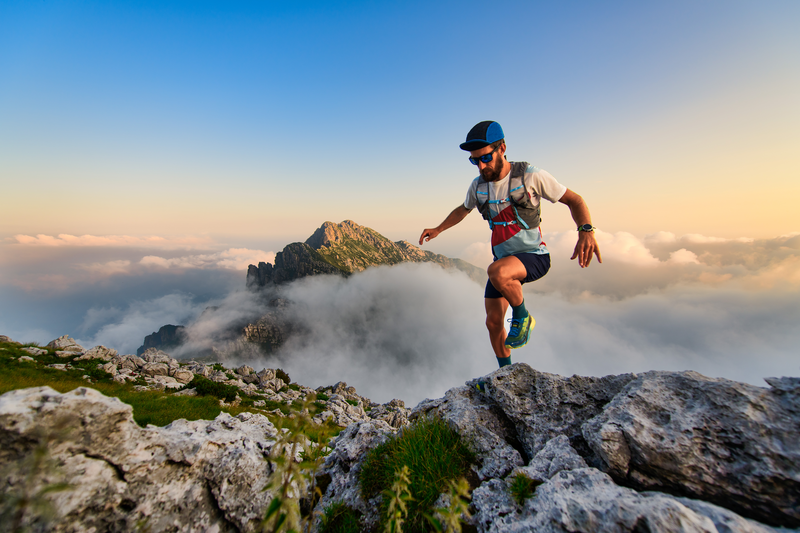
94% of researchers rate our articles as excellent or good
Learn more about the work of our research integrity team to safeguard the quality of each article we publish.
Find out more
ORIGINAL RESEARCH article
Front. Pharmacol.
Sec. Neuropharmacology
Volume 16 - 2025 | doi: 10.3389/fphar.2025.1555079
The final, formatted version of the article will be published soon.
You have multiple emails registered with Frontiers:
Please enter your email address:
If you already have an account, please login
You don't have a Frontiers account ? You can register here
Cognitive impairments following post-stroke significantly hinder neurological recovery and exacerbate patient morbidity, underscoring urgent need for effective therapeutic strategies. Vortioxetine (VTX), a prominent Selective Serotonin Reuptake Inhibitor (SSRI), boasts notable antidepressant, cognition-enhancing, and antiinflammatory properties. This investigation delves into VTX's influence on motor skills, spatial learning-memory capabilities, and depressive behaviors in Middle Cerebral Artery Occlusion (MCAO) rats, alongside its underlying mechanisms. Our findings reveal that while VTX fails to entirely reverse ischemic-reperfusion damage, it substantially ameliorates spontaneous locomotor functions, augments post-stroke learning-memory capacities, and exhibits potent antidepressant and anxiety-like efficacy. Preliminary data propose that these beneficial effects may stem from inflammation modulation via the Toll-Like Receptor 2 (TLR-2)/Nuclear Factor-Kappa B (NF-κB) signaling pathway. Collectively, our work underscores VTX's promising role in enhancing motor, cognitive functions, and mitigating depressive symptoms following cerebrovascular accidents, potentially through inflammation regulation. These insights pave the way for novel interventions addressing post-stroke complications, warranting further exploration.
Keywords: Vortioxetine, ischemic stroke, cognitive dysfunction, Neuroinflammation, TLR-2/NF-κB pathway
Received: 03 Jan 2025; Accepted: 12 Feb 2025.
Copyright: © 2025 Zou, Dong, Dong, Xu, Li, Wang and Huang. This is an open-access article distributed under the terms of the Creative Commons Attribution License (CC BY). The use, distribution or reproduction in other forums is permitted, provided the original author(s) or licensor are credited and that the original publication in this journal is cited, in accordance with accepted academic practice. No use, distribution or reproduction is permitted which does not comply with these terms.
* Correspondence:
Zhengqiang Zou, Department of Planning and Quality Control, Ganzhou Hospital-Nanfang Hospital, Southern Medical University, Ganzhou, China
Disclaimer: All claims expressed in this article are solely those of the authors and do not necessarily represent those of their affiliated organizations, or those of the publisher, the editors and the reviewers. Any product that may be evaluated in this article or claim that may be made by its manufacturer is not guaranteed or endorsed by the publisher.
Research integrity at Frontiers
Learn more about the work of our research integrity team to safeguard the quality of each article we publish.