2018). Effect of Typha angustifolia L. differentiation on patients with and its influence on absorption and fibrinolysis state of fundus hemorrhage. Guangming J. Chin. Med. 33, 3. doi:10.3969/j.issn.1003-8914.2018.17.020
Liao, M., Liu, Y., and Xiao, P. (1990). The study on flavonoids in Typha angustifolia L. leaves. Acta Bot. Sin. 32, 21–24. doi:10.3969/j.cnki.0253-2395.1990.04.018
Lin, J., Jia, C., Wang, R., Wang, X., Xiao, Y., and Ye, Z. (2011). Protective effect of flavonoids from Typha angustifolia L. on vascular endothelial cells damaged by hypoxia. J. Hunan Univ. Chin. Med. 31, 4–7. doi:10.3969/j.issn.1674-070X.2011.05.003.010.03
Liu, B., Lu, Y., and Zhang, T. (1998). Study on chemical constituents of Typha angustifolia L. Chin. Pharm. J. 33, 4–7. doi:10.1233/j.cnki.1998-10-004
Liu, Y., Feng, X., Wang, W., Wang, T., Chen, Y., and Chen, W. (2014). Effects of total flavonoids from Typha angustifolia L. on glucose consumption and Src protein expression in C2C12 skeletal muscle cells. Chin. Tradit. Pat. Med. 36, 3. doi:10.3969/j.issn.1001-1528.2014.07.040
Liu, Y., Shi, L., Qiu, W., and Shi, Y. (2022). Ferulic acid exhibits anti-inflammatory effects by inducing autophagy and blocking NLRP3 inflammasome activation. Mol. Cell. Toxicol. 18, 509–519. doi:10.1007/s13273-021-00219-5
Liu, Y., Zhao, Y., Wang, X., Kong, L., Guo, M., Fang, Y., et al. (2021). Effects of Typha angustifolia L. on PARP1/MAPK signaling pathway gene expression in rats with acute spinal cord injury. Chin. J. Tradit. Chin. Med. Pharm. 36, 3133–3137.
Lou, M., Liu, C., Wu, J., Chen, W., and Ding, G. (2017). The role of sodium-potassium ATPase in the inhibition of calcification of human vascular smooth muscle cells in Typha angustifolia L. J. Wuhan. Univ. 2, 5. doi:10.14188/j.1671-8852.2017.02.007
Lou, S., Liu, Y., Chen, W., Ying, J., He, Y., and Wang, W. (2008a). Effect of total flavonoids from Typha angustifolia L. on glucose metabolism in C2C12 skeletal muscle cells under palmitate culture. J. Hunan Univ. Chin. Med. 2, 39–42. doi:10.16306/j.1008-861x.2008.02.018
Lou, S., Liu, Y., Chen, W. H., Ying, J., He, Y., and Wang, W. (2008b). Total flavonoids from Typha angustifolia L. inhibit the expression of interleukin-6 in C2C12 skeletal muscle cells cultured with palmitic acid. J. Integr. Med. 5, 488–492. doi:10.3969/j.issn.1008-861X.2008.02.013
Lu, Z., Du, Y., Tang, Y., and Chen, J. (2018). Study on the content of Isorhamnetin-3-O-neohesperidin and Typhaneoside in Typha angustifolia L. with different maturity. J. Clin. Med. Lit. 5, 172–174. doi:10.16281/j.cnki.jocml.2018.11.099
Ma, C., Chen, P., Zhang, L., Li, X., and Ding, A. (2010). Experimental study on the effects of Typha angustifolia L. charcoal on the coagulation system of rats. J. Nanjing Univ. Tradit. Chin. Med. 26, 42–43. doi:10.14148/j.issn.1672-0482.2010.01.022
Ma, J., Kang, M., Chen, X., Li, Y., Wu, B., Feng, X., et al. (2014). Study on purification technology of total flavonoids from Typha angustifolia L. by macroporous resin. J. Guangxi Univ. Chin. Med. 17, 78–81. doi:10.10820/2014-01-038
Medical Department Pharmacopoeia Committee (1998). Ministry of health drug standard: traditional Chinese medicine prescription preparation. Beijing: People's Health Publishing House.
Miao, X. (2011). Classic of the materia medica. Beijing, China: Medical Science and Technology Press.
Mo, R. (2019). Clinical observation and mechanism study of Typha angustifolia L. in the treatment of unstable angina pectoris patients with blood stasis. (Doctoral dissertation). Guangzhou University of Traditional Chinese Medicine.
Mo, W., Qin, Y., Huang, Y., and Qin, L. (2009). Research progress of Typha angustifolia L. Pharm. Today. 19, 5–8. doi:10.11256/j.cnki140-46.10.2009-08-013
Monaco, P., Greca, M., and Previtera, L. (1990). Stigmasterols from Typha angustifolia. J. Nat. Prod. 53, 1430–1435. doi:10.1021/np50072a005
Nabuyanda, M., Bruggen, J., Kelderman, P., and Irvine, K. (2019). Investigating Co, Cu, and Pb retention and remobilization after drying and rewetting treatments in greenhouse laboratory-scale constructed treatments with and without Typha angustifolia L., and connected phytoremediation potential. J. Environ. Manage. 15, 510–518. doi:10.1016/j.jenvman.2019.02.016
Nanjing University of Traditional Chinese Medicine (2006). Great dictionary of Chinese medicine. Shanghai, China: Science and Technology Press.
National Medical Products Administration (2002). Compilation of national standards for proprietary Chinese medicine. Beijing: People's Health Publishing House.
Qi, L., Zhou, J., and Zhu, L. (2022). Clinical study of Moluodan combined with Itopride in the treatment of chronic atrophic gastritis. Drug Clin. 38, 15–18. doi:10.3969/j.cnki.1673-7202.2022.38.052
Qiang, W., Zhang, C., and Xu, D. (1987). Effects of Typha angustifolia L., vitamins E and C on prostacyclin synthesis and lipid peroxide production in rat arterial endothelial cells. Acta Univ. Med. Shanghai. 4, 296–300.
Qin, Z., Zhu, C., Zhang, K., and Zhou, S. (2017). Experimental study on the anti-prostatic hyperplasia of Typha angustifolia L. granules in mice. J. Pract. Tradit. Chin. Med. 33, 3. doi:10.12006/j.cnki.0201.2017-09-003
Reng, W., Huang, G., Xun, D., Qiao, L., Xun, Y., and Ding, J. (1989). Study on the effects of Typha angustifolia and Typha angustifolia L. preparations on hypercholesterolemia and macrophage function. Shanghai J. Tradit. Chin. Med. 8, 46–48. doi:10.16305/j.1007-1334.1989.08.032
Reyes, C., Villamar, C., Neubauer, M., Pozo, G., and Vidal, G. (2013). Behavior of Typha angustifolia L. in a free water surface constructed wetlands for the treatment of swine wastewater. J. Environ. Sci. Health. A Tox. Hazard. Subst. Environ. Eng. 48, 1216–1224. doi:10.1080/10934529.2013.776852
Shen, X., Liu, C., Chen, P., and Ding, A. (2015). Effects of the compatibility of Typha angustifolia L. and Wulingzhi on platelet parameters in blood stasis model rats. Mod. Chin. Med. 17, 11–14. doi:10.13313/j.issn.1673-4890.2015.1.003
State Administration of Traditional Chinese Medicine of the PR China (1999). Chinese materia medica. Shanghai, China: Science and Technology Press.
Takatsuto, S. (1997). Composition of phytosterols in the pollen of Typha angustifolia L. J. Jap. Chem. Soc. 46, 687–689,715. doi:10.5650/jos1996.46.687
Tang, M., Sun, F., Liang, S., Wang, S., and Ge, Y. (2022). Study on UPLC based fingerprint and multi-component content determination of Typha angustifolia L. J. Chin. Med. Mater 45, 1894–1898. doi:10.13863/j.issn1001-4454.2022.08.021
Tao, B., and Li, X. (2004). Experimental study on the effect of Typha angustifolia L. on atherosclerotic vascular endothelial damage. Chin. J. Integr. Med. Cardio-/Cerebrovascuiar Dis. 20, 121–127. doi:10.3969/j.issn.1672-1349.2004.04.020
Tao, W., Yang, N., Liu, L., Duan, J., Wu, D., Qian, D., et al. (2010). Two new cerebrosides from the pollen of Typha angustifolia L. Fitoterapia 81, 196–199. doi:10.1016/j.fitote.2009.08.026
Wang, D., Sun, G., and Wang, Y. (2017a). Effects of serum containing total flavonoids from Typha angustifolia L. on macrophage autophagy and Akt/mTOR signaling pathway. J. Pract. Med. 33, 51–55. doi:10.3969/j.issn.1006-5725.2017.16.012
Wang, L., Li, D., and Zhou, Q. (2003). Protective effect of Typha angustifolia L. extract of traditional Chinese medicine on cerebral ischemia-reperfusion injury in rats. Clin. J. Med. Off. 31, 2. doi:10.3969/j.issn.1671-3826.2003.03.001
Wang, L., Liao, M., and Xiao, P. (1998). Chemical and pharmacological activities of Chinese medicine Typha angustifolia. Lishizhen Med. Mat. Med. Res. 12, 49–50. doi:10.13463/j.cnki.szgyy.1998.03.12.021
Wang, X., Tian, X., Zhan, Y., Wu, H., Wang, Y., and Zhu, Y. (2017b). Selective inhibitory effects of Typha angustifolia L. and red paeony on platelet aggregation based on activity screening and target network prediction. Chin. J. Exp. Tradit. Med. 23, 120–126. doi:10.13422/j.cnki.syfjx.2017010120
Wang, Y., and Huang, W. (2010). Protective effect of Typha angustifolia L. extract on vascular endothelial cells damaged by oxidized low-density lipoprotein. Chin. J. Gerontol. 30, 948–951. doi:10.3969/j.issn.1005-9202.2010.07.034
Wang, Y., and Li, W. (2010). Study on the resistance of Typha angustifolia L. extract to oxidative damage in human umbilical vein endothelial cells. Chin. Arch. Tradit. Chin. Med. 28, 2193–2194. doi:10.7346/j.cnki.180-1301.2010-10-072
Wang, Y., and Li, W. (2012). Effect of Typha angustifolia L. extract on IL-8 expression in endothelial cells. Chin. Arch. Tradit. Chin. Med. 30, 1370–1371+1456. doi:10.13193/j.archtcm.2012.06.188.wangyh.065
Wen, H., Wang, Y., Peng, G., and Li, W. (2006). HPLC method was used to determine the content of total flavonoids in Typha angustifolia L. Res. Pract. Chin. Med. 3, 41–44. doi:10.3969/j.issn.1673-6427.2006.03.013
Wu, X., Dai, H., Xu, C., Liu, L., and Li, S. (2019). Citric acid modification of a polymer exhibits antioxidant and anti-inflammatory properties in stem cells and tissues. J. Biomed. Mat. Res. A 11, 2414–2424. doi:10.1002/jbm.a.36748
Wu, Y. (2013). New compilation of materia medica. Beijing, China: Traditional Chinese Medicine Press.
Xia, Y., Xiang, J., Liu, X., and Shi, Y. (2008). Effect of compound Typha angustifolia L. granules on heat shock protein 70 and its mRNA expression in rats with focal cerebral ischemia reperfusion. J. Tradit. Pharm. 49, 1119–1122. doi:10.13288/j.11-2166/r.2008.12.044
Xia, Y., Xiang, J., Liu, X., and Shi, Y. (2009). Effect of compound Typha angustifolia L. granules on neuronal apoptosis and caspase-3 mRNA expression in rats with focal cerebral ischemia-reperfusion. China Pharm. 20, 168–170.
Xu, L., Wang, S., Zhu, T., and Yu, D. (1987). Structure of cataphylactic acid from Typha angustifolia L. Acta Pharm. Sin. 10, 433–437. doi:10.16438/j.0513-4870.1987.06.007
Xue, W., Lin, L., Lin, Y., Guo, W., and Fu, C. (2023). The clinical study of Fujing Zhiling tablet combined with dydrogesterone in the treatment of dysfunctional uterine bleeding. Drug Clin. 38, 1991–1995. doi:10.7501/j.cnki.1674-5515.2016.08.021
Yang, H., and Li, J. (2012). Effects of total flavonoids from Typha angustifolia L. on blood rheology parameters and platelet aggregation in rabbits. Chin. J. Exp. Tradit. Med. 18, 244–246. doi:10.13422/j.cnki.syfjx.2012.17.075
Yang, Z., Wang, Y., and Zuo, J. (2021). Protective effect of Typha angustifolia L. extract on diabetic retinopathy rats. J. Int. Eye Sci. 21, 5. doi:10.3980/j.issn.1672-5123.2021.3.05
Yuan, J., and Xia, Y. (2014). Effect of compound Typha angustifolia L. decoction on Parkin and Cyclin E proteins in brain tissue of mice with ischemic brain injury. J. Tongji Univ. 35, 38–46. doi:10.3969/j.issn1008-0392.2014.05.008
Zeng, G., Wu, Z., Cao, W., Wang, Y., Deng, X., and Zhou, Y. (2018). Identification of anti-nociceptive constituents from the pollen of Typha angustifolia L. using effect-directed fractionation. Nat. Prod. Res. 34, 1041–1045. doi:10.1080/14786419.2018.1539979
Zhang, B. (1936). Convenient reader on materia medica. Shanxi, China: Taiyuan Medical Science and Technology Press.
Zhang, J., Feng, C., and Fu, L. (2017). Study on the analgesic effect of Typha angustifolia L. on trained rats. Heilongjiang J. Anim. Sci. Vet. Med. 15, 3–4. doi:10.160/j.issn.01.2017-09-060
Zhang, J., Zhou, Z., and Zuo, B. (2003). Protective effect of Typha angustifolia L. on endothelial damage caused by hyperlipidemia. Pharmacol. Clin. Chin. Mat. Med. 19, 3. doi:10.3969/j.issn.1001-859X.2003.04.013
Zhang, L., Wang, Z., Xu, Y., Qiao, L., and Sun, M. (1987). Effects of Typha angustifolia L. on TXA2/PGI2 and blood lipids in rabbits with acute hyperlipidemia. Chin. J. Pathophysiol. 14, 211–214.
Zhang, X., Liu, L., Rao, Y., Zhang, X., and Li, S. (2013). Effect of compound Typha angustifolia L. extract on rat model of nephritis hematuria. Chin. Pharm. J. 48, 1919–1922. doi:10.11669/cpj.2013.22.008
Zhang, Y., Chen, J. M., Song, G., He, Z., and Feng, X. (2019). UPLC method was used to simultaneously determine the two flavonoids in Typha angustifolia L. Guangzhou Chem. Ind. 47, 94–97. doi:10.10129/j.cnki.2019-19-037
Zhang, Z. (1983). Reverence for the origin of the materia medica. Beijing, China: Medical Science and Technology Press.
Zhao, G., and Zhu, Q. (2011). Anti-thrombotic effect of Typha angustifolia L. decoction in rats. Chin. J. Biochem. Pharm. 32, 3–4. doi:10.182/j.cnki.2011-03-017
Zhao, L., and Xu, K. (2007). Comprehensive development and utilization of wild plant resources Typha angustifolia. Chin. J. For. Byprod. 1, 80–81. doi:10.3969/j.issn.1001-6902.2007.01.035
Zheng, R. (2018). Water purification and resource utilization of wetland aquatic plants. Environ. Dev. 30, 206–208. doi:10.16647/j.cnki.cn15-1369/X.2018.08.123
Zheng, Y., Zhao, J., Miao, D., Xu, T., Wang, L., Liu, C., et al. (2023). Hepatoprotective effect of Typhaneoside on non-alcoholic fatty liver disease via farnesoid X receptor in vivo and in vitro. Biomed. Pharmacother. 164, 114957. doi:10.1016/j.biopha.2023.114957
Zhou, F., Li, A., Xie, J., and Yang, X. (2006). Experimental study on the anti-hyperlipidemia and atherosclerosis effects of Typha angustifolia L. Quail. Chin. J. Exp. Tradit. Med. 8, 2. doi:10.3969/j.issn.1005-9903.2006.08.021
Zhu, C., Qin, Z., Zhang, K., and Zhou, S. (2018). Experimental study on the mechanism of Typha angustifolia L. granules against prostatic hyperplasia in mice. Lab. Med. Clin. 15, 3. doi:10.117/j.cnki.10.2018-18-007
Keywords: Typha angustifolia L., botany, traditional uses, phytochemistry, pharmacological activity, quality control
Citation: Liu J, Chen Z, Tao S, Shu X, Shao B, Zhang X, Yang Y and Wang X (2025) Traditional uses and emerging therapeutic potential of Typha angustifolia L.: insights into its phytochemistry, pharmacological activities and quality control. Front. Pharmacol. 16:1557136. doi: 10.3389/fphar.2025.1557136
Received: 08 January 2025; Accepted: 21 March 2025;
Published: 01 April 2025.
Edited by:
Karim Hosni, Institut National de Recherche et d’Analyse Physico-Chimique (INRAP), TunisiaReviewed by:
Neeraj Khatri, Institute of Microbial Technology (CSIR), IndiaCopyright © 2025 Liu, Chen, Tao, Shu, Shao, Zhang, Yang and Wang. This is an open-access article distributed under the terms of the Creative Commons Attribution License (CC BY). The use, distribution or reproduction in other forums is permitted, provided the original author(s) and the copyright owner(s) are credited and that the original publication in this journal is cited, in accordance with accepted academic practice. No use, distribution or reproduction is permitted which does not comply with these terms.
*Correspondence: Yang Yang, eWFuZ3lhbmc5ODYyMEAxMjYuY29t; Xiaoli Wang, eGlhb0x3d3dhbmdAMTYzLmNvbQ==
†These authors have contributed equally to this work
Disclaimer: All claims expressed in this article are solely those of the authors and do not necessarily represent those of their affiliated organizations, or those of the publisher, the editors and the reviewers. Any product that may be evaluated in this article or claim that may be made by its manufacturer is not guaranteed or endorsed by the publisher.
Research integrity at Frontiers
Learn more about the work of our research integrity team to safeguard the quality of each article we publish.