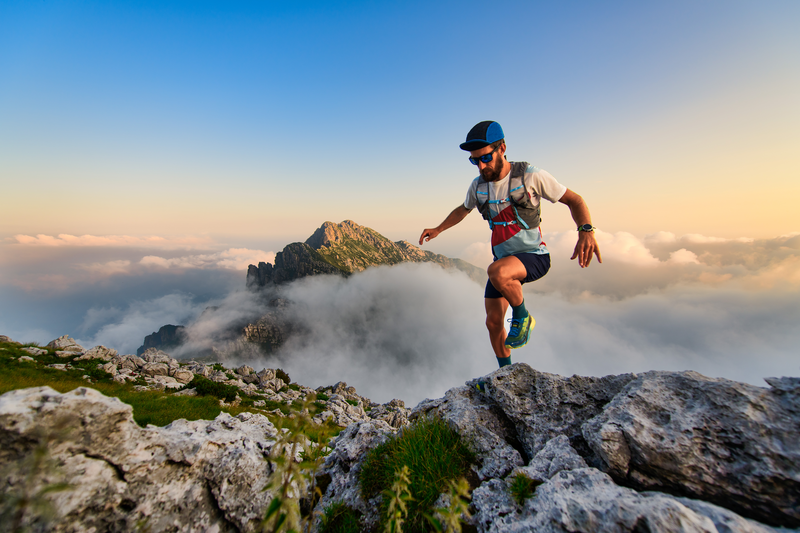
94% of researchers rate our articles as excellent or good
Learn more about the work of our research integrity team to safeguard the quality of each article we publish.
Find out more
ORIGINAL RESEARCH article
Front. Pharmacol.
Sec. Ethnopharmacology
Volume 16 - 2025 | doi: 10.3389/fphar.2025.1545585
This article is part of the Research Topic Traditional, Complementary and Integrative Medicine – Opportunities for Managing and Treating Neurodegenerative Diseases and Ischaemic Stroke View all 3 articles
The final, formatted version of the article will be published soon.
You have multiple emails registered with Frontiers:
Please enter your email address:
If you already have an account, please login
You don't have a Frontiers account ? You can register here
Background: Age-related decline in nicotinamide adenine dinucleotide (NAD+)-a central regulator of cellular metabolism, DNA repair, and immune homeostasis-is strongly associated with physiological dysfunction. Nicotinamide mononucleotide (NMN), a potent NAD+ precursor, shows promise in counteracting aging-related pathologies, particularly neurodegenerative decline. Methods: An aging model was established in mice through 8-week D-galactose (D-gal) exposure, followed by NMN oral supplementation. Behavioral outcomes (open field test, Morris water maze) were analyzed alongside oxidative stress markers (SOD, CAT, AGEs), inflammatory cytokines (TNF-α, IL-1β, IL-6, IL-10), and neurotransmitters (LC-MS/MS). Apoptotic activity (TUNEL, p16/p21), mitochondrial regulators (Sirt1, p-AMPK, PGC-1α), and intestinal barrier integrity (HE/AB-PAS staining) were evaluated. Sirt1 dependency was confirmed using inhibitor Ex527. Results: NMN restored locomotor activity and spatial memory in D-gal mice without altering body weight. Mechanistically, NMN synergistically attenuated oxidative stress and systemic inflammation, elevating antioxidant enzymes (SOD, CAT) and IL-10 while suppressing pro-inflammatory cytokines (TNF-α, IL-6) and AGEs. Cortical/hippocampal analyses revealed reduced apoptosis (TUNEL + cells) and senescence markers (p16, p21), with enhanced mitochondrial function via Sirt1/AMPK/PGC-1α activation (Sirt1, p-AMPK). NMN concurrently preserved intestinal mucosal architecture, mitigating D-gal-induced barrier disruption. Crucially, all benefits were abolished by Sirt1 inhibition, confirming pathway specificity. Conclusion: Our findings establish NMN as a multifaceted therapeutic agent that preserves neurocognitive function and intestinal homeostasis in aging models by orchestrating antioxidative, anti-inflammatory, and antiapoptotic responses through Sirt1/AMPK/PGC-1α activation. This work provides translational insights into NAD+boosting strategies for age-related disorders.
Keywords: Aging, NMN, Oxidative Stress, Neuroinflammation, intestinal barrier, SIRT1
Received: 15 Dec 2024; Accepted: 17 Feb 2025.
Copyright: © 2025 Lin, Wang, Yang, Ding, Hu, Huang, Zhang and Yu. This is an open-access article distributed under the terms of the Creative Commons Attribution License (CC BY). The use, distribution or reproduction in other forums is permitted, provided the original author(s) or licensor are credited and that the original publication in this journal is cited, in accordance with accepted academic practice. No use, distribution or reproduction is permitted which does not comply with these terms.
* Correspondence:
Qichun Zhang, Nanjing University of Chinese Medicine, Nanjing, China
Yingcong Yu, Third Clinical Institute Affiliated to Wenzhou Medical University, Wenzhou, China
Disclaimer: All claims expressed in this article are solely those of the authors and do not necessarily represent those of their affiliated organizations, or those of the publisher, the editors and the reviewers. Any product that may be evaluated in this article or claim that may be made by its manufacturer is not guaranteed or endorsed by the publisher.
Research integrity at Frontiers
Learn more about the work of our research integrity team to safeguard the quality of each article we publish.