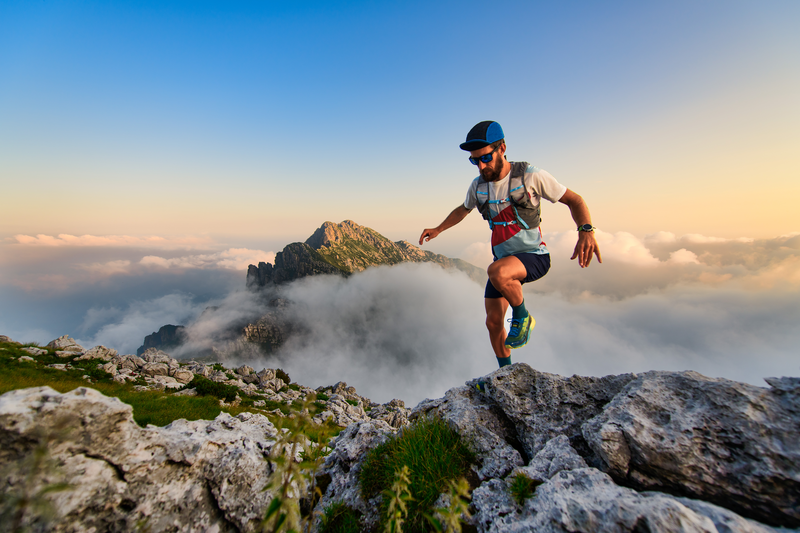
94% of researchers rate our articles as excellent or good
Learn more about the work of our research integrity team to safeguard the quality of each article we publish.
Find out more
ORIGINAL RESEARCH article
Front. Pharmacol.
Sec. Inflammation Pharmacology
Volume 16 - 2025 | doi: 10.3389/fphar.2025.1546785
The final, formatted version of the article will be published soon.
You have multiple emails registered with Frontiers:
Please enter your email address:
If you already have an account, please login
You don't have a Frontiers account ? You can register here
Background: Eosinophilic granulomatosis with polyangiitis (EGPA) is a rare, serious, and intractable chronic disease. Glucocorticoids are the mainstay of treatment for EGPA. The drugs that target interleukin (IL)-5 signaling have also been marketed or developed in recent years. While no animal model can completely recapitulate EGPA, the ovalbumin (OVA)-induced eosinophilic vasculitis mouse model has been reported to exhibits pathological similarities in the lungs. However, the effect of an anti-IL-5 drug has not yet been investigated using this model. This study used the OVA-induced eosinophilic vasculitis model to evaluate and characterize its usefulness, focusing on the effects of an anti-IL-5 antibody.Methods: Female C57BL/6NCrSlc mice were intraperitoneally immunized on days 0 and 14 with OVA adsorbed onto an aluminum gel. From days 26 to 32, the mice were exposed to aerosolized 1% OVA daily for 1 h. Anti-IL-5 antibody or vehicle was injected intravenously or intraperitoneally once on the first day of aerosol exposure (day 26). On day 33, the eosinophil and lymphocyte counts in the blood and bronchoalveolar lavage fluid (BALF) were measured. Lung specimens were used to assess the vascular lesions formed in the pulmonary arteries. Plasma cytokine levels were measured on day 28.The anti-IL-5 antibody significantly reduced eosinophil counts in the blood and BALF. In contrast, it did not inhibit the lymphocyte counts in BALF or vascular lesion formation.
Keywords: EGPA, IL-5, Ovalbumin, Eosinophilic vasculitis, mouse model
Received: 17 Dec 2024; Accepted: 03 Mar 2025.
Copyright: © 2025 Kageyama, Kikuchi and Hoshino. This is an open-access article distributed under the terms of the Creative Commons Attribution License (CC BY). The use, distribution or reproduction in other forums is permitted, provided the original author(s) or licensor are credited and that the original publication in this journal is cited, in accordance with accepted academic practice. No use, distribution or reproduction is permitted which does not comply with these terms.
* Correspondence:
Kiyoto Kageyama, Nippon Shinyaku (Japan), Kyoto, Japan
Disclaimer: All claims expressed in this article are solely those of the authors and do not necessarily represent those of their affiliated organizations, or those of the publisher, the editors and the reviewers. Any product that may be evaluated in this article or claim that may be made by its manufacturer is not guaranteed or endorsed by the publisher.
Research integrity at Frontiers
Learn more about the work of our research integrity team to safeguard the quality of each article we publish.