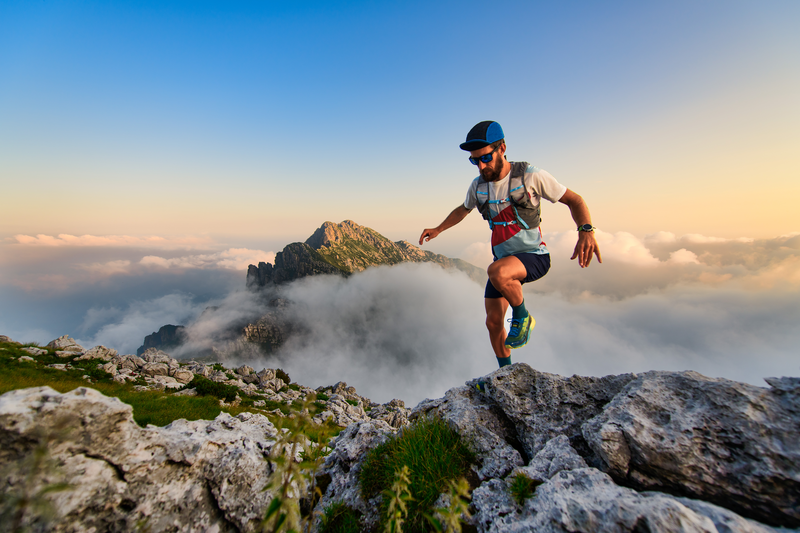
94% of researchers rate our articles as excellent or good
Learn more about the work of our research integrity team to safeguard the quality of each article we publish.
Find out more
ORIGINAL RESEARCH article
Front. Pharmacol.
Sec. Ethnopharmacology
Volume 16 - 2025 | doi: 10.3389/fphar.2025.1556579
This article is part of the Research Topic The Application of Network Analysis in Ethnopharmacology and Food Nutrition Volume II View all 4 articles
The final, formatted version of the article will be published soon.
You have multiple emails registered with Frontiers:
Please enter your email address:
If you already have an account, please login
You don't have a Frontiers account ? You can register here
Gut homeostasis is critical for human health, ulcerative colitis (UC) can disrupt gut homeostasis and cause disease. Panax ginseng C.A. Meyer is a widely used traditional herbal medicine known for its anti-inflammatory, antioxidant, and immunomodulatory effects. However, the protective mechanisms of total ginsenosides (TG) in treating UC remain unclear. In this study, we employed Drosophila melanogaster as a model organism to investigate the protective effects of TG on dextran sulfate sodium (DSS)-induced intestinal injury. Our data showed that TG significantly improved survival rates in female flies, restored intestinal length, maintained intestinal barrier integrity, and alleviated oxidative stress. Additionally, TG may protect against intestinal damage by activating the PI3K/Akt signaling pathway and inhibiting the JAK/STAT signaling pathway. These findings suggest that TG alleviates UC symptoms through multi-target regulation, highlighting its potential for developing novel therapeutic strategies for UC.
Keywords: Total ginsenosides, ulcerative colitis, Network analysis, Drosophila melanogaster, Intestinal Homeostasis
Received: 07 Jan 2025; Accepted: 06 Mar 2025.
Copyright: © 2025 He, Sun, Gu, Zheng, Wang and Sun. This is an open-access article distributed under the terms of the Creative Commons Attribution License (CC BY). The use, distribution or reproduction in other forums is permitted, provided the original author(s) or licensor are credited and that the original publication in this journal is cited, in accordance with accepted academic practice. No use, distribution or reproduction is permitted which does not comply with these terms.
* Correspondence:
Yanyan Sun, Jilin Academy of Agricultural Sciences (CAAS), Changchun, 130033, Jilin Province, China
Disclaimer: All claims expressed in this article are solely those of the authors and do not necessarily represent those of their affiliated organizations, or those of the publisher, the editors and the reviewers. Any product that may be evaluated in this article or claim that may be made by its manufacturer is not guaranteed or endorsed by the publisher.
Research integrity at Frontiers
Learn more about the work of our research integrity team to safeguard the quality of each article we publish.