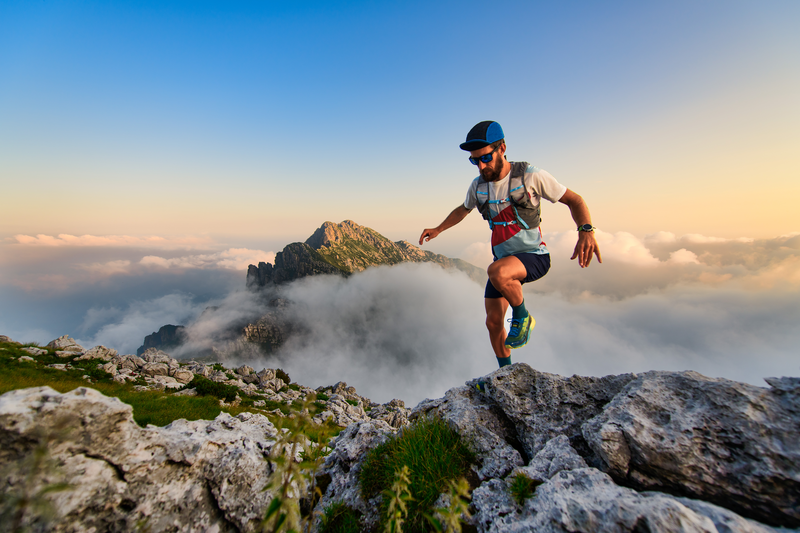
94% of researchers rate our articles as excellent or good
Learn more about the work of our research integrity team to safeguard the quality of each article we publish.
Find out more
MINI REVIEW article
Front. Pharmacol.
Sec. Pharmacology of Anti-Cancer Drugs
Volume 16 - 2025 | doi: 10.3389/fphar.2025.1551219
The final, formatted version of the article will be published soon.
You have multiple emails registered with Frontiers:
Please enter your email address:
If you already have an account, please login
You don't have a Frontiers account ? You can register here
Bladder cancer is a malignant tumor with a high global incidence and recurrence rate. Traditional diagnostic methods, such as cystoscopy and urine cytology, have limitations in sensitivity and specificity, particularly in detecting low-grade bladder cancer. Circulating tumor DNA (ctDNA) offers a non-invasive alternative, reflecting tumor genetic characteristics through blood samples. It demonstrates high sensitivity and repeatability, making it a promising tool for early detection, recurrence monitoring, and treatment evaluation. Clinical studies have shown that ctDNA not only detects tumor burden but also captures dynamic tumor mutations, aiding in personalized treatment strategies. Despite its potential, clinical implementation of ctDNA faces challenges, including optimization of detection techniques, standardization, and the cost of testing. This paper explores the role of ctDNA in advancing bladder cancer diagnosis and treatment, with a focus on refining its clinical application and guiding future research toward improved patient outcomes.
Keywords: Bladder cancer, circulating tumor DNA, Early detection, personalized treatment, liquid biopsy
Received: 25 Dec 2024; Accepted: 25 Feb 2025.
Copyright: © 2025 Zhou, Wang, Zeng and Liu. This is an open-access article distributed under the terms of the Creative Commons Attribution License (CC BY). The use, distribution or reproduction in other forums is permitted, provided the original author(s) or licensor are credited and that the original publication in this journal is cited, in accordance with accepted academic practice. No use, distribution or reproduction is permitted which does not comply with these terms.
* Correspondence:
Mingtang Zeng, West China Hospital, Sichuan University, Chengdu, China
Sijia Liu, West China Hospital, Sichuan University, Chengdu, China
Disclaimer: All claims expressed in this article are solely those of the authors and do not necessarily represent those of their affiliated organizations, or those of the publisher, the editors and the reviewers. Any product that may be evaluated in this article or claim that may be made by its manufacturer is not guaranteed or endorsed by the publisher.
Research integrity at Frontiers
Learn more about the work of our research integrity team to safeguard the quality of each article we publish.