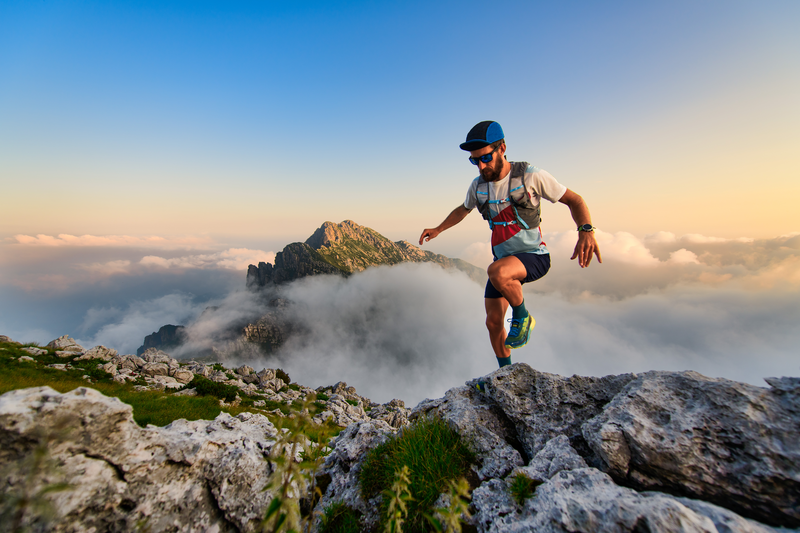
94% of researchers rate our articles as excellent or good
Learn more about the work of our research integrity team to safeguard the quality of each article we publish.
Find out more
ORIGINAL RESEARCH article
Front. Pharmacol.
Sec. Ethnopharmacology
Volume 16 - 2025 | doi: 10.3389/fphar.2025.1551841
This article is part of the Research Topic Herbal Medicine for the Treatment of Chronic Metabolic Diseases, Volume II View all 14 articles
The final, formatted version of the article will be published soon.
You have multiple emails registered with Frontiers:
Please enter your email address:
If you already have an account, please login
You don't have a Frontiers account ? You can register here
This study evaluates the phenolic and flavonoid contents, as well as the antioxidant, antidiabetic, and anti-glycation properties of aqueous and methanolic extracts from Pistacia lentiscus L. leaves. The antioxidant activity was assessed using DPPH, ABTS, FRAP, and iron-chelation assays, revealing superior activity in the aqueous extract. Both extracts exhibited potent antidiabetic effects by inhibiting the digestive enzyme alpha-amylase, with IC50 values of 2,291 ± 0.002 μg/mL (aqueous) and 2,889 ± 0.002 μg/mL (methanolic). Additionally, the extracts demonstrated significant anti-glycation activity, reducing advanced glycation end-product (AGE) formation, inhibiting fructosamine levels, and protecting thiol groups, with the aqueous extract providing greater protection. These findings underscore the potential of Pistacia lentiscus L. as a natural source of bioactive compounds for managing oxidative stress and diabetes-related complications.
Keywords: Pistacia lentiscus L., Diabete mellitus, Oxidative Stress, medical herbes, Phytochemical screening, Antidiabatic activity, Antiglycation activity
Received: 26 Dec 2024; Accepted: 24 Feb 2025.
Copyright: © 2025 EL ALLAOUI, Haboubi, El Ahmadi, Bouhrim, Elabdouni, Eto, Shahat Hussein, Herqash, El Bestrioui, Zouaoui and NHIRI. This is an open-access article distributed under the terms of the Creative Commons Attribution License (CC BY). The use, distribution or reproduction in other forums is permitted, provided the original author(s) or licensor are credited and that the original publication in this journal is cited, in accordance with accepted academic practice. No use, distribution or reproduction is permitted which does not comply with these terms.
* Correspondence:
HASNAE EL ALLAOUI, Laboratory of Engineering Sciences and Applications, National School of Applied Sciences of Al Hoceima. Abdelmalek Essâadi University, Al-Hoceima, Morocco., Al Hoceima, Morocco
Mohamed Bouhrim, Laboratoire Géoressources et Environnement, Faculté des Sciences et Techniques, Université Sultan Moulay Slimane, Beni Mellal, 23000, Morocco
Disclaimer: All claims expressed in this article are solely those of the authors and do not necessarily represent those of their affiliated organizations, or those of the publisher, the editors and the reviewers. Any product that may be evaluated in this article or claim that may be made by its manufacturer is not guaranteed or endorsed by the publisher.
Research integrity at Frontiers
Learn more about the work of our research integrity team to safeguard the quality of each article we publish.