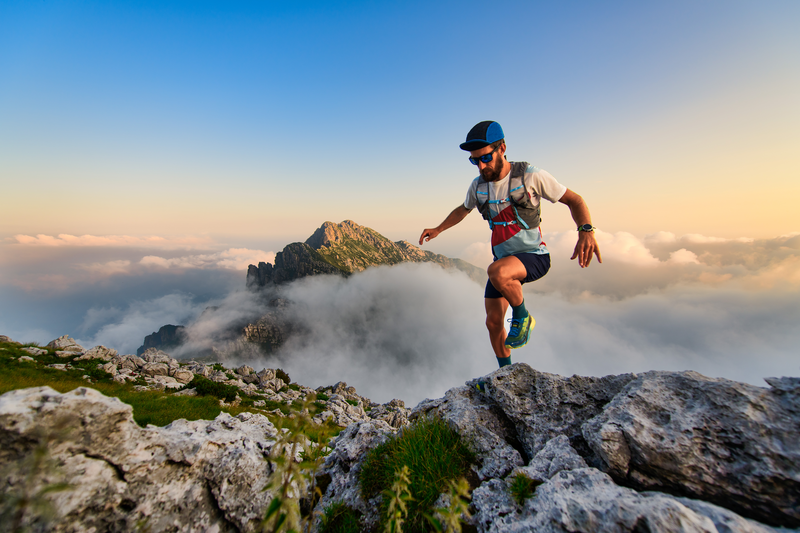
94% of researchers rate our articles as excellent or good
Learn more about the work of our research integrity team to safeguard the quality of each article we publish.
Find out more
ORIGINAL RESEARCH article
Front. Pharmacol.
Sec. Ethnopharmacology
Volume 16 - 2025 | doi: 10.3389/fphar.2025.1547415
This article is part of the Research Topic Emerging Trends in the Quality Check of Herbal Medicines, Supplements and 'Botanicals' View all 6 articles
The final, formatted version of the article will be published soon.
You have multiple emails registered with Frontiers:
Please enter your email address:
If you already have an account, please login
You don't have a Frontiers account ? You can register here
Sendeng-4 is a Mongolia Drug. The Mongolian people have been using it to treat rheumatoid arthritis. At present, More and more Han people pay attention to the anti-rheumatoid effect of Sendeng-4. However, information on the pharmacokinetics of Sendeng-4 is limited, which limits its wide application in China. Objective: Therefore, Liquid chromatography-tandem mass spectrometry (LC-MS/MS) was established to study the pharmacokinetics of Sendeng-4.The MS/MS with negative ionization mode (ESI-) and multiple reaction monitoring at m/z 300.95→193.09 and 317.08→192.10 were detected for (2R,3R)-dihydromyricetin and myricetin, respectively.The pharmacokinetic parameters were analyzed by DAS 2.0. Result: The results showed that the plasma concentration time (C-T) curves of (2R,3R)-dihydromyricetin and myricetin showed double peaks. The Tmax of (2R,3R)-dihydromyricetin and myricetin in both groups was 3h. In the absorption, the AUC(0-∞) of (2R,3R)-dihydromyricetin and myricetin in the Medicine 删除[B.
Keywords: Mongolian drug, Sendeng-4, Myricetin, LC-MS/MS, pharmacokinetic, (2R,3R)-Dihydromyricetin
Received: 18 Dec 2024; Accepted: 21 Feb 2025.
Copyright: © 2025 Bai and Dong. This is an open-access article distributed under the terms of the Creative Commons Attribution License (CC BY). The use, distribution or reproduction in other forums is permitted, provided the original author(s) or licensor are credited and that the original publication in this journal is cited, in accordance with accepted academic practice. No use, distribution or reproduction is permitted which does not comply with these terms.
* Correspondence:
Yu Dong, College of Pharmacy, Inner Mongolia Medical University, Hohhot, Inner Mongolia Autonomous Region, China
Disclaimer: All claims expressed in this article are solely those of the authors and do not necessarily represent those of their affiliated organizations, or those of the publisher, the editors and the reviewers. Any product that may be evaluated in this article or claim that may be made by its manufacturer is not guaranteed or endorsed by the publisher.
Research integrity at Frontiers
Learn more about the work of our research integrity team to safeguard the quality of each article we publish.