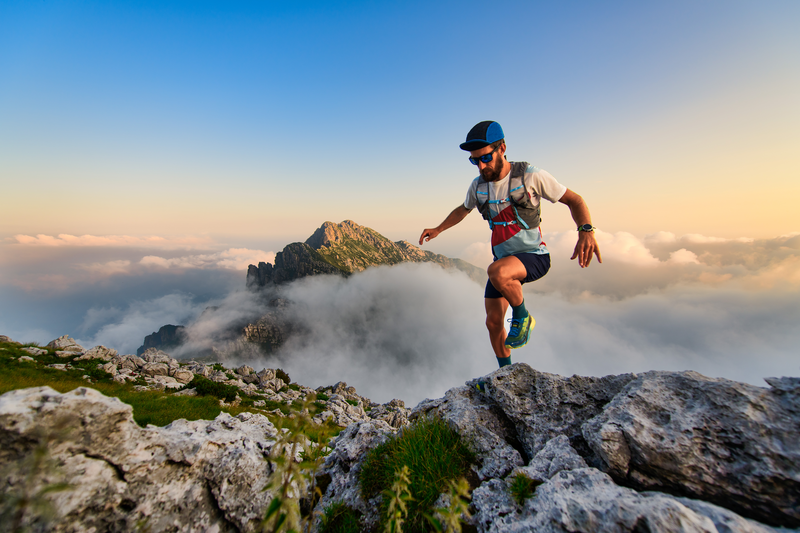
95% of researchers rate our articles as excellent or good
Learn more about the work of our research integrity team to safeguard the quality of each article we publish.
Find out more
ORIGINAL RESEARCH article
Front. Pharmacol.
Sec. Pharmacology of Anti-Cancer Drugs
Volume 16 - 2025 | doi: 10.3389/fphar.2025.1553791
This article is part of the Research Topic Enhancing Cancer Therapy: Integrating Plant-Derived Bioactives with Chemotherapy through Traditional Knowledge and Modern Advances View all 4 articles
The final, formatted version of the article will be published soon.
You have multiple emails registered with Frontiers:
Please enter your email address:
If you already have an account, please login
You don't have a Frontiers account ? You can register here
Background: Gastric cancer (GC) is one of the most common malignant tumors with poor survival. Although cisplatin is a first-line chemotherapy drug for GC, it still has the potential to develop drug resistance and side effects. Miltirone, extracted from Chinese herb Salvia miltiorrhiza Bunge, has been reported to significantly inhibit some types of cancer. However, its effects on GC have not been studied, the possible anti-tumor effects of miltirone in combination with cisplatin in GC patients have not been explored.Human GC cell lines AGS, HGC27, MKN45 and MGC803 cells were treated with miltirone and cisplatin individually or combinatorially. Cell proliferation assay, flow cytometric assay, colony formation assay and Western blot were employed to evaluate the cytotoxic effects under these treatments. Wound healing and transwell assays were used to examine the effects of miltirone and/or cisplatin on GC cell migration and invasion. RNA-seq analysis was used to determine miltirone 's potential target genes in AGS cells. GO analysis and molecular docking assay were used to determine the pathways affected by miltirone. Next, we examined changes in the selected pathway proteins. The in vivo animal model was verified the results of the in vitro experiments.Results: Miltirone inhibited cell growth, migration, and invasion, as well as induced apoptosis in GC cells. In combinatorial treatments, miltirone synergistically enhanced cytotoxicity of cisplatin in GC cells. Moreover, the expression levels of 606 genes appeared to be significantly modulated by miltirone via RNA-seq analyses, and PI3K/AKT signaling pathway was found to refer to miltirone activity. Furthermore, miltirone together with cisplatin treatment significantly reduced the expression levels of p-PI3K, p-Akt, p-mTOR, while the total levels of PI3K and Akt remained unchanged. In addition, compared with the control group, the tumors growth was significantly suppressed in groups treated with the two agents alone or in combination, and even more so in the combination group in vivo.Discussion: miltirone inhibited the proliferation of GC cells and significantly potentiates the anticancer activities of cisplatin by downregulating the PI3K/AKT signaling pathway. Combination therapy of miltirone and cisplatin represents a novel potential treatment of gastric cancer.
Keywords: gastric cancer, Miltirone, combination therapy, Cisplatin, PI3K/Akt signaling pathway
Received: 31 Dec 2024; Accepted: 11 Mar 2025.
Copyright: © 2025 Wang, Lv, Shen and Chen. This is an open-access article distributed under the terms of the Creative Commons Attribution License (CC BY). The use, distribution or reproduction in other forums is permitted, provided the original author(s) or licensor are credited and that the original publication in this journal is cited, in accordance with accepted academic practice. No use, distribution or reproduction is permitted which does not comply with these terms.
* Correspondence:
Li Shen, Institute of Basic Theory of TCM, China Academy of Chinese Medical Sciences, Beijing, China
Zhe Chen, First Affiliated Hospital, Zhejiang Chinese Medical University, Hangzhou, China
Disclaimer: All claims expressed in this article are solely those of the authors and do not necessarily represent those of their affiliated organizations, or those of the publisher, the editors and the reviewers. Any product that may be evaluated in this article or claim that may be made by its manufacturer is not guaranteed or endorsed by the publisher.
Research integrity at Frontiers
Learn more about the work of our research integrity team to safeguard the quality of each article we publish.