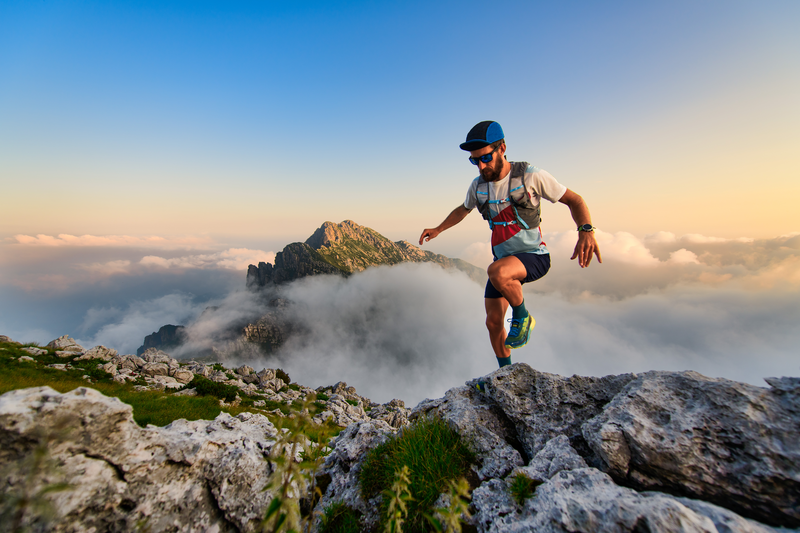
94% of researchers rate our articles as excellent or good
Learn more about the work of our research integrity team to safeguard the quality of each article we publish.
Find out more
MINI REVIEW article
Front. Pharmacol.
Sec. Experimental Pharmacology and Drug Discovery
Volume 16 - 2025 | doi: 10.3389/fphar.2025.1555115
The final, formatted version of the article will be published soon.
You have multiple emails registered with Frontiers:
Please enter your email address:
If you already have an account, please login
You don't have a Frontiers account ? You can register here
Several preclinical assays and clinical trials have found hypothermia as an efficient protective treatment for stroke. However, systemic hypothermia impairs several physiological functions being difficult to implement in acute critical patients. A deeper understanding of the mechanisms underlying the therapeutic effects of hypothermia could inspire new treatments based on the protective effects of cold. Furthermore, this could contribute to the reduction of the side effects associated with it. One of the metabolic landmarks of hypothermia is the overexpression of a small subset of shock proteins while global protein synthesis is reduced. Among these cold-shock proteins, RBM3 (RNA-binding motif protein 3) seems to play a central protective role. In physiological conditions, RBM3 is involved in the regulation of protein synthesis. In several models of cerebral diseases, in vitro and in vivo, RBM3 exhibited the ability to mitigate apoptosis or increase neural proliferation. In stroke models, RBM3 has shown specially promising effects attenuating neural damage and enhancing cell survival. Future prospects should be directed towards the design of efficient strategies to modulate RBM3 levels. This mini review aims to summarize the progress made in understanding the role of RBM3 in cerebral tissue protection, while encouraging efforts to address research gaps, particularly in its modulation and clinical application.
Keywords: Brain, Hypothermia, Ischemia, modulation, Neuroprotection, RBM3, Stroke, therapy
Received: 03 Jan 2025; Accepted: 12 Feb 2025.
Copyright: © 2025 Ávila-Gómez, Vieites-Prado, Correa-Paz, Del Pozo-Filíu, Palomar-Alonso, Campos and López-Arias. This is an open-access article distributed under the terms of the Creative Commons Attribution License (CC BY). The use, distribution or reproduction in other forums is permitted, provided the original author(s) or licensor are credited and that the original publication in this journal is cited, in accordance with accepted academic practice. No use, distribution or reproduction is permitted which does not comply with these terms.
* Correspondence:
Francisco Campos, Health Research Institute of Santiago de Compostela (IDIS), Santiago de Compostela, 15706, Galicia, Spain
Esteban López-Arias, Health Research Institute of Santiago de Compostela (IDIS), Santiago de Compostela, 15706, Galicia, Spain
Disclaimer: All claims expressed in this article are solely those of the authors and do not necessarily represent those of their affiliated organizations, or those of the publisher, the editors and the reviewers. Any product that may be evaluated in this article or claim that may be made by its manufacturer is not guaranteed or endorsed by the publisher.
Research integrity at Frontiers
Learn more about the work of our research integrity team to safeguard the quality of each article we publish.