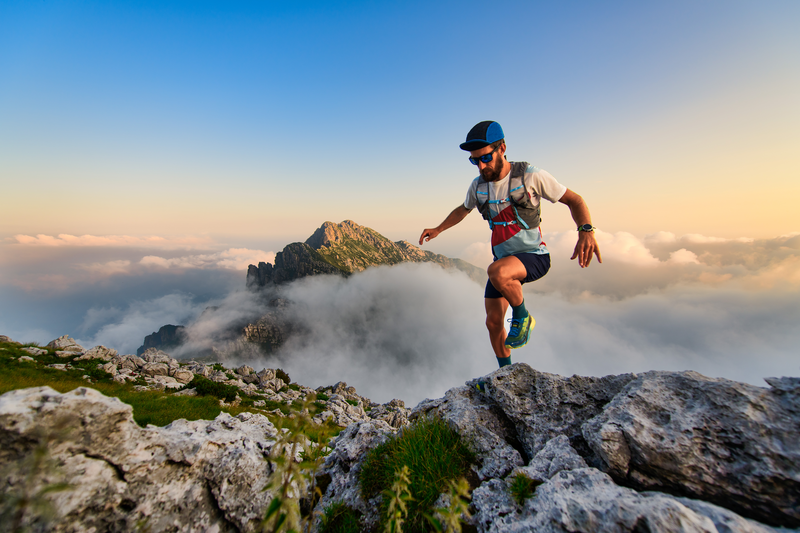
94% of researchers rate our articles as excellent or good
Learn more about the work of our research integrity team to safeguard the quality of each article we publish.
Find out more
ORIGINAL RESEARCH article
Front. Pharmacol.
Sec. Ethnopharmacology
Volume 16 - 2025 | doi: 10.3389/fphar.2025.1534748
The final, formatted version of the article will be published soon.
You have multiple emails registered with Frontiers:
Please enter your email address:
If you already have an account, please login
You don't have a Frontiers account ? You can register here
DDAs diester-diterpenoid alkaloids DESI-MSI desorption electrospray ionization mass spectrometry imaging Hezi Terminalia chebula Retz. HDI high-definition imaging HPLC high-performance liquid chromatography MDAs monoester-diterpenoid alkaloids NDAs non-esterified diterpene alkaloids OPLS-DA orthogonal partial least-square discriminant analysis PCA principal component analysis PLS-DA partial least-square discriminant analysis RSD relative standard deviation TBC Aconitum pendulum Busch UPLC ultra-high-performance liquid chromatography UPLC-Q-TOF-MS ultra-high-performance liquid chromatography-quadrupole time-of-flight mass spectrometry VIP variable importance for projection
Keywords: Tiebangchui, UPLC-Q-ToF-MS, Hezi-decoction-processed, Toxicity attenuation, DESI-MSI
Received: 26 Nov 2024; Accepted: 27 Feb 2025.
Copyright: © 2025 Zhou, Huimin, Pei, Sha, Li, Zhang, Su, Liu and Meng. This is an open-access article distributed under the terms of the Creative Commons Attribution License (CC BY). The use, distribution or reproduction in other forums is permitted, provided the original author(s) or licensor are credited and that the original publication in this journal is cited, in accordance with accepted academic practice. No use, distribution or reproduction is permitted which does not comply with these terms.
* Correspondence:
Zhen Zhou, Chengdu University of Traditional Chinese Medicine, Chengdu, China
Disclaimer: All claims expressed in this article are solely those of the authors and do not necessarily represent those of their affiliated organizations, or those of the publisher, the editors and the reviewers. Any product that may be evaluated in this article or claim that may be made by its manufacturer is not guaranteed or endorsed by the publisher.
Research integrity at Frontiers
Learn more about the work of our research integrity team to safeguard the quality of each article we publish.