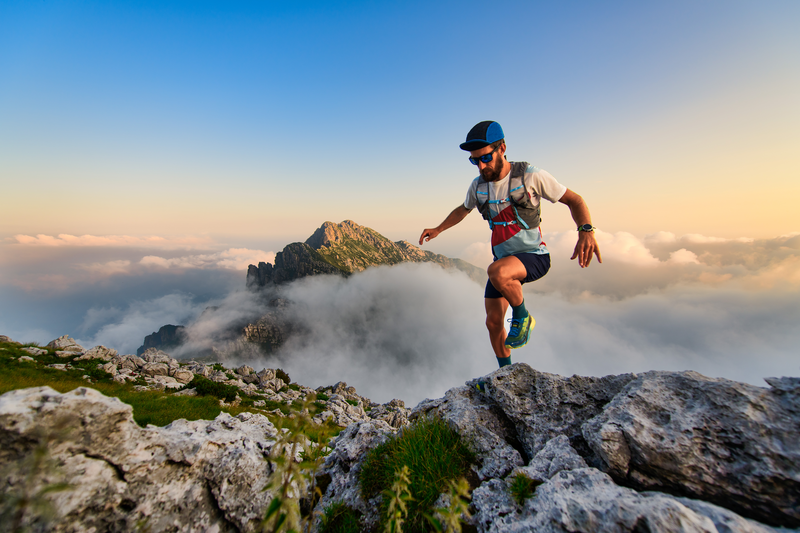
94% of researchers rate our articles as excellent or good
Learn more about the work of our research integrity team to safeguard the quality of each article we publish.
Find out more
ORIGINAL RESEARCH article
Front. Pharmacol.
Sec. Pharmacology of Anti-Cancer Drugs
Volume 16 - 2025 | doi: 10.3389/fphar.2025.1552707
This article is part of the Research Topic Decoding Tumor Drug Resistance: Machine Learning’s Role from Molecules to Treatment View all 4 articles
The final, formatted version of the article will be published soon.
You have multiple emails registered with Frontiers:
Please enter your email address:
If you already have an account, please login
You don't have a Frontiers account ? You can register here
Small molecule inhibitors of vascular endothelial growth factor receptor 2 (VEGFR2) are among the most widely used anti-angiogenic agents for tumor treatment, but their adverse effects have become increasingly apparent. In this study, we investigated the small molecule compound SB218078 to assess its effects on tumor angiogenesis and epithelial-mesenchymal transition (EMT) in breast cancer, employing both in vitro and in vivo models. Initially, we evaluated the impact of SB218078 on human umbilical vein endothelial cells (HUVECs) and found that it significantly inhibited their migration, invasion, and tube formation. Next, we utilized Tg(Fli-1:EGFP) zebrafish embryos to examine the angiogenic landscape in vivo. Treatment with SB218078 disrupted the development of intersegmental vessels (ISV), dorsal longitudinal anastomotic vessels, and subintestinal vessels (SIV), leading to impaired tail regeneration in the embryos. We also assessed the effects of SB218078 on breast cancer cells, observing that it effectively reduced proliferation, migration, and invasion across various breast cancer cell lines. Additionally, the administration of SB218078 in xenograft mouse models notably suppressed tumor angiogenesis, which correlated with reduced tumor growth and metastasis. To further investigate the mechanistic pathways involved, we discovered that SB218078 targets Chk1, a downstream factor of the transcription factor ZEB1. Importantly, we established that the downregulation of ZEB1, rather than Snail or Twist, specifically inhibited the development of ISV and SIV in the zebrafish model. In summary, our research identifies SB218078 as a novel anti-angiogenic agent for the treatment of breast cancer, emphasizing its potential as a promising therapeutic option for patients.
Keywords: Angiogenesis, Zeb1, breast cancer, EMT, SB218078
Received: 29 Dec 2024; Accepted: 12 Feb 2025.
Copyright: © 2025 Wu, Xu, Tang, Yu, Li, Jun and Zhang. This is an open-access article distributed under the terms of the Creative Commons Attribution License (CC BY). The use, distribution or reproduction in other forums is permitted, provided the original author(s) or licensor are credited and that the original publication in this journal is cited, in accordance with accepted academic practice. No use, distribution or reproduction is permitted which does not comply with these terms.
* Correspondence:
Xiang Zhang, Department of Endocrinology and Breast Surgery, First Affiliated Hospital of Chongqing Medical University, Chongqing, China
Disclaimer: All claims expressed in this article are solely those of the authors and do not necessarily represent those of their affiliated organizations, or those of the publisher, the editors and the reviewers. Any product that may be evaluated in this article or claim that may be made by its manufacturer is not guaranteed or endorsed by the publisher.
Research integrity at Frontiers
Learn more about the work of our research integrity team to safeguard the quality of each article we publish.