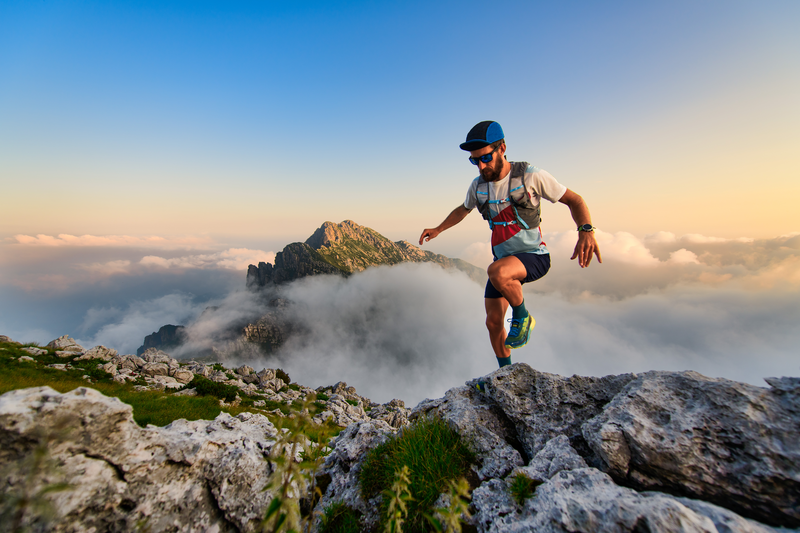
94% of researchers rate our articles as excellent or good
Learn more about the work of our research integrity team to safeguard the quality of each article we publish.
Find out more
REVIEW article
Front. Pharmacol.
Sec. Neuropharmacology
Volume 16 - 2025 | doi: 10.3389/fphar.2025.1557133
This article is part of the Research Topic New Strategies for Spinal Cord Injury and Immunotherapy Targeting Novel Programmed Death Pathways View all articles
The final, formatted version of the article will be published soon.
You have multiple emails registered with Frontiers:
Please enter your email address:
If you already have an account, please login
You don't have a Frontiers account ? You can register here
Spinal cord injury (SCI) is a severe traumatic condition that frequently results in various neurological disabilities, including significant sensory, motor, and autonomic dysfunctions. Ferroptosis, a recently identified non-apoptotic form of cell death, is characterized by the accumulation of reactive oxygen species (ROS), intracellular iron overload, and lipid peroxidation, ultimately culminating in cell death.Recent studies have demonstrated that ferroptosis plays a critical role in the pathophysiology of SCI, contributing significantly to neural cell demise. Three key cellular enzymatic antioxidants such as glutathione peroxidase 4 (GPX4), ferroptosis suppressor protein 1 (FSP1), and dihydroorotate dehydrogenase (DHODH), have been elucidated as crucial components in the defense against ferroptosis. Natural products, which are bioactive compounds mostly derived from plants, have garnered considerable attention for their potential therapeutic effects. Numerous studies have reported that several natural products can effectively mitigate neural cell death and alleviate SCI symptoms. This review summarizes fifteen natural products that have shown promise in treating SCI by inhibiting ferroptosis:containing (-)-Epigallocatechin-3-gallate (EGCG), Proanthocyanidin, Carnosic acid, Astragaloside IV, Trehalose, 8-gingerol, Quercetin, Resveratrol, Albiflorin, Alpha-tocopherol, Celastrol, Hispolon, Dendrobium Nobile Polysaccharide, Silibinin, and Tetramethylpyrazine. that have shown promise in treating SCI by inhibiting ferroptosis. Additionally, this review provides an overview of the mechanisms involved in these studies and proposes several perspectives to guide future research directions.
Keywords: spinal cord injury, ferroptosis, natural product, GPx4, ROS
Received: 08 Jan 2025; Accepted: 10 Mar 2025.
Copyright: © 2025 She, Junxiao, Ma, Ma, Li, Zhang, Qiu and Li. This is an open-access article distributed under the terms of the Creative Commons Attribution License (CC BY). The use, distribution or reproduction in other forums is permitted, provided the original author(s) or licensor are credited and that the original publication in this journal is cited, in accordance with accepted academic practice. No use, distribution or reproduction is permitted which does not comply with these terms.
* Correspondence:
Cheng Qiu, Peking Union Medical College Hospital (CAMS), Beijing, China
Disclaimer: All claims expressed in this article are solely those of the authors and do not necessarily represent those of their affiliated organizations, or those of the publisher, the editors and the reviewers. Any product that may be evaluated in this article or claim that may be made by its manufacturer is not guaranteed or endorsed by the publisher.
Research integrity at Frontiers
Learn more about the work of our research integrity team to safeguard the quality of each article we publish.