- 1Department of Anesthesiology, Union Hospital, Tongji Medical College, Huazhong University of Science and Technology, Wuhan, China
- 2Institute of Anesthesia and Critical Care Medicine, Union Hospital, Tongji Medical College, Huazhong University of Science and Technology, Wuhan, China
- 3Key Laboratory of Anesthesiology and Resuscitation (Huazhong University of Science and Technology), Ministry of Education, Wuhan, China
Cervical cancer is a common malignancy among women, and tumor excision is the most common surgical intervention. Anesthetics used during surgery include general intravenous, volatile, local anesthetics, sedative and analgesic. Studies have shown that the selection of perioperative surgical methods and anesthetics may influence postoperative metastasis and cancer recurrence through their effects on the immune response and tumor cells. Therefore, the selection of perioperative anesthetic has a significant impact on patients undergoing surgery for cervical cancer. This study summarizes the effects and related mechanisms of common anesthetics on the prognosis of patients undergoing surgery for cervical cancer to provide a basis for developing more optimal anesthesia protocols.
1 Introduction
Cervical cancer is the fourth most common malignancy in women worldwide (Bray et al., 2024), and surgical resection is the primary intervention. However, the postoperative recurrence rate of cervical cancer is still very high. Patients undergoing surgery for cancer may experience tumor cell spread to the circulating blood or lymphatic system, or may shed tumor cells during resection. These factors affect postoperative metastasis and the recurrence of cervical cancer. Studies have shown that the selection of perioperative anesthetic has an impact on postoperative recurrence, metastasis, and immune function (Kim, 2018; Ackerman et al., 2021).
Anesthetics include general intravenous, volatile, local anesthetics, sedative and analgesic. Propofol, a widely used intravenous anesthetic, may exert antitumor effects by modulating noncoding RNAs, signaling pathways, and host immune functions (Liu et al., 2021; Liu et al., 2016). There is evidence that volatile anesthetics may also influence cancer recurrence and metastasis by modulating immunity (Wang et al., 2022; Buckley et al., 2014). Long-term exposure with sevoflurane negatively affects estrogen and progesterone regulation (Dogru et al., 2017), and estrogen and progesterone may influence anesthetic dose (Shimizu et al., 2010; Basaran et al., 2019). In addition, studies have shown that estrogen may promote the development of cervical cancer (Baik et al., 2022), while progesterone often inhibits the progression of the disease (Yoo et al., 2013). Nonsteroidal anti-inflammatory drugs (NSAIDs) may have an antitumor effect by exerting anti-inflammatory effects (Saka-Herrán et al., 2021). In addition, local anesthetics may inhibit tumor progression by regulating immunity and inducing apoptosis (Pérez-González et al., 2017; Chen et al., 2022). These findings suggest that perioperative anesthetics may have varying effects on cervical cancer. Therefore, anesthesiologists can improve the prognosis of patients undergoing surgery for cervical cancer by selecting the appropriate anesthetic agents.
The present article reviews the postoperative effects of intravenous, volatile, local anesthetics, sedative and analgesic on patients undergoing surgery for cervical cancer and their underlying mechanisms (Table1).
2 Intravenous anesthetic agents
2.1 Propofol
Available data suggest that propofol can inhibit proliferation and metastasis, and induce apoptosis of malignant tumors (Yu et al., 2020; Liu et al., 2021; Du et al., 2021a; Hu et al., 2022), thereby reducing the risk for cancer recurrence and improving survival, especially in patients undergoing major surgery for cancer (Yap et al., 2019; Sessler and Riedel, 2019). Propofol may affect the development and prognosis of cancer by regulating microRNAs (miRNAs), and modulating signaling pathways and host immune function.
miRNA dysregulation is associated with cancer progression. An in vitro study demonstrated that propofol inhibits the growth and invasion of cervical cancer cells and epithelial-mesenchymal transition by inhibiting miR155HG (Du Y. et al., 2021). The long noncoding RNA (lncRNA) Hox transcript antisense intergenic RNA (HOTAIR) has been reported to be associated with tumor recurrence in cervical cancer (Li et al., 2015; Zhou et al., 2020). Propofol inhibits activation of the mammalian target of rapamycin (mTOR)/p70S6K pathway (Zhang et al., 2015) and modulates the HOTAIR/miR-129-5p/ribosomal protein L14 (RPL14) axis (Sun et al., 2021) by decreasing HOTAIR expression, which subsequently inhibits the growth of cervical cancer cells.
Signaling pathways play an important role in regulating the development and progression of cancer. Researchers have found that the overexpression of epidermal growth factor receptor (EGFR) and signal transducer and activator of transcription (STAT3) are associated with poor prognosis in patients with cervical cancer, and propofol can enhance the antitumor effect of cisplatin on cervical cancer cells by inhibiting the EGFR/Janus tyrosine kinase 2 (JAK2)/STAT3 pathway (Li et al., 2017).In addition, it has been reported that propofol induces ferroptosis by regulating the solute carrier family 7 member 11 (SLC7A11)/glutathione peroxidase 4 (GPX4), ubiquinol/CoQ10/FSP1, and Yes1 associated transcriptional regulator (YAP)/acyl-CoA synthetase long-chain family member 4 (ACSL4)/transferrin receptor (TFRC) pathways, and has a synergistic antitumor effect with paclitaxel (Zhao et al., 2022). Propofol also induces endoplasmic reticulum stress, regulates adenosine monophosphate-activated protein kinase (AMPK)/mTOR signaling, and impairs autophagic flux, thereby inhibiting the growth of HeLa cells (Chen et al., 2018). In addition, inhibition of the Wnt/β-catenin pathway may be the underlying mechanism by which propofol inhibits the growth and migration of cervical cancer cells (Huang et al., 2020a).
Atomic force microscopy has been used to analyze propofol-induced changes in the ultrastructure of the cell membrane of cervical cancer cells. Cervical cancer cells exhibit an evident decrease in membrane roughness, and their lamellipodia significantly retract or disappear after treatment with propofol, which affects cell migration (Zhang et al., 2016).
The antitumor effects of propofol have been verified in animal trials. By generating xenograft tumor and lung metastasis model animals, researchers have found that propofol significantly reduced the level of MIR155HG in tumor tissues and suppressed tumor growth and metastasis of cervical carcinoma cells in vivo (Du et al., 2021a). Additionally, propofol inhibited increases in tumor size in a cervical cancer xenograft model (Zhang et al., 2015; Huang et al., 2020a; Sun et al., 2021).
Patients who experience recurrence and metastasis are usually due to immunosuppression. Natural killer (NK) cells play an important role in controlling tumor metastasis (López-Soto et al., 2017; Lo et al., 2020). A clinical study found that, during the perioperative period in laparoscopic radical procedures in patients with cervical cancer, the number of CD3-positive (+), CD4+, and NK cells, and the ratio of CD4+/CD8+ cells in the sevoflurane anesthesia group were significantly lower than those in the propofol anesthesia group, indicating that propofol may be beneficial in reducing perioperative immunosuppression, thus mitigating adverse prognoses in patients with cervical cancer (Liu et al., 2016). In addition, a retrospective study comparing the effects of anesthesia on overall, cancer-specific, and recurrence-free survival in patients undergoing surgery for cervical cancer reported that propofol was associated with better long-term outcomes (Takeyama et al., 2021). These findings suggest that propofol has an antitumor effect on cervical cancer.
2.2 Ketamine
Ketamine acts rapidly and does not cause respiratory depression, which supports its safety and widespread use. However, studies have demonstrated that ketamine has immunosuppressive effects that may adversely affect tumor prognosis. Ketamine may promote tumor metastasis by inhibiting NK cell activity and increasing the number of regulatory T cells (Tregs) to suppress immunity (Melamed et al., 2003; Hou et al., 2016). However, recent clinical and in vitro studies have shown that ketamine has no effect on the cytotoxicity of NK cells in patients undergoing surgery for cancer (Kubota et al., 2022; Cho et al., 2021). Ketamine can even inhibit the proliferation and migration of cancer cells and induce ferroptosis (Li D. et al., 2021; Hu et al., 2020). Two clinical studies found that ketamine inhibited immune function in cervical cancer patients undergoing surgery (Wang et al., 2011; Jiang et al., 2022). Studies investigating the role of ketamine in cervical cancer cells are lacking and, thus, merit elaboration.
2.3 Etomidate
Etomidate is beneficial for improving hemodynamic stability during surgery. Many in vitro studies have shown that etomidate plays an antitumor role by suppressing cell proliferation and migration and promoting cell apoptosis (Chu et al., 2019; Li H. et al., 2021). In addition, a retrospective cohort study found that etomidate was more detrimental than propofol to the prognosis of patients who underwent radical gastrectomy (Lu et al., 2022). However, research investigating the relationship between etomidate and the progression of cervical cancer is lacking and, as such, merits further promotion.
2.4 Dexmedetomidine
Dexmedetomidine (DEX) is an α-2A adrenergic receptor (ADRA2A) agonist with unique sedative and analgesic effects and minimal respiratory depression, and is widely used in the clinic. A meta-analysis revealed that DEX can reduce perioperative stress and inflammation, protect immune function in patients undergoing surgery, reduce postoperative complications, and improve prognosis (Wang et al., 2019). Studies have found that DEX promotes the proliferation and metastasis of breast, lung and colon cancers (Lavon et al., 2018), but plays an antitumor role in ovarian and esophageal cancers (Shin et al., 2021; Hu et al., 2021). These conflicting results may be attributed to differences in the duration and concentration of DEX exposure and tumor models. DEX plays multiple roles in cancer cells, the effects on tumor progression are complex and non-uniform, and there is a lack of high-quality clinical evidence on the prognostic effects of DEX in cancer patients. In vitro studies have shown that DEX inhibits cervical cancer cell proliferation and migration through the JAK/STAT signaling pathway (Xu et al., 2021). Data regarding the effects of DEX on the progression of cervical cancer are insufficient and further research is needed.
In summary, propofol and DEX may exert antitumor effects on cervical cancer, whereas the effects of ketamine and etomidate remain uncertain. More studies are needed to explore the relationship between intravenous anesthetics and the progression of cervical cancer and the molecular mechanisms involved, especially in clinical trials, to verify the effect of intravenous anesthetics on the long-term prognosis of cervical cancer in humans.
3 Volatile anesthetic agents
3.1 Sevoflurane
The biological effect of sevoflurane on cancer cells remains unclear. Sevoflurane enhances the proliferation and migration of ovarian cancer cells. Sevoflurane enhances the proliferation and migration of ovarian cancer cells (Ishikawa et al., 2021; Hu et al., 2022), but inhibits proliferation and migration of colorectal cancer and gastric cancer cells (Fan et al., 2019; Yong et al., 2021). Studies have shown that sevoflurane mediates antiproliferation and antimigration of cervical cancer cells by targeting resistance to audiogenic seizures (Ras) and ras homolog family member A (RhoA) and up-regulating miR-203 (Ding et al., 2019; Zhang et al., 2020a). However, another study suggested that sevoflurane promotes the proliferation of cervical cancer cells but has no effect on cisplatin sensitivity (Xue et al., 2019). Exposure to clinically relevant concentrations of sevoflurane induces the upregulation of histone deacetylase 6 through the phosphatidyl inositol 3-kinase (PI3K)/protein kinase B (AKT-) and extracellular regulated protein kinases (ERK1/2-) signaling pathways, thereby promoting the proliferation and metastatic potential of cervical cancer cells (Zhang et al., 2020a). These conflicting results can be attributed to the duration and concentration of sevoflurane exposure (Figure 1).
A retrospective cohort study showed that the prognosis of patients undergoing surgery for cervical cancer and received sevoflurane anesthesia was worse than that of those who received propofol anesthesia (Takeyama et al., 2021). However, studies have shown that the anesthetic effect of sevoflurane combined with remifentanil in laparoscopic radical surgery for cervical cancer is better than that of propofol combined with remifentanil. Sevoflurane combined with remifentanil anesthesia can also improve perioperative cellular immune function and relieve physiological stress (Wang et al., 2022; Suo et al., 2022).
3.2 Isoflurane
Isoflurane promotes proliferation, migration, and invasion in most cancer cells (Zheng et al., 2022; Benzonana et al., 2013). Isoflurane upregulated the expression of histone deacetylase 6, which is associated with the mTOR-dependent pathway, thereby promoting the proliferation of squamous cervical cancer cells (Zhang et al., 2021). Isoflurane promoted cell proliferation and inhibited apoptosis in cervical cancer by downregulating miR-375 (Li et al., 2019). However, another study reported that isoflurane inhibited the proliferation of cancer cells and promoted apoptosis and autophagy through the AMPK/mTOR pathway (Wei et al., 2021). In addition, isoflurane has a potential inhibitory effect on cervical cancer in nude mice (Ma et al., 2020). To our knowledge, no laboratory studies have investigated the relationship between isoflurane and the progression of cervical cancer.
3.3 Desflurane
Conclusions regarding the effects of desflurane on cancer prognosis are mixed. Studies have suggested that total intravenous and desflurane anesthesia have comparable effects on cancer prognosis in patients undergoing surgery (Cho et al., 2021). Studies have also shown that the survival rate of patients with gastric and colon cancer undergoing surgery and received total intravenous anesthesia with propofol was higher than that of those who received desflurane anesthesia during the 3–5-year follow-up period after surgery (Wu et al., 2018; Huang et al., 2020b). There have been no laboratory or clinical studies investigating the relationship between desflurane and the progression of cervical cancer.
Volatile anesthetics can affect cancer recurrence and metastasis by altering innate immunity and decreasing NK cell cytotoxicity (Buckley et al., 2014). One study demonstrated that propofol was superior to sevoflurane in protecting circulating lymphocytes in patients undergoing laparoscopic radical hysterectomy for cervical cancer (Liu et al., 2016). Moreover, the effect of volatile anesthetics on the prognosis of patients with cervical cancer remains controversial; as such, further laboratory and clinical trials are required to obtain more conclusive results.
4 Sedative and analgesic agents
4.1 Opioids
Opioids are the most commonly used perioperative analgesics in patients with cancer who undergo surgery. Opioids may contribute to cancer progression by suppressing immunity and promoting cancer-related inflammation, tumor cell migration, and angiogenesis (Perry and Douglas, 2019; Amaram-Davila et al., 2020; Abdel Shaheed et al., 2022). However, studies have shown that opioids may play an antitumor role by activating tumor cell apoptosis and reducing invasion and metastasis (Connolly and Buggy, 2016; Lec et al., 2020; Ramirez et al., 2021). These conflicting results may be related to the type and dosage of opioids, and the type of cancer.
Morphine is an opioid-based analgesic. In vitro studies have shown that morphine can promote the proliferation of cervical cancer cells by activating the opioid receptor-dependent EGFR-mediated signaling pathway and stimulate migration by activating the opioid receptor-independent RhoA-mediated signaling pathway (Yu et al., 2022). In contrast, sufentanil induces apoptosis in cervical cancer cells through the PI3K/AKT/mTOR signaling pathway (Jin et al., 2021). Another study reported that morphine suppresses immunity through the JAK3/STAT5 pathway (Jiang et al., 2022). A clinical study reported that bupivacaine combined with morphine inhibited postoperative immune function in patients with cervical cancer, whereas ropivacaine combined with fentanyl had a lower immunosuppressive effect (Dianqing et al., 2007). In addition, compared with fentanyl, remifentanil had a minimal effect on T lymphocytes after surgery for cervical cancer (Lu et al., 2018). The effect of opioids on the long-term prognosis of patients undergoing surgery for cervical cancer has not been extensively studied, and further research, especially clinical studies, is required to confirm this finding.
4.2 Benzodiazepines
Benzodiazepines have both sedative and hypnotic effects. The results of two meta-analyses suggested that there may be no association between benzodiazepines and survival in patients with cancer (O'Donnell et al., 2019; O'Donnell et al., 2018). However, in vitro and animal studies have shown that diazepam and midazolam exhibit antitumor and anti-inflammatory effects (Oshima et al., 2022; Wang et al., 2018; Kim et al., 2008). Midazolam may exert its antitumor effect by inhibiting the local invasion of tumor-associated neutrophils and tumor-associated macrophages, or by inhibiting their proliferation and migration. However, no laboratory or clinical studies have investigated the relationship between benzodiazepines and the progression of cervical cancer; therefore, further research is needed.
4.3 NSAIDs
NSAIDs are commonly used as analgesics during surgery. It is well known that inflammatory responses to tumor tissues may promote the occurrence and development of tumors (Nasry et al., 2018; Balkwill and Mantovani, 2001). In contrast, NSAIDs play an anti-inflammatory role by inhibiting cyclooxygenase (COX) enzymes to inhibit the synthesis of prostaglandins, thus playing an antitumor role (Retsky et al., 2013; Brusselaers and Lagergren, 2018).
NSAIDs have been shown to exert antitumor effects in cervical cancer in vitro and in animal models by inhibiting growth and migration, and inducing apoptosis (Soriano-Hernandez et al., 2015; Marinov et al., 2021; Kim et al., 2003). Celecoxib radio-sensitizes HeLa cells by downregulation of COX-2 and vascular endothelial growth factor C (VEGF-C) (Wang et al., 2012). Moreover, in addition to COX-2, NSAIDs, such as celecoxib, inhibit HeLa growth through tNOX, a cancer-specific cell surface oxidase (ECTO-NOX), via protein disulfide-thiol interchange activity (Morre and Morre, 2006). In vitro studies have also shown that NF-kappaB, DNA damage inducible gene (GADD153) and survivin play important roles in celecoxib-induced apoptosis (Kim et al., 2004; Kim et al., 2007; Fukada et al., 2007). Moreover, celecoxib induces apoptosis and cell cycle arrest of cervical cancer cells by upregulation of p53 through various molecular mechanisms (Setiawati and Setiawati, 2016; Saha et al., 2012). However, a meta-analysis revealed that the long-term use of NSAIDs was not associated with the progression of cervical intraepithelial neoplasia (Grabosch et al., 2018; Grabosch et al., 2014). More clinical trials are needed to confirm the relationship between NSAIDs and the long-term prognosis of cervical cancer.
5 Local anesthetic agents
Local anesthetics are typically used as regional and neuraxial anesthetics during the perioperative period. Studies have shown that local anesthetics can enhance immune response and reduce inflammation, thus exhibiting antitumor effects (Pérez-González et al., 2017; Ramirez et al., 2015). In addition, many in vitro experiments have demonstrated that local anesthetics may inhibit the growth, migration, and invasive capacity of cancer cells, and induce apoptosis and autophagy through various mechanisms, thereby playing an inhibitory role in tumor progression (Chen et al., 2022; Li et al., 2018). However, a meta-analysis revealed that perioperative local anesthesia may improve the survival of patients with cancer after oncological surgery, although there is no evidence supporting a correlation with cancer recurrence after oncological surgery (Sun et al., 2015).
Studies have found that lidocaine, a commonly used local anesthetic, decreases Ki-67 expression in cervical cancer cells, thus inhibiting their growth (Haraguchi-Suzuki et al., 2022). LncRNA maternally expressed gene 3 (lncRNA-MEG3) is associated with the progression of cervical cancer (Wang et al., 2017). In an in vitro study, lidocaine inhibited the proliferation and induced apoptosis of cervical cancer cells by activating the lncRNA-MEG3/miR-421/BTG anti-proliferation factor 1 (BTG1) pathway (Zhu and Han, 2019). Ropivacaine has been shown to exert similar antitumor effects by reducing miR-96 expression and upregulating MEG2 expression, leading to STAT3 dephosphorylation (Chen et al., 2020). Clinical studies have demonstrated that intraoperative lidocaine has a protective effect on perioperative immune function in patients with cervical cancer undergoing radical hysterectomy, which may inhibit tumor metastasis (Wang et al., 2015; Hong and Lim, 2008). Clinical studies investigating the relationship between local anesthetics and the progression of cervical cancer are lacking. As such, further research is needed to determine the long-term effects of local anesthetics in patients undergoing surgery for cervical cancer.
6 Conclusion
Perioperative anesthetics affect cancer progression. Herein, we summarized the long-term postoperative effects of anesthetics on patients with cervical cancer. Current in vitro studies have demonstrated that propofol, DEX, remifentanil, celecoxib, and local anesthetics, such as lidocaine, may exert antitumor effects on cervical cancer. The effects of sevoflurane and isoflurane on cervical cancer, however, remain controversial (Figure 2). Studies have demonstrated that shorter periods of sevoflurane and isoflurane use may have tumor-promoting effects on cervical cancer, although further basic research is needed to confirm this.
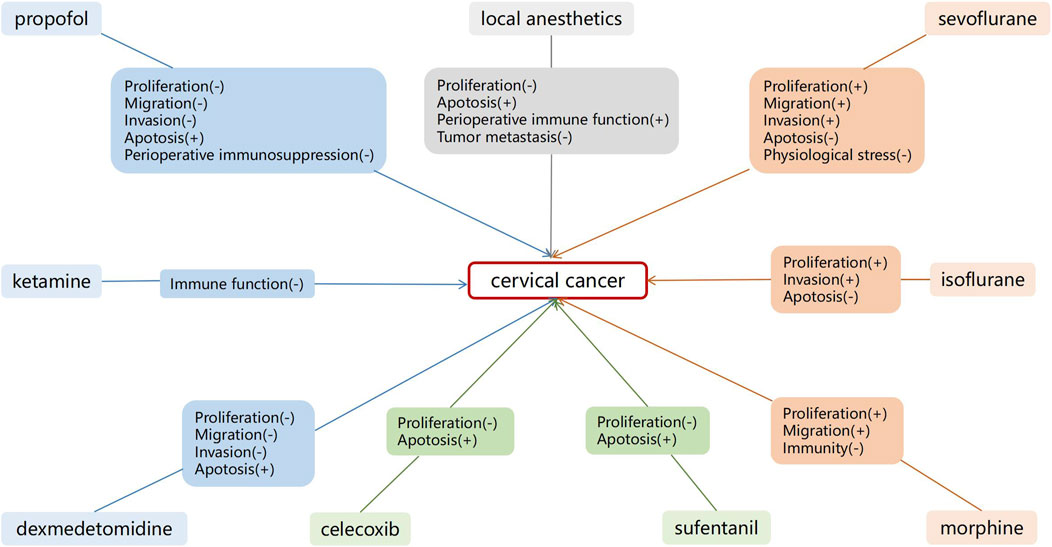
Figure 2. Effects of anesthetics on cervical cancer cells. Shorter duration of sevoflurane and isoflurane use may have a tumor promoting effect on cervical cancer.
Clinical trials aimed at confirming that the perioperative use of DEX, remifentanil, and celecoxib can reduce tumor recurrence and prolong the survival of patients undergoing surgery for cervical cancer are lacking. Furthermore, randomized controlled trials that evaluate the impact of perioperative lidocaine use on patients undergoing cervical cancer surgery have not included assessments of patient survival (Wang et al., 2015; Hong and Lim, 2008). The investigation into the effects of perioperative sevoflurane and propofol on the prognosis of patients with cervical cancer is limited to retrospective cohort studies (Takeyama et al., 2021), with a distinct lack of randomized controlled trials. Presently, there is an absence of robust clinical evidence, such as randomized controlled trials or high-quality meta-analyses, to substantiate the influence of various anesthetic agents on the long-term prognosis of patients following cervical cancer surgery. Therefore, more prospective studies and long-term follow-ups of cancer recurrence after surgery are needed to develop more sophisticated and effective anesthesia protocols for patients undergoing surgery for cervical cancer.
Author contributions
LD: Writing–original draft. TW: Writing–original draft. QZ: Writing–review and editing. SS: Writing–review and editing. XC: Writing–review and editing.
Funding
The author(s) declare that financial support was received for the research, authorship, and/or publication of this article. This study was funded by National Natural Science Foundation (grant 82071251 to XC); Hubei Province Key Research and Development Program (grant 2021BCA145 to XC).
Conflict of interest
The authors declare that the research was conducted in the absence of any commercial or financial relationships that could be construed as a potential conflict of interest.
Generative AI statement
The author(s) declare that no Generative AI was used in the creation of this manuscript.
Publisher’s note
All claims expressed in this article are solely those of the authors and do not necessarily represent those of their affiliated organizations, or those of the publisher, the editors and the reviewers. Any product that may be evaluated in this article, or claim that may be made by its manufacturer, is not guaranteed or endorsed by the publisher.
References
Abdel Shaheed, C., Beardsley, J., Day, R. O., and McLachlan, A. J. (2022). 'Immunomodulatory effects of pharmaceutical opioids and antipyretic analgesics: mechanisms and relevance to infection. Br. J. Clin. Pharmacol. 88, 3114–3131. doi:10.1111/bcp.15281
Ackerman, R. S., Luddy, K. A., Icard, B. E., Piñeiro Fernández, J., Gatenby, R. A., and Muncey, A. R. (2021). The effects of anesthetics and perioperative medications on immune function: a narrative review. Anesth. Analg. 133, 676–689. doi:10.1213/ANE.0000000000005607
Amaram-Davila, J., Davis, M., and Reddy, A. (2020). Opioids and cancer mortality. Curr. Treat. Options Oncol. 21, 22. doi:10.1007/s11864-020-0713-7
Baik, S., Mehta, F. F., Unsal, E., Park, Y., and Chung, S. H. (2022). Estrogen inhibits epithelial progesterone receptor-dependent progestin therapy efficacy in a mouse model of cervical cancer. Am. J. Pathol. 192, 353–360. doi:10.1016/j.ajpath.2021.10.008
Balkwill, F., and Mantovani, A. (2001). Inflammation and cancer: back to Virchow? Lancet 357, 539–545. doi:10.1016/S0140-6736(00)04046-0
Basaran, B., Gundogdu, H., Tutar, S., and Gulerman, C. (2019). Relationship between serum estrogen concentration and propofol consumption: a prospective observational study of patients undergoing oocyte retrieval. J. Obstet. Gynaecol. Res. 45, 148–155. doi:10.1111/jog.13793
Benzonana, L. L., Perry, N. J., Watts, H. R., Yang, B., Perry, I. A., Coombes, C., et al. (2013). 'Isoflurane, a commonly used volatile anesthetic, enhances renal cancer growth and malignant potential via the hypoxia-inducible factor cellular signaling pathway in vitro. Anesthesiology 119, 593–605. doi:10.1097/ALN.0b013e31829e47fd
Bray, F., Laversanne, M., Sung, H., Ferlay, J., Siegel, R. L., Soerjomataram, I., et al. (2024). 'Global cancer statistics 2022: GLOBOCAN estimates of incidence and mortality worldwide for 36 cancers in 185 countries. CA Cancer J. Clin. 74, 229–263. doi:10.3322/caac.21834
Brusselaers, N., and Lagergren, J. (2018). 'Maintenance use of non-steroidal anti-inflammatory drugs and risk of gastrointestinal cancer in a nationwide population-based cohort study in Sweden. BMJ Open 8, e021869. doi:10.1136/bmjopen-2018-021869
Buckley, A., McQuaid, S., Johnson, P., and Buggy, D. J. (2014). Effect of anaesthetic technique on the natural killer cell anti-tumour activity of serum from women undergoing breast cancer surgery: a pilot study. Br. J. Anaesth. 113 (Suppl. 1), i56–i62. doi:10.1093/bja/aeu200
Chen, J. L., Liu, S. T., Huang, S. M., and Wu, Z. F. (2022). 'Apoptosis, proliferation, and autophagy are involved in local anesthetic-induced cytotoxicity of human breast cancer cells. Int. J. Mol. Sci. 23, 15455. doi:10.3390/ijms232415455
Chen, Xi, Li, K., and Zhao, G. (2018). Propofol inhibits HeLa cells by impairing autophagic flux via AMP-activated protein kinase (AMPK) activation and endoplasmic reticulum stress regulated by calcium. Med. Sci. Monit. 24, 2339–2349. doi:10.12659/msm.909144
Chen, X., Liu, W., Guo, X., Huang, S., and Song, X. (2020). Ropivacaine inhibits cervical cancer cell growth via suppression of the miR-96/MEG2/pSTAT3 axis. Oncol. Rep. 43, 1659–1668. doi:10.3892/or.2020.7521
Cho, J. S., Kim, N. Y., Shim, J. K., Jun, J. H., Lee, S., and Kwak, Y. L. (2021). The immunomodulatory effect of ketamine in colorectal cancer surgery: a randomized-controlled trial. Can. J. Anaesth. 68, 683–692. doi:10.1007/s12630-021-01925-3
Chu, C. N., Wu, K. C., Chung, W. S., Zheng, L. C., Juan, T. K., Hsiao, Y. T., et al. (2019). 'Etomidate suppresses invasion and migration of human A549 lung adenocarcinoma cells. Anticancer Res. 39, 215–223. doi:10.21873/anticanres.13100
Connolly, C., and Buggy, D. J. (2016). Opioids and tumour metastasis: does the choice of the anesthetic-analgesic technique influence outcome after cancer surgery? Curr. Opin. Anaesthesiol. 29, 468–474. doi:10.1097/ACO.0000000000000360
Dianqing, C. A. O., Hanxin, W. E. N., Cuirong, G. U. O., and Xin, L. I. U. (2007). Influence of PCEA with compounds of Ropivacaine and Fentanyl on immunofunction in patients with cervical cancer. China J. Madern Med. 17, 195–197.
Ding, J., Zhang, L., Zeng, S., and Feng, T. (2019). Clinically relevant concentration of sevoflurane suppresses cervical cancer growth and migration through targeting multiple oncogenic pathways. Biochem. Biophys. Res. Commun. 514, 1179–1184. doi:10.1016/j.bbrc.2019.05.082
Dogru, S., Dogru, H. Y., Butun, I., Arici, A., Benli, I., Karaman, T., et al. (2017). Effects of sevoflurane on female reproductive functions in Wistar rats. J. Pak Med. Assoc. 67, 877–883.
Du, X.-T., Wang, X.-Y., Zheng, Y.-He, and Liu, D.-P. (2021a). 'Propofol suppresses the growth and invasion of cervical carcinoma cells by inhibiting MIR155HG. Aging-Us 13, 24464–24475. doi:10.18632/aging.203697
Du, Y., Zhang, X., Zhang, H., Chen, Y., Zhu, S., Shu, J., et al. (2021b). 'Propofol modulates the proliferation, invasion and migration of bladder cancer cells through the miR-145-5p/TOP2A axis. Mol. Med. Rep. 23, 439. doi:10.3892/mmr.2021.12078
Fan, L., Wu, Y., Wang, J., He, J., and Han, X. (2019). Sevoflurane inhibits the migration and invasion of colorectal cancer cells through regulating ERK/MMP-9 pathway by up-regulating miR-203. Eur. J. Pharmacol. 850, 43–52. doi:10.1016/j.ejphar.2019.01.025
Fukada, K., Takahashi-Yanaga, F., Sakoguchi-Okada, N., Shiraishi, F., Miwa, Y., Morimoto, S., et al. (2007). Celecoxib induces apoptosis by inhibiting the expression of survivin in HeLa cells. Biochem. Biophys. Res. Commun. 357, 1166–1171. doi:10.1016/j.bbrc.2007.04.077
Grabosch, S. M., Shariff, O. M., and William Helm, C. (2018). Non-steroidal anti-inflammatory agents to induce regression and prevent the progression of cervical intraepithelial neoplasia. Cochrane Database Syst. Rev. 2, CD004121. doi:10.1002/14651858.CD004121.pub4
Grabosch, S. M., Shariff, O. M., Wulff, J. L., and William Helm, C. (2014). Non-steroidal anti-inflammatory agents to induce regression and prevent the progression of cervical intraepithelial neoplasia. Cochrane Database Syst. Rev. 2014, CD004121. doi:10.1002/14651858.CD004121.pub3
Haraguchi-Suzuki, K., Kawabata-Iwakawa, R., Suzuki, T., Suto, T., Takazawa, T., and Saito, S. (2022). Local anesthetic lidocaine induces growth suppression of HeLa cells by decreasing and changing the cellular localization of the proliferation marker Ki-67. Genes cells. 27, 675–684. doi:10.1111/gtc.12983
Hong, J. Y., and Lim, K. T. (2008). Effect of preemptive epidural analgesia on cytokine response and postoperative pain in laparoscopic radical hysterectomy for cervical cancer. Reg. Anesth. Pain Med. 33, 44–51. doi:10.1016/j.rapm.2007.07.010
Hou, M., Zhou, N. B., Li, H., Wang, B. S., Wang, X. Q., Wang, X. W., et al. (2016). Morphine and ketamine inhibit immune function of gastric cancer patients by increasing percentage of CD4(+)CD25(+)Foxp3(+) regulatory T cells in vitro. J. Surg. Res. 203, 306–312. doi:10.1016/j.jss.2016.02.031
Hu, C., Wang, B., Liu, Z., Chen, Q., Ishikawa, M., Lin, H., et al. (2022). Sevoflurane but not propofol enhances ovarian cancer cell biology through regulating cellular metabolic and signaling mechanisms. Cell Biol. Toxicol. 39, 1395–1411. doi:10.1007/s10565-022-09766-6
Hu, J., Duan, W., and Liu, Y. (2020). Ketamine inhibits aerobic glycolysis in colorectal cancer cells by blocking the NMDA receptor-CaMK II-c-Myc pathway. Clin. Exp. Pharmacol. Physiol. 47, 848–856. doi:10.1111/1440-1681.13248
Hu, Y., Qiu, L. L., Zhao, Z. F., Long, Y. X., and Yang, T. (2021). Dexmedetomidine represses proliferation and promotes apoptosis of esophageal cancer cells by regulating C-Myc gene expression via the ERK signaling pathway. Eur. Rev. Med. Pharmacol. Sci. 25, 950–956. doi:10.26355/eurrev_202101_24664
Huang, J., Pan, M., Wang, L., and Tu, Y. (2020a). Regulation effects of propofol inhibition of wnt/beta-catenin pathway on growth and motor ability of cervical cancer HELA cells. Chin. J. Immunol. 36, 2861–2865.
Huang, N.-C., Lee, M.-S., Lai, H.-C., Lin, H.-T., Huang, Y.-H., Lu, C.-He, et al. (2020b). Propofol-based total intravenous anesthesia improves survival compared to desflurane anesthesia in gastric cancer surgery A retrospective analysis. Med. Baltim. 99, e20714. doi:10.1097/MD.0000000000020714
Ishikawa, M., Iwasaki, M., Zhao, H., Saito, J., Hu, C., Sun, Q., et al. (2021). Sevoflurane and desflurane exposure enhanced cell proliferation and migration in ovarian cancer cells via miR-210 and miR-138 downregulation. Int. J. Mol. Sci. 22, 1826. doi:10.3390/ijms22041826
Jiang, Y., Tong, Li, Qian, Yi, Zuo, X., and Liu, J. (2022). Morphine in combination with ketamine improves cervical cancer pain and suppresses immune function via the JAK3/STAT5 pathway. Pain Res. and Manag. 2022, 9364365. doi:10.1155/2022/9364365
Jin, Y., Sun, L., and Liu, K.-C. (2021). Effect of sufentanil on the viability and apoptosis of cervical cancer cells via the inactivation of PI3K/AKT/mTOR signaling pathway. Eur. J. Gynaecol. Oncol. 42, 325–332. doi:10.31083/j.ejgo.2021.02.2257
Kim, D. H., Lee, J. T., Lee, I. K., and Ha, J. H. (2008). Comparative anticancer effects of flavonoids and diazepam in cultured cancer cells. Biol. Pharm. Bull. 31, 255–259. doi:10.1248/bpb.31.255
Kim, K. Y., Seol, J. Y., Jeon, G. A., and Nam, M. J. (2003). The combined treatment of aspirin and radiation induces apoptosis by the regulation of bcl-2 and caspase-3 in human cervical cancer cell. Cancer Lett. 189, 157–166. doi:10.1016/s0304-3835(02)00519-0
Kim, R. (2018). Effects of surgery and anesthetic choice on immunosuppression and cancer recurrence. J. Transl. Med. 16, 8. doi:10.1186/s12967-018-1389-7
Kim, S. H., Hwang, C. I., Juhnn, Y. S., Lee, J. H., Park, W. Y., and Song, Y. S. (2007). GADD153 mediates celecoxib-induced apoptosis in cervical cancer cells. Carcinogenesis 28, 223–231. doi:10.1093/carcin/bgl227
Kim, S. H., Song, S. H., Kim, S. G., Chun, K. S., Lim, S. Y., Na, H. K., et al. (2004). Celecoxib induces apoptosis in cervical cancer cells independent of cyclooxygenase using NF-kappaB as a possible target. J. Cancer Res. Clin. Oncol. 130, 551–560. doi:10.1007/s00432-004-0567-6
Kubota, M., Niwa, H., Seya, K., Kawaguchi, J., Kushikata, T., and Hirota, K. (2022). Ketamine does not change natural killer cell cytotoxicity in patients undergoing cancer surgery: basic experiment and clinical trial. J. Oncol. 2022, 8946269. doi:10.1155/2022/8946269
Lavon, H., Matzner, P., Benbenishty, A., Sorski, L., Rossene, E., Haldar, R., et al. (2018). Dexmedetomidine promotes metastasis in rodent models of breast, lung, and colon cancers. Br. J. Anaesth. 120, 188–196. doi:10.1016/j.bja.2017.11.004
Lec, P. M., Lenis, A. T., Golla, V., Brisbane, W., Shuch, B., Garraway, I. P., et al. (2020). The role of opioids and their receptors in urological malignancy: a review. J. Urol. 204, 1150–1159. doi:10.1097/JU.0000000000001156
Li, D., Zhang, J., Yin, L., Jin, Z., Chen, X., and Meng, X. (2021a). Etomidate inhibits cell proliferation and induces apoptosis in A549 non-small cell lung cancer cells via downregulating WWP2. Exp. Ther. Med. 22, 1254. doi:10.3892/etm.2021.10689
Li, H., Liu, W., Zhang, X., Wu, F., Sun, D., and Wang, Z. (2021b). 'Ketamine suppresses proliferation and induces ferroptosis and apoptosis of breast cancer cells by targeting KAT5/GPX4 axis. Biochem. Biophys. Res. Commun. 585, 111–116. doi:10.1016/j.bbrc.2021.11.029
Li, H., Lu, Y., Pang, Y., Li, M., Cheng, Xi, and Chen, J. (2017). Propofol enhances the cisplatin-induced apoptosis on cervical cancer cells via EGFR/JAK2/STAT3 pathway. Biomed. and Pharmacother. 86, 324–333. doi:10.1016/j.biopha.2016.12.036
Li, J., Wang, Y., Yu, J., Dong, R., and Qiu, H. (2015). A high level of circulating HOTAIR is associated with progression and poor prognosis of cervical cancer. Tumour Biol. 36, 1661–1665. doi:10.1007/s13277-014-2765-4
Li, R., Xiao, C., Liu, H., Huang, Y., Dilger, J. P., and Lin, J. (2018). Effects of local anesthetics on breast cancer cell viability and migration. BMC Cancer 18, 666. doi:10.1186/s12885-018-4576-2
Li, Y., Liu, R., Li, J., Dong, X., Shen, J., and Wu, S. (2019). Isoflurane promotes proliferation and invasion of cervical carcinoma cells via downregulation of miR-375 expression. Eur. J. Gynaecol. Oncol. 40, 368–372. doi:10.12892/ejgo4406.2019
Liu, S., Gu, X., Zhu, L., Wu, G., Zhou, H., Song, Y., et al. (2016). Effects of propofol and sevoflurane on perioperative immune response in patients undergoing laparoscopic radical hysterectomy for cervical cancer. Med. Baltim. 95, e5479. doi:10.1097/MD.0000000000005479
Liu, Y. P., Qiu, Z. Z., Li, X. H., and Li, E. Y. (2021). 'Propofol induces ferroptosis and inhibits malignant phenotypes of gastric cancer cells by regulating miR-125b-5p/STAT3 axis. World J. Gastrointest. Oncol. 13, 2114–2128. doi:10.4251/wjgo.v13.i12.2114
Lo, H. C., Xu, Z., Kim, I. S., Pingel, B., Aguirre, S., Kodali, S., et al. (2020). Resistance to natural killer cell immunosurveillance confers a selective advantage to polyclonal metastasis. Nat. Cancer 1, 709–722. doi:10.1038/s43018-020-0068-9
López-Soto, A., Gonzalez, S., Smyth, M. J., and Galluzzi, L. (2017). 'Control of metastasis by NK cells. Cancer Cell 32, 135–154. doi:10.1016/j.ccell.2017.06.009
Lu, X., Yu, Y., Wang, Y., and Lyu, Y. (2022). Effect of propofol or etomidate as general anaesthesia induction on gastric cancer: a retrospective cohort study with 10 Years' follow-up. Cancer Manag. Res. 14, 2399–2407. doi:10.2147/CMAR.S361052
Lu, X. Y., Chen, M., Chen, D. H., Li, Y., Liu, P. T., and Liu, Y. (2018). Remifentanil on T lymphocytes, cognitive function and inflammatory cytokines of patients undergoing radical surgery for cervical cancer. Eur. Rev. Med. Pharmacol. Sci. 22, 2854–2859. doi:10.26355/eurrev_201805_14987
Ma, D., Li, Hu, and Liu, S. (2020). Effects of isoflurane on the proliferation and apoptosis of cervical cancer in nude mice and cervical cancer cells. Chin. J. Clin. Pharmacol. 36, 2273–2276.
Marinov, L., Georgieva, A., Voynikov, Y., Toshkova, R., Nikolova, I., and Malchev, M. (2021). Cytotoxic and antiproliferative effects of the nonsteroidal anti-inflammatory drug diclofenac in human tumour cell lines. Biotechnol. and Biotechnol. Equip. 35, 1118–1126. doi:10.1080/13102818.2021.1953401
Melamed, R., Bar-Yosef, S., Shakhar, G., Shakhar, K., and Ben-Eliyahu, S. (2003). Suppression of natural killer cell activity and promotion of tumor metastasis by ketamine, thiopental, and halothane, but not by propofol: mediating mechanisms and prophylactic measures. Anesth. Analg. 97, 1331–1339. doi:10.1213/01.ane.0000082995.44040.07
Morre, D. J., and Morre, D. M. (2006). tNOX, an alternative target to COX-2 to explain the anticancer activities of non-steroidal anti-inflammatory drugs (NSAIDS). Mol. Cell Biochem. 283, 159–167. doi:10.1007/s11010-006-2568-z
Nasry, W. H. S., Rodriguez-Lecompte, J. C., and Martin, C. K. (2018). 'Role of COX-2/PGE2 mediated inflammation in oral squamous cell carcinoma. Cancers (Basel) 10, 348. doi:10.3390/cancers10100348
O'Donnell, S. B., Nicholson, M. K., and Boland, J. W. (2018). The association of benzodiazepines and z-drugs with mortality in patients with cancer: a systematic review. BMJ Support Palliat. Care 8, A9.
O'Donnell, S. B., Nicholson, M. K., and Boland, J. W. (2019). The association between benzodiazepines and survival in patients with cancer: a systematic review. J. Pain Symptom Manage 57, 999–1008.e11. doi:10.1016/j.jpainsymman.2019.01.010
Oshima, Y., Sano, M., Kajiwara, I., Ichimaru, Y., Itaya, T., Kuramochi, T., et al. (2022). Midazolam exhibits antitumour and anti-inflammatory effects in a mouse model of pancreatic ductal adenocarcinoma. Br. J. Anaesth. 128, 679–690. doi:10.1016/j.bja.2021.12.042
Pérez-González, O., Cuéllar-Guzmán, L. F., Soliz, J., and Cata, J. P. (2017). Impact of regional anesthesia on recurrence, metastasis, and immune response in breast cancer surgery: a systematic review of the literature. Reg. Anesth. Pain Med. 42, 751–756. doi:10.1097/AAP.0000000000000662
Perry, J. A., and Douglas, H. (2019). Immunomodulatory effects of surgery, pain, and opioids in cancer patients. Vet. Clin. North Am. Small Anim. Pract. 49, 981–991. doi:10.1016/j.cvsm.2019.07.008
Ramirez, M. F., Gorur, A., and Cata, J. P. (2021). Opioids and cancer prognosis: a summary of the clinical evidence. Neurosci. Lett. 746, 135661. doi:10.1016/j.neulet.2021.135661
Ramirez, M. F., Tran, P., and Cata, J. P. (2015). The effect of clinically therapeutic plasma concentrations of lidocaine on natural killer cell cytotoxicity. Reg. Anesth. Pain Med. 40, 43–48. doi:10.1097/AAP.0000000000000191
Retsky, M., Demicheli, R., Hrushesky, W. J., Forget, P., De Kock, M., Gukas, I., et al. (2013). Reduction of breast cancer relapses with perioperative non-steroidal anti-inflammatory drugs: new findings and a review. Curr. Med. Chem. 20, 4163–4176. doi:10.2174/09298673113209990250
Saha, B., Adhikary, A., Ray, P., Saha, S., Chakraborty, S., Mohanty, S., et al. (2012). 'Restoration of tumor suppressor p53 by differentially regulating pro- and anti-p53 networks in HPV-18-infected cervical cancer cells. Oncogene 31, 173–186. doi:10.1038/onc.2011.234
Saka-Herrán, C., Jané-Salas, E., Estrugo-Devesa, A., and López-López, J. (2021). 'Head and neck cancer and non-steroidal anti-inflammatory drugs: systematic review and meta-analysis. Head. Neck 43, 1664–1682. doi:10.1002/hed.26663
Sessler, D. I., and Riedel, B. (2019). Anesthesia and cancer recurrence: context for divergent study outcomes. Anesthesiology 130, 3–5. doi:10.1097/ALN.0000000000002506
Setiawati, A., and Setiawati, A. (2016). Celecoxib, a COX-2 selective inhibitor, induces cell cycle arrest at the G2/M phase in HeLa cervical cancer cells. Asian Pac J. Cancer Prev. 17, 1655–1660. doi:10.7314/apjcp.2016.17.4.1655
Shimizu, T., Inomata, S., and Tanaka, M. (2010). 'Progesterone decreases sevoflurane requirement in male mice: a dose-response study. Br. J. Anaesth. 104, 603–605. doi:10.1093/bja/aeq068
Shin, S., Kim, K. J., Hwang, H. J., Noh, S., Oh, J. E., and Yoo, Y. C. (2021). Immunomodulatory effects of perioperative dexmedetomidine in ovarian cancer: an in vitro and xenograft mouse model study. Front. Oncol. 11, 722743. doi:10.3389/fonc.2021.722743
Soriano-Hernandez, A. D., Madrigal-Perez, D., Galvan-Salazar, H. R., Martinez-Fierro, M. L., Valdez-Velazquez, L. L., Espinoza-Gomez, F., et al. (2015). Anti-inflammatory drugs and uterine cervical cancer cells: antineoplastic effect of meclofenamic acid. Oncol. Lett. 10, 2574–2578. doi:10.3892/ol.2015.3580
Sun, N., Zhang, W., Liu, J., Yang, X., and Chu, Q. (2021). Propofol inhibits the progression of cervical cancer by regulating HOTAIR/miR-129-5p/RPL14 Axis. Onco Targets Ther. 14, 551–564. doi:10.2147/OTT.S279942
Sun, Y., Li, T., and Gan, T. J. (2015). The effects of perioperative regional anesthesia and analgesia on cancer recurrence and survival after oncology surgery: a systematic review and meta-analysis. Reg. Anesth. Pain Med. 40, 589–598. doi:10.1097/AAP.0000000000000273
Suo, X., Wang, Z., and Zhu, Y. (2022). Application effect of sevoflurane combined with remifentanil intravenous inhalation anesthesia in patients undergoing laparoscopic radical resection of cervical cancer. Am. J. Transl. Res. 14, 1034–1042.
Takeyama, E., Miyo, M., Matsumoto, H., Tatsumi, K., Amano, E., Hirao, M., et al. (2021). 'Long-term survival differences between sevoflurane and propofol use in general anesthesia for gynecologic cancer surgery. J. Anesth. 35, 495–504. doi:10.1007/s00540-021-02941-9
Wang, A. H., Tian, X. Y., Yu, J. J., Mi, J. Q., Liu, H., and Wang, R. F. (2012). Celecoxib radiosensitizes the human cervical cancer HeLa cell line via a mechanism dependent on reduced cyclo-oxygenase-2 and vascular endothelial growth factor C expression. J. Int. Med. Res. 40, 56–66. doi:10.1177/147323001204000106
Wang, C., Datoo, T., Zhao, H., Wu, L., Date, A., Jiang, C., et al. (2018). Midazolam and dexmedetomidine affect neuroglioma and lung carcinoma cell biology in vitro and in vivo. Anesthesiology 129, 1000–1014. doi:10.1097/ALN.0000000000002401
Wang, H. L., Yan, H. D., Liu, Y. Y., Sun, B. Z., Huang, R., Wang, X. S., et al. (2015). Intraoperative intravenous lidocaine exerts a protective effect on cell-mediated immunity in patients undergoing radical hysterectomy. Mol. Med. Rep. 12, 7039–7044. doi:10.3892/mmr.2015.4235
Wang, K., Rao, Y., and Dong, X. (2022). Application of sevoflurane combined with remifentanil anesthesia in laparoscopic radical hysterectomy for cervical cancer. Am. J. Transl. Res. 14, 8361–8370.
Wang, K., Wu, M., Xu, J., Wu, C., Zhang, B., Wang, G., et al. (2019). Effects of dexmedetomidine on perioperative stress, inflammation, and immune function: systematic review and meta-analysis. Br. J. Anaesth. 123, 777–794. doi:10.1016/j.bja.2019.07.027
Wang, W., Liu, Y., and Qi, E. (2011). The effect of lower dosage of ketamine on Th cell drift and post-operation awakening in cervical cancer patients. J. Clin. Anesth. 27, 231–233.
Wang, X., Wang, Z., Wang, J., Wang, Y., Liu, L., and Xu, X. (2017). LncRNA MEG3 has anti-activity effects of cervical cancer. Biomed. Pharmacother. 94, 636–643. doi:10.1016/j.biopha.2017.07.056
Wei, H., Sun, T., Liu, J., Wang, X., Zhao, G., Shi, J., et al. (2021). 'Isoflurane activates AMP-activated protein kinase to inhibit proliferation, and promote apoptosis and autophagy in cervical carcinoma both in vitro and in vivo. J. Recept Signal Transduct. Res. 41, 538–545. doi:10.1080/10799893.2020.1831535
Wu, Z. F., Lee, M. S., Wong, C. S., Lu, C. H., Huang, Y. S., Lin, K. T., et al. (2018). 'Propofol-based total intravenous anesthesia is associated with better survival than desflurane anesthesia in colon cancer surgery. Anesthesiology 129, 932–941. doi:10.1097/ALN.0000000000002357
Xu, F. L., Xu, L., Xu, X., Huang, Z., and Su, L. (2021). Dexmedetomidine inhibited proliferation and invasion of cervical cancer cells by inhibiting the janus tyrosine kinase/signal transducer and activator of transcription signaling pathway. J. Biomaterials Tissue Eng. 11, 1293–1304. doi:10.1166/jbt.2021.2702
Xue, F., Xu, Y., Song, Y., Zhang, W., Li, R., and Zhu, X. (2019). The effects of sevoflurane on the progression and cisplatinum sensitivity of cervical cancer cells. Drug Des. Devel Ther. 13, 3919–3928. doi:10.2147/DDDT.S219788
Yap, A., Lopez-Olivo, M. A., Dubowitz, J., Hiller, J., and Riedel, B.Global Onco-Anesthesia Research Collaboration Group (2019). Anesthetic technique and cancer outcomes: a meta-analysis of total intravenous versus volatile anesthesia. Can. J. Anaesth. 66, 546–561. doi:10.1007/s12630-019-01330-x
Yong, F., Wang, H., Li, C., and Jia, H. (2021). 'Sevoflurane represses the migration and invasion of gastric cancer cells by regulating forkhead box protein 3. J. Int. Med. Res. 49, 3000605211005936. doi:10.1177/03000605211005936
Yoo, Y. A., Son, J., Mehta, F. F., DeMayo, F. J., Lydon, J. P., and Chung, S. H. (2013). Progesterone signaling inhibits cervical carcinogenesis in mice. Am. J. Pathol. 183, 1679–1687. doi:10.1016/j.ajpath.2013.07.026
Yu, X., Shi, J., Wang, X., and Zhang, F. (2020). Propofol affects the growth and metastasis of pancreatic cancer via ADAM8. Pharmacol. Rep. 72, 418–426. doi:10.1007/s43440-019-00015-y
Yu, Z., Jin, S., Tian, S., and Wang, Z. (2022). Morphine stimulates cervical cancer cells and alleviates cytotoxicity of chemotherapeutic drugs via opioid receptor-dependent and -independent mechanisms. Pharmacol. Res. and Perspect. 10, e01016. doi:10.1002/prp2.1016
Zhang, D., Zhou, X.-hui, Zhang, J., Zhou, Y.-xiao, Ying, J., Wu, G.-qing, et al. (2015). Propofol promotes cell apoptosis via inhibiting HOTAIR mediated mTOR pathway in cervical cancer. Biochem. Biophys. Res. Commun. 468, 561–567. doi:10.1016/j.bbrc.2015.10.129
Zhang, F., Wang, C., Cui, Y., Li, S., Yao, Y., Ci, Y., et al. (2016). Effects of propofol on several membrane characteristics of cervical cancer cell lines. Cell. Physiology Biochem. 40, 172–182. doi:10.1159/000452535
Zhang, Li, Shiyou, W., Xu, Z., Wen, S., and Lihua, H. (2020a). Sevoflurane inhibits growth and metastasis of cervical cancer through up-regulating miR-203 by targeting tumor protein translationally controlled 1. J. Biomaterials Tissue Eng. 10, 874–883. doi:10.1166/jbt.2020.2332
Zhang, W., Sheng, B., Chen, S., Zhao, H., Wu, L., Sun, Y., et al. (2020b). 'Sevoflurane enhances proliferation, metastatic potential of cervical cancer cells via the histone deacetylase 6 modulation in vitro. Anesthesiology 132, 1469–1481. doi:10.1097/ALN.0000000000003129
Zhang, W., Xue, F., Xie, S., Chen, C., Li, J., and Zhu, X. (2021). Isoflurane promotes proliferation of squamous cervical cancer cells through mTOR-histone deacetylase 6 pathway. Mol. Cell Biochem. 476, 45–55. doi:10.1007/s11010-020-03884-7
Zhao, M.-Y., Liu, P., Sun, C., Pei, L.-J., and Huang, Y.-G. (2022). Propofol augments paclitaxel-induced cervical cancer cell ferroptosis in vitro. Front. Pharmacol. 13, 816432. doi:10.3389/fphar.2022.816432
Zheng, J., Chen, G., Li, T., He, X., Luo, Y., and Yang, K. (2022). Isoflurane promotes cell proliferation, invasion, and migration by regulating BACH1 and miR-375 in prostate cancer cells in vitro. Int. J. Toxicol. 41, 212–224. doi:10.1177/10915818221084906
Zhou, Y.-H., Cui, Y.-H., Wang, T., and Luo, Y. (2020). Long non-coding RNA HOTAIR in cervical cancer: molecular marker, mechanistic insight, and therapeutic target. Adv. Clin. Chem. 97, 117–140. doi:10.1016/bs.acc.2019.12.004
Keywords: cervical cancer, anesthetics, perioperative, metastasis, recurrence
Citation: Deng L, Wang T, Zhang Q, Shu S and Chen X (2025) Effects of perioperative anesthetics on the postoperative prognosis of patients undergoing surgery for cervical cancer. Front. Pharmacol. 16:1536663. doi: 10.3389/fphar.2025.1536663
Received: 29 November 2024; Accepted: 14 February 2025;
Published: 10 March 2025.
Edited by:
Cheng Zhou, West China Hospital of Si Chuan University, ChinaCopyright © 2025 Deng, Wang, Zhang, Shu and Chen. This is an open-access article distributed under the terms of the Creative Commons Attribution License (CC BY). The use, distribution or reproduction in other forums is permitted, provided the original author(s) and the copyright owner(s) are credited and that the original publication in this journal is cited, in accordance with accepted academic practice. No use, distribution or reproduction is permitted which does not comply with these terms.
*Correspondence: Xiangdong Chen, eGRjaGVuQGh1c3QuZWR1LmNu
†These authors have contributed equally to this work and share first authorship