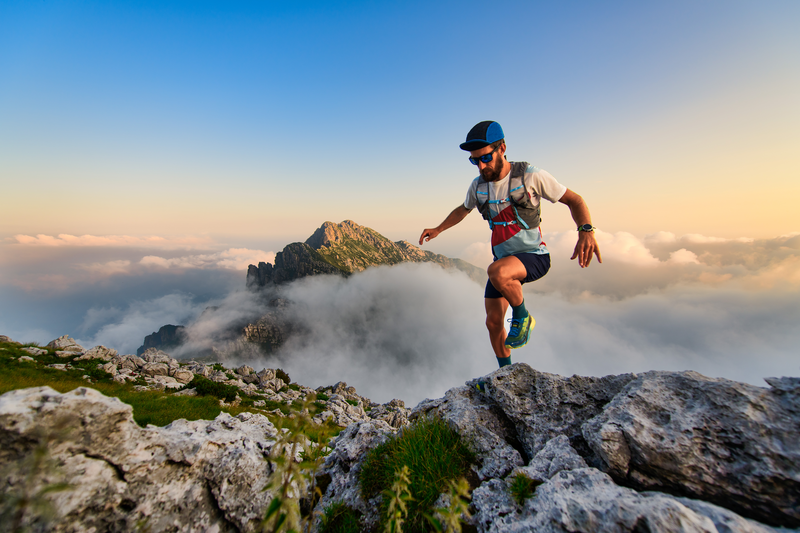
94% of researchers rate our articles as excellent or good
Learn more about the work of our research integrity team to safeguard the quality of each article we publish.
Find out more
ORIGINAL RESEARCH article
Front. Pharmacol.
Sec. Pharmacology of Anti-Cancer Drugs
Volume 16 - 2025 | doi: 10.3389/fphar.2025.1547685
The final, formatted version of the article will be published soon.
You have multiple emails registered with Frontiers:
Please enter your email address:
If you already have an account, please login
You don't have a Frontiers account ? You can register here
In recent years, natural compounds have attracted wide attention for the treatment of liver cancer.Schisandrin B (Sch B) is a major active constituent of Schisandra chinensis. Our experiments showed that Sch B inhibited the growth of hepatocellular carcinoma (HCC). The tumor-killing effect of drugs is influenced by the tumor microenvironment (TME). In the TME of HCC, macrophages interact with HCC cells and affect its development. We constructed a co-culture system between 2 macrophages and HCC cells and determined that Sch B inhibited HCC cell proliferation. Furthermore, Sch B caused cell cycle arrest, downregulated the expression of CDK4, CDK2, and cyclin A2 proteins, upregulated the expression of p27 Kip1 protein, and blocked HCC cells in the S phase. Additionally, Sch B inhibited the migration of HCC cells in a co-culture system. Exosomes are important mediators of cellular interactions in the TME. We further collected exosomes from the co-culture system for identification and performed proteomic analysis, and determined that Sch B downregulated the expression of fibronectin 1 (FN1) protein in exosomes in the co-culture system. In vivo, Sch B inhibited the growth of HCC tumors and downregulated the expression of FN1 protein in tumor tissues. In general, Sch B may inhibit the development of HCC by inhibiting the expression of exosomal FN1during interactions between macrophages and HCC cells.
Keywords: Schisandrin B, Hepatocellular Carcinoma, Exosomes, fibronectin 1, Tumor Microenvironment
Received: 18 Dec 2024; Accepted: 10 Mar 2025.
Copyright: © 2025 Jiang, Yang, Huang, Li, Peng, Gan, Peng, Yao and Qi. This is an open-access article distributed under the terms of the Creative Commons Attribution License (CC BY). The use, distribution or reproduction in other forums is permitted, provided the original author(s) or licensor are credited and that the original publication in this journal is cited, in accordance with accepted academic practice. No use, distribution or reproduction is permitted which does not comply with these terms.
* Correspondence:
Tieli Peng, Division of Gastroenterology, Institute of Digestive Disease, the Affiliated Qingyuan Hospital (Qingyuan People's Hospital), Guangzhou Medical University, Qingyuan, China
Leyi Yao, Zhanjiang Institute of Clinical Medicine,Zhanjiang Central Hospital, Guangdong Medical University, Zhanjiang, 524033, PR China., Zhanjiang, China
Ling Qi, Division of Gastroenterology, Institute of Digestive Disease, the Affiliated Qingyuan Hospital (Qingyuan People's Hospital), Guangzhou Medical University, Qingyuan, China
Disclaimer: All claims expressed in this article are solely those of the authors and do not necessarily represent those of their affiliated organizations, or those of the publisher, the editors and the reviewers. Any product that may be evaluated in this article or claim that may be made by its manufacturer is not guaranteed or endorsed by the publisher.
Research integrity at Frontiers
Learn more about the work of our research integrity team to safeguard the quality of each article we publish.