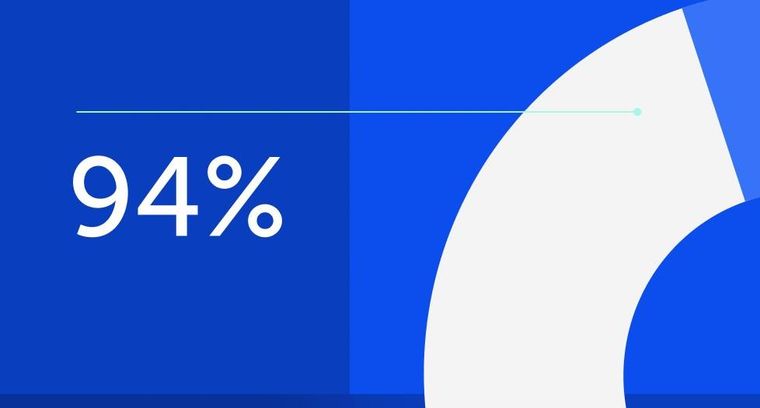
94% of researchers rate our articles as excellent or good
Learn more about the work of our research integrity team to safeguard the quality of each article we publish.
Find out more
REVIEW article
Front. Pharmacol., 20 March 2025
Sec. Experimental Pharmacology and Drug Discovery
Volume 16 - 2025 | https://doi.org/10.3389/fphar.2025.1553331
This article is part of the Research TopicExploiting Non-Oncogene Addiction for Overcoming Drug Resistance in Metastatic TumorsView all 3 articles
Rescue therapies of relapsed/refractory (r/r) Hodgkin’s lymphoma (HL) in the third to sixth-line provide major, yet unresolved problems. The MEPED regimen includes nuclear receptor agonists such as pioglitazone and dexamethasone, which counterbalance HL homeostasis, HL stress response inhibitors, everolimus and COX-2 inhibitor, and a stress response inducer, low-dose metronomic treosulfan. CR (six of seven patients) and long-term cCR in patients receiving no consolidating allogeneic stem cell transplantation highlight MEPED as a potent salvage therapy in advanced refractory HL. MEPED edits everolimus activities in such a way that mTORC1 becomes a non-oncogene addiction bottleneck, hence determining long-term therapy outcome. The implications of the therapeutic paradigm shift toward editing of HL tissue, and particularly mTOR addiction, could prove to be profound for clinical practice, both in terms of outcome and treatment tolerability. The long-term results of MEPED treatment indicate the urgent evaluation of the schedule in a multicenter trial for r/r HL.
The incidence of Hodgkin’s lymphomas (HLs) in 2020 was 0.4% for all newly reported cancer-related cases, and 0.2% of deaths were due to Hodgkin’ lymphoma (Huang et al., 2022). The current first-line treatment results for HL are outstanding. The 5-year event-free survival (EFS) is 79.1% for children with stage IIIB or IVB disease, with a high rate of involved-site radiation therapy (76%). An OS between 89% and 94% at 6 years has been observed for adult patients (Kelly and Friedberg, 2024). Nevertheless, 10 to 30% of patients show relapsing or refractory disease, highlighting the urgent quest to identify potent salvage therapies for those patients (Ansell, 2020; Grover et al., 2023). Salvage therapies are dose-intensive and include chemotherapy followed by high-dose chemotherapy with autologous stem cell transplantation (ASCT), targeted chemotherapy with brentuximab vedotin (BV), and immune checkpoint inhibitors (ICPi) such as nivolumab or pembrolizumab (Cellini et al., 2023). Allogeneic stem cell transplantation (allo-SCT) serves as a non-standard treatment but has a curative role in selected cases of relapsed/refractory (r/r) HL (Sureda et al., 2012; Mehta-Shah and Bartlett, 2018). Beyond these salvage treatments, no further standard therapy options exist for r/r HL, with particularly very few curative options (Chen et al., 2019; Kuruvilla et al., 2021; Johnson, 2023; Randall and Spinner, 2023). Clinical trials that focus on quality of life, comorbidity, and survival are necessary to improve survival rates for this expanding population with complex needs.
In HL tissues, few malignant CD30-positive Hodgkin–Reed–Sternberg (H-RS) cells are surrounded by an extended inflammatory stromal reaction comprising heterogeneous, non-neoplastic cell populations, consisting of a broad variety of not only hematologic cells but also mesenchymal cells and a variable degree of fibrosis in the tumor microenvironment (Dürkop et al., 1992; Steidl et al., 2011; Cader et al., 2018; Connors et al., 2020; Ribatti et al., 2022). Recently, we published literature on a less toxic therapy approach, the MEPED schedule, targeting non-oncogene addiction (NOA) targets in HR-S cells and in adjacent, by H-RS cells educated stroma cells with pioglitazone, dexamethasone, everolimus and etoricoxib, as well as low-dose metronomic chemotherapy with treosulfan. MEPED may induce continuous complete remission (cCR) in relapsed/refractory (r/r) HL, irrespective of the kind of pre-treatment used, either chemotherapy, BV, ICPi, autologous, or allogeneic SCT (Ugocsai et al., 2016; Lüke et al., 2021; Pophali et al., 2024; Reuthner et al., 2024).
Taking into account the outstanding results of MEPED in “last-line” therapy and respective modest therapy related side effects, MEPED deserves a detailed discussion in the context of HL pathophysiology, as well as the targeted NOA proteome that copes with HL stress responses and rewires HL hallmarks, thus providing novel perspectives for future HL treatment strategies in the r/r stage (Harrer et al., 2023b).
MEPED comprises various orally administered drugs, as depicted in Table 1, that specifically target the tumor microenvironment of HL. Pioglitazone acts to counterbalance HL homeostasis, while the mTOR inhibitor everolimus synergizes with the COX-2 inhibitors etoricoxib and dexamethasone to counteract HL inflammatory responses, which are mostly driven by mTOR. Finally, low-dose metronomic chemotherapy, such as a daily regimen of Treosulfan, is intended to disturb the vascular architecture and communicative network in the tumor microenvironment (Chan et al., 2018; Lüke et al., 2021).
Table 1. MEPED schedule: concerted transcriptional modulation combined with the induction of stress response.
In the first clinical trial evaluating MEPED in seven patients with refractory or relapsing HL following several lines of pretreatment (third- to sixth-line therapy, including allogeneic stem cell transplantation), a modest toxicity profile and a high rate of complete remissions were observed (Table 2). Moreover, it could be demonstrated that the all-oral MEPED treatment approach for r/r HL is applicable in an outpatient setting irrespective of comorbidities, treatment status, or the sequence of pretreatments. The immunomodulatory feature of MEPED was demonstrated by a significant decrease in C-reactive protein (CRP) in serum during objective response (Ugocsai et al., 2016), as well as a robust neurological improvement in a patient with severe myasthenia gravis who did not qualify for dose-intensive therapy (Fichtner et al., 2020). Regarding MEPED, it is important to emphasize that no single drug component, but the concerted strike of the modular system may induce CR or cCR (Lüke et al., 2021). Case in point, both the entire omission and dose reduction of everolimus below the trough level of 15 μg/L compromised the capacity of MEPED to achieve complete remission, rendering everolimus an essential component of MEPED (Kirchner et al., 2004). To date, several clinical trials have been conducted showing activity of mTOR inhibitors in patients with advanced refractory HL (Table 2). The MEPED schedule has been demonstrated to induce continuous complete remission from the third- to sixth-line therapy, even after the failure of allo-SCT (n = 1) and ASCT (Table 3). CRs occurred regardless of the previous treatment history, including chemotherapy, irradiation, BV, ICI, or autologous or allogeneic SCT, metastatic sites and respective tumor–host interfaces (lung or bone), and disease states (relapsed or refractory) before start of the MEPED schedule (Table 2). The initial intention of MEPED therapy was palliative. Shortening of the adjuvant therapy was possible due to the rapid CR induction within approximately 2–3 months.
Table 2. Outcome of clinical trials of mTOR inhibition as mono- or combination therapy and a trial with lenalidomide monotherapy in relapsed/refractory Hodgkin’s lymphoma (r/r HL).
To date, only a few oncogene addiction targets have been identified for the treatment of classic HL, with pulsed chemotherapy remaining the most important element for frontline therapy (Bröckelmann et al., 2023). Next, targeting the tumor immune microenvironment via CD30-targeted immunochemotherapy with brentuximab vedotin (BV) and/or an immune checkpoint inhibitor (ICPi) therapy were a considerable progress to HL therapy (Moskowitz et al., 2021; Fornecker et al., 2023; Herrera et al., 2023; Spinner et al., 2023; Alig et al., 2024). Moreover, targets such as mTOR, COX-2, peroxisome proliferator-activated receptor alpha/gamma (PPARα/γ), and the glucocorticoid receptor have attracted attention as promising avenue to target the inflammatory framework of the HL-related tumor microenvironment (Konopleva et al., 2004; Solimini et al., 2007; Luo et al., 2009; Harrer et al., 2023a; Cuéllar Mendoza et al., 2024). In addition, repetitive pulsed therapies exploit the possibilities to induce comprehensive apoptosis in r/r H-RS cells. Single NOA proteins may even be rate-limiting in HL stress-elicited response pathways, like mTOR. Others are available for re-adjusting pathological homeostatic balances, including nuclear receptor (NR) patterns such as PPARα/γ and the glucocorticoid receptor (Luo et al., 2009; Pierdominici et al., 2017; Chang et al., 2021; Harrer et al., 2023a).
In HL, tumor suppressor genes such as PTEN (Xia et al., 2018) are frequently dysregulated or inactivated, as is growth arrest and DNA damage-inducible protein (GADD45G) (Ying et al., 2005). Phosphatidylinositol 3-kinase/protein kinase B/mammalian target of rapamycin (PI3K/PTEN/AKT/mTOR) signaling appears dysregulated in HL, as it is in many histologically different tumor types (Mossmann et al., 2018). Although, mTOR inhibitors have been used in combined treatment schedules for many tumor types, no comparable long-term treatment results have been achieved (Hua et al., 2019).
The inhibitory function of the oncogenic PI3K/AKT/mTOR signaling network is absent in many tumor types as PTEN is frequently suppressed (Álvarez-Garcia et al., 2019). Pioglitazone may upregulate PTEN in neoplasia (Patel et al., 2001; Teresi and Waite, 2008; Esmaeili et al., 2021).
The resolution of post-therapy developing M-CRAC, i.e., metastases, cancer cell repopulation, acquired resistance, and tumor cell heterogeneity, following pulsed systemic apoptosis-inducing tumor therapies is a critical achievement of modular configured editing approaches targeting neoplastic and stromal cells (Harrer et al., 2023b).
An example of NOA targets distributed within many cell compartments in HL tissue represents the successful targeting of mTOR if edited as therapeutic bottleneck with the MEPED schedule (Figure 1) (Harrer et al., 2023b). ICPs in HL may frequently be targets of oncogene addiction due to the amplification of PD-1 (Gordon et al., 2017; Karihtala et al., 2022).
Figure 1. Hodgkin’s lymphoma (HL) tissue editing with MEPED: impact of target selection on patient performance status.
Poor clinical results following mTOR inhibition in r/r HL contrast the pivotal biological role of mTOR in HL stress responses. Activities of HL stress response pathways cumulatively enhance mTOR activity (Figure 2) (Jundt et al., 2005; Márk et al., 2013).
Figure 2. Communicatively integrating, counterregulating, and inhibiting non-oncogene addiction targets, while instigating HL stress response enables durable long-term responses in relapsed/refractory Hodgkin’s lymphoma (r/r HL).
Thus, optimizing the prerequisites for ensuring the efficacy of everolimus by strengthening mTOR addiction in r/r HL is an exemplary issue of how to establish a more efficacious use of inhibitory NOA proteins in the clinical setting.
Relatively few substrates of mTOR contrast with wide-ranging activities on many transcription factors, such as HIF-1α, NF-κB, and signaling pathways, that are involved in r/r HL pathophysiology and are either direct or indirect targets of pioglitazone and dexamethasone, both of which reprogram r/r HL tissue via PI3K, NF-κB, and Wnt pathways, as well as extrinsic and intrinsic apoptotic , STAT, and p53 pathways (Heudobler et al., 2018; Vallée and Lecarpentier, 2018; Rashid et al., 2021; Battaglioni et al., 2022; Glaviano et al., 2023; Harrer et al., 2023a). mTOR activity and constitutive high NF-κB expression are directly correlated in HL (Márk et al., 2013).
Phosphorylation of mTORC1 consistently suppresses multiple autophagy factors (Kim and Guan, 2015). Thus, damaged molecules may not be recycled in response to nutrient starvation (Dossou and Basu, 2019). The availability of nutrients and cellular stress modulate mTORC1 activity. Gain in mTOR and PI3K activity, gain-of-function of AKT, and decreased or lost function of PTEN are common promoters of treatment resistance and disease progression in neoplasia (Glaviano et al., 2023; Harrer et al., 2023b).
NOA proteins are undetectable by commonly used genetic and molecular genetic analyses due to only quantitative changes in their expression or altered expression patterns in the case of NRs. Currently, routinely performed front-line diagnostic approaches do not include NOA proteins.
However, if the intended therapies rely on unlocking HL-specific phenotypic characteristics, therapeutic targets derived from the NOA proteome are becoming more prominent and represent a huge, unexplored, modularly linked therapeutic tool that contributes to rewiring communicative interactions while covering the whole HL tissue.
Critical points for targeting NOA include the degree of addiction and the multifunctionality of an NOA target, such as mTOR, the appropriate selection of NR patterns and their selected on-topic activity in communicative networks, potential resistance mechanisms due to escape phenomena, and tumor cell heterogeneity (Harrer et al., 2023b) (Figure 1; Table 3).
mTORC1 plays a central role in activating stress-responsive transcription factors (Aramburu et al., 2014). Therefore, stress response mechanisms, such as those involving NOA proteins, are therapeutically expedient. Elevated levels of reactive oxygen species, as well as spontaneous/therapy-induced DNA damage, ER stress response, and aneuploidy, which are frequently found in HL, cause mTOR adaption to stress responses(Figure 2) (Sen et al., 1990; Bur et al., 2014; Cuceu et al., 2018; Daniel et al., 2019; Alao et al., 2021; Mafi et al., 2023; Marini et al., 2023). Each of these stressors and promoters of mTOR expression/activation correlates with a specific phenotype of cellular stress response (Weigelt et al., 2011). mTORC1 activates stress-responsive transcription factors (Aramburu et al., 2014).
Therefore, the therapeutic potential of mTOR inhibitors is entirely dependent on combinatorial therapeutic strategies while taking into account both stressors related to mTOR induction/activation and the wide range of mTOR effectors (Figure 2). Stress response research revealed that the stressor dose and adaptation time dramatically influence the outcome of in vitro studies (Zelenka et al., 2018). The relationship between mTOR and multiple stressors and effectors characterizes the conserved function of mTOR within stress responses as well as its universal function in a wide range of tumor types, leading to the issue of editing mTOR for strengthening NOA (Figure 3).
Figure 3. Multiple cues of HL stress response modulate and activate mTOR, followed by mTOR-dependent regulation and activation of specific transcription factors, as well as receptor-triggered transcription factors targeted with the MEPED schedule.
The requirements for modulating stressors and effectors of mTOR activity are addressed by the concerted activity profiles of biomodulatory active drugs, as shown in the MEPED schedule (Figure 3) (Ishizuka et al., 1997; Jundt et al., 2005; Georgakis et al., 2006; Rosenwald et al., 2008; Laplante and Sabatini, 2013; Poggi and Zocchi, 2014; Doloff and Waxman, 2015; Kim and Guan, 2015; Gandin et al., 2016; Kewitz et al., 2016; Saxton and Sabatini, 2017; Xia et al., 2018; Matolay et al., 2019; Méhes et al., 2019; Lüke et al., 2021; Weniger and Küppers, 2021; Battaglioni et al., 2022; Kyriazopoulou et al., 2022; Shiau et al., 2022; Mafi et al., 2023; Masel et al., 2023; Reuthner et al., 2024). In contrast, the striking activity profile of MEPED reveals that stress-responsive NOA signaling plays a key role in progressive r/r HL and provides multifold functionally interacting targets for resolving M-CRAC (Harrer et al., 2022; Xu et al., 2024).
Rescue therapies of r/r HL in the second or third line provide major, yet unresolved problems (Brice et al., 2021). To date, no single, currently available therapy approach for r/r HL >second line may be prioritized (Hazane Leroyer et al., 2022).
Noteworthy developments in targeting oncogenic events in HL, such as using ICPIs, are fraught with difficulties in sufficiently drugging stress response pathways and reprograming HL hallmarks, as systemic pulsed HL therapy may promote M-CRAC via apoptosis induction in r/r HL (Harrer et al., 2022). Instead, the NOA proteome provides a decisive unique modular therapeutic infrastructure to definitely overcome M-CRAC if an HL tissue editing technique is applied.
Tumor tissue editing, along with the MEPED regimen, represents a novel multimodal bioregulatory therapy to treat r/r HL. Targets are NOA mechanisms. The system-directed therapeutic strategy demonstrates potential in achieving complete remission in challenging clinical situations, such as third- to sixth-line therapy.
The presented study results on the metronomic combined application of pioglitazone, dexamethasone, everolimus, etoricoxib, and treosulfan, tested in heavily pre-treated patients, and the resulting high rate of CRs (six of seven patients) and seven cCRs made us to study the mechanistic activity profile of everolimus using available clinical data on everolimus in r/r HL and the huge amount of experimental data on stress responses and mTOR activity in HL (Figure 3). The exploration provides a detailed explanation of how a multimodal HL tissue editing therapy targets r/r HL cells and the adjacent microenvironment, highlighting mTOR as a critical therapeutic bottleneck due to concerted transcription modulation and MEPED as a regulator of HL stress responses and HL hallmarks.
The pilot study on MEPED requires definitive validation in a larger multicenter trial because it lacks comprehensive statistical power and raises potential concerns, particularly whether the results may be generalized across all HL subgroups and whether responders may be selected via molecular profiling. Long-term sustainability of complete remission in r/r HL (seven patients) stimulates to proceed with further validating studies (Table 2).
The MEPED approach represents a promising therapeutic strategy for r/r HL, with significant potential in challenging clinical scenarios. The research requires, however, further investigation to establish its definitive clinical utility. If validated, this approach could provide a less toxic, more targeted treatment option for patients with advanced r/r HL, thereby improving both survival and quality of life.
DCH: Conceptualization, Investigation, Writing–original draft, Writing–review and editing. FL: Writing–original draft, Writing–review and editing. TP: Writing–original draft, Writing–review and editing. LG: Writing–original draft, Writing–review and editing. AR: Conceptualization, Investigation, Writing–original draft, Writing–review and editing. DH: Conceptualization, Investigation, Writing–original draft, Writing–review and editing.
The author(s) declare that no financial support was received for the research, authorship, and/or publication of this article.
The authors declare that the research was conducted in the absence of any commercial or financial relationships that could be construed as a potential conflict of interest.
The author(s) declared that they were an editorial board member of Frontiers, at the time of submission. This had no impact on the peer review process and the final decision.
The author(s) declare that no Generative AI was used in the creation of this manuscript.
All claims expressed in this article are solely those of the authors and do not necessarily represent those of their affiliated organizations, or those of the publisher, the editors and the reviewers. Any product that may be evaluated in this article, or claim that may be made by its manufacturer, is not guaranteed or endorsed by the publisher.
Alao, J.-P., Legon, L., and Rallis, C. (2021). Crosstalk between the mTOR and DNA damage response pathways in fission yeast. Cells 10, 305. doi:10.3390/cells10020305
Alig, S. K., Shahrokh Esfahani, M., Garofalo, A., Li, M. Y., Rossi, C., Flerlage, T., et al. (2024). Distinct Hodgkin lymphoma subtypes defined by noninvasive genomic profiling. Nature 625, 778–787. doi:10.1038/s41586-023-06903-x
Álvarez-Garcia, V., Tawil, Y., Wise, H. M., and Leslie, N. R. (2019). Mechanisms of PTEN loss in cancer: it's all about diversity. Semin. Cancer Biol. 59, 66–79. doi:10.1016/j.semcancer.2019.02.001
Ansell, S. M. (2020). Hodgkin lymphoma: a 2020 update on diagnosis, risk-stratification, and management. Am. J. Hematol. 95, 978–989. doi:10.1002/ajh.25856
Aramburu, J., Ortells, M. C., Tejedor, S., Buxadé, M., and López-Rodríguez, C. (2014). Transcriptional regulation of the stress response by mTOR. Sci. Signal 7, re2. doi:10.1126/scisignal.2005326
Battaglioni, S., Benjamin, D., Wälchli, M., Maier, T., and Hall, M. N. (2022). mTOR substrate phosphorylation in growth control. Cell 185, 1814–1836. doi:10.1016/j.cell.2022.04.013
Brice, P., Kerviler, E. de, and Friedberg, J. W. (2021). Classical Hodgkin lymphoma. Lancet 398, 1518–1527. doi:10.1016/S0140-6736(20)32207-8
Bröckelmann, P. J., Bühnen, I., Meissner, J., Trautmann-Grill, K., Herhaus, P., Halbsguth, T. V., et al. (2023). Nivolumab and doxorubicin, vinblastine, and dacarbazine in early-stage unfavorable Hodgkin lymphoma: final analysis of the randomized German Hodgkin study group phase II NIVAHL trial. J. Clin. Oncol. 41, 1193–1199. doi:10.1200/JCO.22.02355
Bur, H., Haapasaari, K.-M., Turpeenniemi-Hujanen, T., Kuittinen, O., Auvinen, P., Marin, K., et al. (2014). Oxidative stress markers and mitochondrial antioxidant enzyme expression are increased in aggressive Hodgkin lymphomas. Histopathology 65, 319–327. doi:10.1111/his.12389
Cader, F. Z., Schackmann, R. C. J., Hu, X., Wienand, K., Redd, R., Chapuy, B., et al. (2018). Mass cytometry of Hodgkin lymphoma reveals a CD4+ regulatory T-cell-rich and exhausted T-effector microenvironment. Blood 132, 825–836. doi:10.1182/blood-2018-04-843714
Cellini, A., Scarmozzino, F., Angotzi, F., Ruggeri, E., Dei Tos, A. P., Trentin, L., et al. (2023). Tackling the dysregulated immune-checkpoints in classical Hodgkin lymphoma: bidirectional regulations between the microenvironment and Hodgkin/Reed-Sternberg cells. Front. Oncol. 13, 1203470. doi:10.3389/fonc.2023.1203470
Chan, J. S. K., Sng, M. K., Teo, Z. Q., Chong, H. C., Twang, J. S., and Tan, N. S. (2018). Targeting nuclear receptors in cancer-associated fibroblasts as concurrent therapy to inhibit development of chemoresistant tumors. Oncogene 37, 160–173. doi:10.1038/onc.2017.319
Chang, H. R., Jung, E., Cho, S., Jeon, Y.-J., and Kim, Y. (2021). Targeting non-oncogene addiction for cancer therapy. Biomolecules 11, 129. doi:10.3390/biom11020129
Chen, R., Zinzani, P. L., Lee, H. J., Armand, P., Johnson, N. A., Brice, P., et al. (2019). Pembrolizumab in relapsed or refractory Hodgkin lymphoma: 2-year follow-up of KEYNOTE-087. Blood 134, 1144–1153. doi:10.1182/blood.2019000324
Connors, J. M., Cozen, W., Steidl, C., Carbone, A., Hoppe, R. T., Flechtner, H.-H., et al. (2020). Hodgkin lymphoma. Nat. Rev. Dis. Prim. 6, 61. doi:10.1038/s41572-020-0189-6
Cuceu, C., Hempel, W. M., Sabatier, L., Bosq, J., Carde, P., and M'kacher, R. (2018). Chromosomal instability in Hodgkin lymphoma: an in-depth review and perspectives. Cancers (Basel) 10, 91. doi:10.3390/cancers10040091
Cuéllar Mendoza, M. E., Chávez Sánchez, F. R., Dorantes Acosta, E. M., Niembro Zúñiga, A. M., Pelayo, R., and Zapata Tarrés, M. (2024). Not only a therapeutic target; mTOR in Hodgkin lymphoma and acute lymphoblastic leukemia. Front. Oncol. 14, 1304605. doi:10.3389/fonc.2024.1304605
Daniel, V., Delamain, M. T., Murbach, B. d. A., Souza, C. A. de, Lima, C. S. P., and Lourenço, G. J. (2019). Role for DNA base-excision repair gene variants in the prognosis of Hodgkin lymphoma. Br. J. Haematol. 186, 171–175. doi:10.1111/bjh.15779
Doloff, J. C., and Waxman, D. J. (2015). Transcriptional profiling provides insights into metronomic cyclophosphamide-activated, innate immune-dependent regression of brain tumor xenografts. BMC Cancer 15, 375. doi:10.1186/s12885-015-1358-y
Dossou, A. S., and Basu, A. (2019). The emerging roles of mTORC1 in macromanaging autophagy. Cancers (Basel) 11, 1422. doi:10.3390/cancers11101422
Dürkop, H., Latza, U., Hummel, M., Eitelbach, F., Seed, B., and Stein, H. (1992). Molecular cloning and expression of a new member of the nerve growth factor receptor family that is characteristic for Hodgkin's disease. Cell 68, 421–427. doi:10.1016/0092-8674(92)90180-K
Esmaeili, S., Safaroghli-Azar, A., Pourbagheri-Sigaroodi, A., Salari, S., Gharehbaghian, A., Hamidpour, M., et al. (2021). Stimulation of peroxisome proliferator-activated receptor-gamma (PPARγ) using pioglitazone decreases the survival of acute promyelocytic leukemia cells through up-regulation of PTEN expression. Anticancer Agents Med. Chem. 21, 108–119. doi:10.2174/1871520620666200817101533
Fehniger, T. A., Watkins, M. P., Ezenwajiaku, N., Wan, F., Hurd, D. D., Cashen, A. F., et al. (2024). A phase II study of interrupted and continuous dose lenalidomide in relapsed/refractory Hodgkin lymphoma. Haematologica. 109, 953–957. doi:10.3324/haematol.2022.282246
Fichtner, M. L., Jiang, R., Bourke, A., Nowak, R. J., and O'Connor, K. C. (2020). Autoimmune pathology in myasthenia gravis disease subtypes is governed by divergent mechanisms of immunopathology. Front. Immunol. 11, 776. doi:10.3389/fimmu.2020.00776
Fornecker, L.-M., Lazarovici, J., Aurer, I., Casasnovas, R.-O., Gac, A.-C., Bonnet, C., et al. (2023). Brentuximab vedotin plus avd for first-line treatment of early-stage unfavorable Hodgkin lymphoma (breach): a multicenter, open-label, randomized, phase II trial. J. Clin. Oncol. 41, 327–335. doi:10.1200/JCO.21.01281
Gandin, V., Masvidal, L., Cargnello, M., Gyenis, L., McLaughlan, S., Cai, Y., et al. (2016). mTORC1 and CK2 coordinate ternary and eIF4F complex assembly. Nat. Commun. 7, 11127. doi:10.1038/ncomms11127
Georgakis, G. V., Li, Y., Rassidakis, G. Z., Medeiros, L. J., Mills, G. B., and Younes, A. (2006). Inhibition of the phosphatidylinositol-3 kinase/Akt promotes G1 cell cycle arrest and apoptosis in Hodgkin lymphoma. Br. J. Haematol. 132, 503–511. doi:10.1111/j.1365-2141.2005.05881.x
Gillessen, S., Hüttmann, A., Vucinic, V., Müller, H., Plütschow, A., Viardot, A., et al. (2022). Reinduction therapy with everolimus in combination with dexamethasone, high-dose cytarabin and cisplatinum in patients with relapsed or refractory classical Hodgkin lymphoma: an experimental phase I/II multicentre trial of the German Hodgkin Study Group (GHSG HD-R3i). Haematol. 196, 606–616. doi:10.1111/bjh.17878
Glaviano, A., Foo, A. S. C., Lam, H. Y., Yap, K. C. H., Jacot, W., Jones, R. H., et al. (2023). PI3K/AKT/mTOR signaling transduction pathway and targeted therapies in cancer. Mol. Cancer 22, 138. doi:10.1186/s12943-023-01827-6
Gordon, S. R., Maute, R. L., Dulken, B. W., Hutter, G., George, B. M., McCracken, M. N., et al. (2017). PD-1 expression by tumour-associated macrophages inhibits phagocytosis and tumour immunity. Nature 545, 495–499. doi:10.1038/nature22396
Grover, N. S., Dittus, C., Thakkar, A., and Beaven, A. W. (2023). The optimal management of relapsed and refractory Hodgkin lymphoma: post-brentuximab and checkpoint inhibitor failure. Hematol. Am. Soc. Hematol. Educ. Program 2023, 510–518. doi:10.1182/hematology.2023000450
Harrer, D. C., Jakob, M., Vogelhuber, M., Lüke, F., Utpatel, K., Corbacioglu, S., et al. (2022). Biomodulatory therapy induces durable remissions in multi-system Langerhans cell histiocytosis. Leuk. Lymphoma 63, 2858–2868. doi:10.1080/10428194.2022.2095627
Harrer, D. C., Lüke, F., Pukrop, T., Ghibelli, L., Gerner, C., Reichle, A., et al. (2023a). Peroxisome proliferator-activated receptorα/γ agonist pioglitazone for rescuing relapsed or refractory neoplasias by unlocking phenotypic plasticity. Front. Oncol. 13, 1289222. doi:10.3389/fonc.2023.1289222
Harrer, D. C., Lüke, F., Pukrop, T., Ghibelli, L., Reichle, A., and Heudobler, D. (2023b). Addressing genetic tumor heterogeneity, post-therapy metastatic spread, cancer repopulation, and development of acquired tumor cell resistance. Cancers (Basel) 16, 180. doi:10.3390/cancers16010180
Hazane Leroyer, E., Ziegler, C., Moulin, C., Campidelli, A., Jacquet, C., Rubio, M. T., et al. (2022). Filling the gap: the immune therapeutic armamentarium for relapsed/refractory Hodgkin lymphoma. J. Clin. Med. 11, 6574. doi:10.3390/jcm11216574
Herrera, A. F., Chen, L., Nieto, Y., Holmberg, L., Johnston, P., Mei, M., et al. (2023). Brentuximab vedotin plus nivolumab after autologous haematopoietic stem-cell transplantation for adult patients with high-risk classic Hodgkin lymphoma: a multicentre, phase 2 trial. Lancet Haematol. 10, e14–e23. doi:10.1016/S2352-3026(22)00318-0
Heudobler, D., Rechenmacher, M., Lüke, F., Vogelhuber, M., Pukrop, T., Herr, W., et al. (2018). Peroxisome proliferator-activated receptors (PPAR)γ agonists as master modulators of tumor tissue. Int. J. Mol. Sci. 19, 3540. doi:10.3390/ijms19113540
Hua, H., Kong, Q., Zhang, H., Wang, J., Luo, T., and Jiang, Y. (2019). Targeting mTOR for cancer therapy. J. Hematol. Oncol. 12, 71. doi:10.1186/s13045-019-0754-1
Huang, J., Pang, W. S., Lok, V., Zhang, L., Lucero-Prisno, D. E., Xu, W., et al. (2022). Incidence, mortality, risk factors, and trends for Hodgkin lymphoma: a global data analysis. J. Hematol. Oncol. 15, 57. doi:10.1186/s13045-022-01281-9
Ishizuka, T., Sakata, N., Johnson, G. L., Gelfand, E. W., and Terada, N. (1997). Rapamycin potentiates dexamethasone-induced apoptosis and inhibits JNK activity in lymphoblastoid cells. Biochem. Biophys. Res. Commun. 230, 386–391. doi:10.1006/bbrc.1996.5967
Janku, F., Park, H., Call, S. G., Madwani, K., Oki, Y., Subbiah, V., et al. (2022). Safety and efficacy of vorinostat plus sirolimus or everolimus in patients with relapsed refractory Hodgkin lymphoma. Clin. Cancer Res. 26, 5579–5587. doi:10.1158/1078-0432.CCR-20-1215
Johnston, B. P., Inwards, D. J., Colgan, J. P., Laplant, B. R., Kabat, B. F., Habermann, T. M., et al. (2010). A phase II trial of the oral mTOR inhibitor everolimus in relapsed Hodgkin lymphoma. Am. J. Hematol. 85, 320–324. doi:10.1002/ajh.21664
Johnston, B. P., Pinter-Brown, L. C., Ghulam Warsi, G., Kristen White, K., and Ramchandren, R. (2018). Phase 2 study of everolimus for relapsed or refractory classical Hodgkin lymphoma. Exp. Hematol. Oncol. 11, 7–12. doi:10.1186/s40164-018-0103-z
Johnson, P. W. M. (2023). Are we reaching the maximum cure rate for Hodgkin lymphoma? Hematol. Oncol. 41 (Suppl. 1), 57–61. doi:10.1002/hon.3140
Jundt, F., Raetzel, N., Müller, C., Calkhoven, C. F., Kley, K., Mathas, S., et al. (2005). A rapamycin derivative (everolimus) controls proliferation through down-regulation of truncated CCAAT enhancer binding protein {beta} and NF-{kappa}B activity in Hodgkin and anaplastic large cell lymphomas. Blood 106, 1801–1807. doi:10.1182/blood-2004-11-4513
Karihtala, K., Leivonen, S.-K., Karjalainen-Lindsberg, M.-L., Chan, F. C., Steidl, C., Pellinen, T., et al. (2022). Checkpoint protein expression in the tumor microenvironment defines the outcome of classical Hodgkin lymphoma patients. Blood Adv. 6, 1919–1931. doi:10.1182/bloodadvances.2021006189
Kelly, K. M., and Friedberg, J. W. (2024). Classic Hodgkin lymphoma in adolescents and young adults. J. Clin. Oncol. 42, 653–664. doi:10.1200/JCO.23.01799
Kewitz, S., Kurch, L., Volkmer, I., and Staege, M. S. (2016). Stimulation of the hypoxia pathway modulates chemotherapy resistance in Hodgkin's lymphoma cells. Tumour Biol. 37, 8229–8237. doi:10.1007/s13277-015-4705-3
Kim, Y. C., and Guan, K.-L. (2015). mTOR: a pharmacologic target for autophagy regulation. J. Clin. Invest 125, 25–32. doi:10.1172/JCI73939
Kirchner, G. I., Meier-Wiedenbach, I., and Manns, M. P. (2004). Clinical pharmacokinetics of everolimus. Clin. Pharmacokinet. 43, 83–95. doi:10.2165/00003088-200443020-00002
Konopleva, M., Elstner, E., McQueen, T. J., Tsao, T., Sudarikov, A., Hu, W., et al. (2004). Peroxisome proliferator-activated receptor gamma and retinoid X receptor ligands are potent inducers of differentiation and apoptosis in leukemias. Mol. Cancer Ther. 3, 1249–1262. doi:10.1158/1535-7163.1249.3.10
Kuruvilla, J., Ramchandren, R., Santoro, A., Paszkiewicz-Kozik, E., Gasiorowski, R., Johnson, N. A., et al. (2021). Pembrolizumab versus brentuximab vedotin in relapsed or refractory classical Hodgkin lymphoma (KEYNOTE-204): an interim analysis of a multicentre, randomised, open-label, phase 3 study. Lancet Oncol. 22, 512–524. doi:10.1016/S1470-2045(21)00005-X
Kyriazopoulou, L., Karpathiou, G., Hatzimichael, E., Peoc'h, M., Papoudou-Bai, A., and Kanavaros, P. (2022). Autophagy and cellular senescence in classical Hodgkin lymphoma. Pathol. Res. Pract. 236, 153964. doi:10.1016/j.prp.2022.153964
Laplante, M., and Sabatini, D. M. (2013). Regulation of mTORC1 and its impact on gene expression at a glance. J. Cell Sci. 126, 1713–1719. doi:10.1242/jcs.125773
Lüke, F., Harrer, D. C., Menhart, K., Wolff, D., Holler, E., Hellwig, D., et al. (2021). Biomodulatory treatment regimen, MEPED, rescues relapsed and refractory classic hodgkin's disease. Front. Pharmacol. 12, 599561. doi:10.3389/fphar.2021.599561
Luo, J., Solimini, N. L., and Elledge, S. J. (2009). Principles of cancer therapy: oncogene and non-oncogene addiction. Cell 136, 823–837. doi:10.1016/j.cell.2009.02.024
Major, A., Kline, J., Karrison, T. G., Fishkin, P. A. S., Kimball, A. S., Petrich, A. M., et al. (2024). Phase I/II clinical trial of temsirolimus and lenalidomide in patients with relapsed and refractory lymphomas. Haematologica. 107, 1608–1618. doi:10.3324/haematol.2021.278853
Mafi, S., Ahmadi, E., Meehan, E., Chiari, C., Mansoori, B., Sadeghi, H., et al. (2023). The mTOR signaling pathway interacts with the ER stress response and the unfolded protein response in cancer. Cancer Res. 83, 2450–2460. doi:10.1158/0008-5472.CAN-22-3032
Marini, C., Cossu, V., Lanfranchi, F., Carta, S., Vitale, F., D'Amico, F., et al. (2023). Divergent oxidative stress in normal tissues and inflammatory cells in Hodgkin and non-hodgkin lymphoma. Cancers (Basel) 15, 3533. doi:10.3390/cancers15133533
Márk, Á., Hajdu, M., Váradi, Z., Sticz, T. B., Nagy, N., Csomor, J., et al. (2013). Characteristic mTOR activity in Hodgkin-lymphomas offers a potential therapeutic target in high risk disease--a combined tissue microarray, in vitro and in vivo study. BMC Cancer 13, 250. doi:10.1186/1471-2407-13-250
Masel, R., Roche, M. E., and Martinez-Outschoorn, U. (2023). Hodgkin Lymphoma: a disease shaped by the tumor micro- and macroenvironment. Best. Pract. Res. Clin. Haematol. 36, 101514. doi:10.1016/j.beha.2023.101514
Matolay, O., Beke, L., Gyurkovics, A., Francz, M., Varjasi, G., Rejtő, L., et al. (2019). Quantitative analysis of carbonic anhydrase IX uncovers hypoxia-related functional differences in classical Hodgkin lymphoma subtypes. Int. J. Mol. Sci. 20, 3463. doi:10.3390/ijms20143463
Méhes, G., Matolay, O., Beke, L., Czenke, M., Jóna, Á., Miltényi, Z., et al. (2019). Hypoxia-related carbonic anhydrase IX expression is associated with unfavourable response to first-line therapy in classical Hodgkin's lymphoma. Histopathology 74, 699–708. doi:10.1111/his.13808
Mehta-Shah, N., and Bartlett, N. L. (2018). Management of relapsed/refractory classical Hodgkin lymphoma in transplant-ineligible patients. Blood 131, 1698–1703. doi:10.1182/blood-2017-09-772681
Moskowitz, A. J., Shah, G., Schöder, H., Ganesan, N., Drill, E., Hancock, H., et al. (2021). Phase II trial of pembrolizumab plus gemcitabine, vinorelbine, and liposomal doxorubicin as second-line therapy for relapsed or refractory classical Hodgkin lymphoma. J. Clin. Oncol. 39, 3109–3117. doi:10.1200/JCO.21.01056
Mossmann, D., Park, S., and Hall, M. N. (2018). mTOR signalling and cellular metabolism are mutual determinants in cancer. Nat. Rev. Cancer 18, 744–757. doi:10.1038/s41568-018-0074-8
Padrnos, L., Ernst, B., Dueck, A. C., Kosiorek, H. E., Ginos, B. F., Toro, A., et al. (2018). A novel combination of the mTORC1 inhibitor everolimus and the immunomodulatory drug lenalidomide produces durable responses in patients with heavily pretreated relapsed lymphoma. Clin. Lymphoma Myeloma Leuk. 18, 664–672. doi:10.1016/j.clml.2018.06.013
Patel, L., Pass, I., Coxon, P., Downes, C. P., Smith, S. A., and Macphee, C. H. (2001). Tumor suppressor and anti-inflammatory actions of PPARgamma agonists are mediated via upregulation of PTEN. Curr. Biol. 11, 764–768. doi:10.1016/s0960-9822(01)00225-1
Pierdominici, M., Maselli, A., Locatelli, S. L., Ciarlo, L., Careddu, G., Patrizio, M., et al. (2017). Estrogen receptor β ligation inhibits Hodgkin lymphoma growth by inducing autophagy. Oncotarget 8, 8522–8535. doi:10.18632/oncotarget.14338
Poggi, A., and Zocchi, M. R. (2014). Stress immunity in lymphomas: mesenchymal cells as a target of therapy. Front. Biosci. (Landmark Ed.) 19, 281–290. doi:10.2741/4207
Pophali, P., Varela, J. C., and Rosenblatt, J. (2024). Immune checkpoint blockade in hematological malignancies: current state and future potential. Front. Oncol. 14, 1323914. doi:10.3389/fonc.2024.1323914
Randall, M. P., and Spinner, M. A. (2023). Optimizing treatment for relapsed/refractory classic Hodgkin lymphoma in the era of immunotherapy. Cancers (Basel) 15, 4509. doi:10.3390/cancers15184509
Rashid, M., Zadeh, L. R., Baradaran, B., Molavi, O., Ghesmati, Z., Sabzichi, M., et al. (2021). Up-down regulation of HIF-1α in cancer progression. Gene 798, 145796. doi:10.1016/j.gene.2021.145796
Reuthner, K., Aubele, P., Menhart, K., Rath, P., Harrer, D. C., Herr, W., et al. (2024). Case report: sustained complete remission with all-oral MEPED therapy in a patient with Hodgkin's disease developing resistance to pembrolizumab. Front. Pharmacol. 15, 1334233. doi:10.3389/fphar.2024.1334233
Ribatti, D., Tamma, R., Annese, T., Ingravallo, G., and Specchia, G. (2022). Inflammatory microenvironment in classical Hodgkin's lymphoma with special stress on mast cells. Front. Oncol. 12, 964573. doi:10.3389/fonc.2022.964573
Rosenwald, I. B., Koifman, L., Savas, L., Chen, J.-J., Woda, B. A., and Kadin, M. E. (2008). Expression of the translation initiation factors eIF-4E and eIF-2* is frequently increased in neoplastic cells of Hodgkin lymphoma. Hum. Pathol. 39, 910–916. doi:10.1016/j.humpath.2007.10.021
Saxton, R. A., and Sabatini, D. M. (2017). mTOR signaling in growth, metabolism, and disease. Cell 168, 960–976. doi:10.1016/j.cell.2017.02.004
Sen, P., Bailey, N. M., Hagemeister, F. B., and Liang, J. C. (1990). Induction of chromosome breaks and sister chromatid exchanges in patients with Hodgkin's disease by two combination chemotherapy regimens of different leukemogenic potential. Cancer Res. 50, 558–562.
Shiau, J.-P., Chuang, Y.-T., Cheng, Y.-B., Tang, J.-Y., Hou, M.-F., Yen, C.-Y., et al. (2022). Impacts of oxidative stress and PI3K/AKT/mTOR on metabolism and the future direction of investigating fucoidan-modulated metabolism. Antioxidants (Basel) 11, 911. doi:10.3390/antiox11050911
Solimini, N. L., Luo, J., and Elledge, S. J. (2007). Non-oncogene addiction and the stress phenotype of cancer cells. Cell 130, 986–988. doi:10.1016/j.cell.2007.09.007
Spinner, M. A., Sica, R. A., Tamaresis, J. S., Lu, Y., Chang, C., Lowsky, R., et al. (2023). Improved outcomes for relapsed/refractory Hodgkin lymphoma after autologous transplantation in the era of novel agents. Blood 141, 2727–2737. doi:10.1182/blood.2022018827
Steidl, C., Connors, J. M., and Gascoyne, R. D. (2011). Molecular pathogenesis of Hodgkin's lymphoma: increasing evidence of the importance of the microenvironment. J. Clin. Oncol. 29, 1812–1826. doi:10.1200/JCO.2010.32.8401
Sureda, A., Canals, C., Arranz, R., Caballero, D., Ribera, J. M., Brune, M., et al. (2012). Allogeneic stem cell transplantation after reduced intensity conditioning in patients with relapsed or refractory Hodgkin's lymphoma. Results of the HDR-ALLO study - a prospective clinical trial by the Grupo Español de Linfomas/Trasplante de Médula Osea (GEL/TAMO) and the Lymphoma Working Party of the European Group for Blood and Marrow Transplantation. Haematologica 97, 310–317. doi:10.3324/haematol.2011.045757
Teresi, R. E., and Waite, K. A. (2008). PPARgamma, PTEN, and the fight against cancer. PPAR Res. 2008, 932632. doi:10.1155/2008/932632
Ugocsai, P., Wolff, D., Menhart, K., Hellwig, D., Holler, E., Herr, W., et al. (2016). Biomodulatory metronomic therapy induces PET-negative remission in chemo- and brentuximab-refractory Hodgkin lymphoma. Br. J. Haematol. 172, 290–293. doi:10.1111/bjh.13480
Vallée, A., and Lecarpentier, Y. (2018). Crosstalk between peroxisome proliferator-activated receptor gamma and the canonical WNT/β-Catenin pathway in chronic inflammation and oxidative stress during carcinogenesis. Front. Immunol. 9, 745. doi:10.3389/fimmu.2018.00745
Weigelt, B., Warne, P. H., and Downward, J. (2011). PIK3CA mutation, but not PTEN loss of function, determines the sensitivity of breast cancer cells to mTOR inhibitory drugs. Oncogene 30, 3222–3233. doi:10.1038/onc.2011.42
Weniger, M. A., and Küppers, R. (2021). Molecular biology of Hodgkin lymphoma. Leukemia 35, 968–981. doi:10.1038/s41375-021-01204-6
Xia, B., Wu, D. W., Wang, T. T., Guo, S. Q., Wang, Y., Yang, H. L., et al. (2018). Expressions and prognostic significance of PTEN and PD-1 protein in patients with classical Hodgkin's lymphoma. Zhonghua Xue Ye Xue Za Zhi 39, 839–844. doi:10.3760/cma.j.issn.0253-2727.2018.10.010
Xu, D., Liang, S.-Q., Su, M., Yang, H., Bruggmann, R., Oberhaensli, S., et al. (2024). Crispr-mediated genome editing reveals a preponderance of non-oncogene addictions as targetable vulnerabilities in pleural mesothelioma. Lung Cancer 197, 107986. doi:10.1016/j.lungcan.2024.107986
Ying, J., Srivastava, G., Hsieh, W.-S., Gao, Z., Murray, P., Liao, S.-K., et al. (2005). The stress-responsive gene GADD45G is a functional tumor suppressor, with its response to environmental stresses frequently disrupted epigenetically in multiple tumors. Clin. Cancer Res. 11, 6442–6449. doi:10.1158/1078-0432.CCR-05-0267
Keywords: relapsed/refractory Hodgkin’s lymphoma, non-oncogene addiction, mTOR, M-CRAC, Hodgkin’s lymphoma tissue editing, anakoinosis, pioglitazone, dexamethasone
Citation: Harrer DC, Lüke F, Pukrop T, Ghibelli L, Reichle A and Heudobler D (2025) MEPED as salvage therapy for relapsed/refractory Hodgkin’s lymphoma incorporating edited non-oncogene addiction: mTOR as a bottleneck. Front. Pharmacol. 16:1553331. doi: 10.3389/fphar.2025.1553331
Received: 30 December 2024; Accepted: 24 February 2025;
Published: 20 March 2025.
Edited by:
Germain Sotoing Taiwe, University of Buea, CameroonReviewed by:
Tamer A. Addissouky, University of Menoufia, EgyptCopyright © 2025 Harrer, Lüke, Pukrop, Ghibelli, Reichle and Heudobler. This is an open-access article distributed under the terms of the Creative Commons Attribution License (CC BY). The use, distribution or reproduction in other forums is permitted, provided the original author(s) and the copyright owner(s) are credited and that the original publication in this journal is cited, in accordance with accepted academic practice. No use, distribution or reproduction is permitted which does not comply with these terms.
*Correspondence: Albrecht Reichle, YWxicmVjaHQucmVpY2hsZUB1a3IuZGU=
†ORCID: Albrecht Reichle, orcid.org/0000-0002-1821-7887
Disclaimer: All claims expressed in this article are solely those of the authors and do not necessarily represent those of their affiliated organizations, or those of the publisher, the editors and the reviewers. Any product that may be evaluated in this article or claim that may be made by its manufacturer is not guaranteed or endorsed by the publisher.
Research integrity at Frontiers
Learn more about the work of our research integrity team to safeguard the quality of each article we publish.