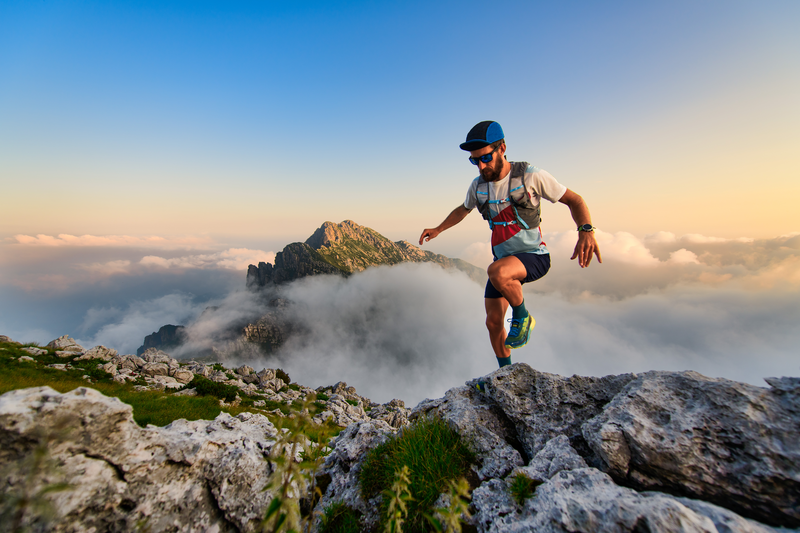
94% of researchers rate our articles as excellent or good
Learn more about the work of our research integrity team to safeguard the quality of each article we publish.
Find out more
ORIGINAL RESEARCH article
Front. Pharmacol.
Sec. Ethnopharmacology
Volume 16 - 2025 | doi: 10.3389/fphar.2025.1554479
This article is part of the Research Topic Plant Metabolites in Drug Discovery: The Prism Perspective between Plant Phylogeny, Chemical Composition, and Medicinal Efficacy, Volume IV View all articles
The final, formatted version of the article will be published soon.
You have multiple emails registered with Frontiers:
Please enter your email address:
If you already have an account, please login
You don't have a Frontiers account ? You can register here
Ovarian function decline results in reduced estrogen levels, leading to endocrine disorders, oxidative stress damage, and excessive activation of inflammatory factors, all of which contribute to the development of premenstrual syndrome (PMS). Kunxinning Granules (KXN) has been clinically approved for PMS treatment, but its bioactive ingredients and mechanism of action remain unclear. This study aimed to investigate the active metabolites and molecular mechanism of KXN in treating PMS rats, laying a foundation for the clinical development of PMS treatment.Methods: An ovariectomized (OVX) rat model was established to evaluate the efficacy of KXN in treating PMS. Molecular network (MN) analysis, combined with UPLC/Q-TOF-MS, identified prototype compounds in the samples and constructed a chemical classification map based on their structures. A network analysis and proteomics were conducted to predict potential pathways through which KXN regulates PMS. Quantitative metabolomics assays were used to confirm these potential pathways. Additionally, target prediction and binding enzyme activity detection elucidated the key active metabolites and mechanisms of action in KXN.Results: KXN exhibited significant effectiveness in supplementing estrogen deficiency and uterine atrophy in the OVX model. We identified 16 absorbed metabolites as the potential pharmacological ingredients of KXN in vivo. The steroid hormone biosynthesis pathway, a crucial pathway of KXN in PMS, played a key role in KXN's effectiveness. KXN improved hormonal metabolic disorders by regulating this pathway. The main metabolites in KXN, including astragaloside IV, icariin and baohuoside I increased estradiol levels by enhancing the activity of CYP19A1, the representative enzyme in hormone biosynthesis pathway.Discussion: This study shows that KXN could relieve anxiety, depression, and osteoporosis in PMS. This pharmacological effect is exerted through steroid hormone synthesis to address estrogen deficiency. The findings provide valuable insights into the underlying mechanisms and support its clinical application.
Keywords: Kunxinning granules, Perimenopausal Syndrome, Hormone disorders, Steroid hormone biosynthesis pathway, Molecular network, multi omics analysis. Article types
Received: 02 Jan 2025; Accepted: 10 Mar 2025.
Copyright: © 2025 Wang, Yang, Wang, Gao, Liu, Zhang, Li, Zhang, Zhou, Hou and Bai. This is an open-access article distributed under the terms of the Creative Commons Attribution License (CC BY). The use, distribution or reproduction in other forums is permitted, provided the original author(s) or licensor are credited and that the original publication in this journal is cited, in accordance with accepted academic practice. No use, distribution or reproduction is permitted which does not comply with these terms.
* Correspondence:
Guirong Zhou, Tasly Pharmaceutical Group (China), Tianjin, 300410, China
Yuanyuan Hou, College of Pharmacy, Nankai University, Tianjin, China
Gang Bai, College of Pharmacy, Nankai University, Tianjin, China
Disclaimer: All claims expressed in this article are solely those of the authors and do not necessarily represent those of their affiliated organizations, or those of the publisher, the editors and the reviewers. Any product that may be evaluated in this article or claim that may be made by its manufacturer is not guaranteed or endorsed by the publisher.
Research integrity at Frontiers
Learn more about the work of our research integrity team to safeguard the quality of each article we publish.