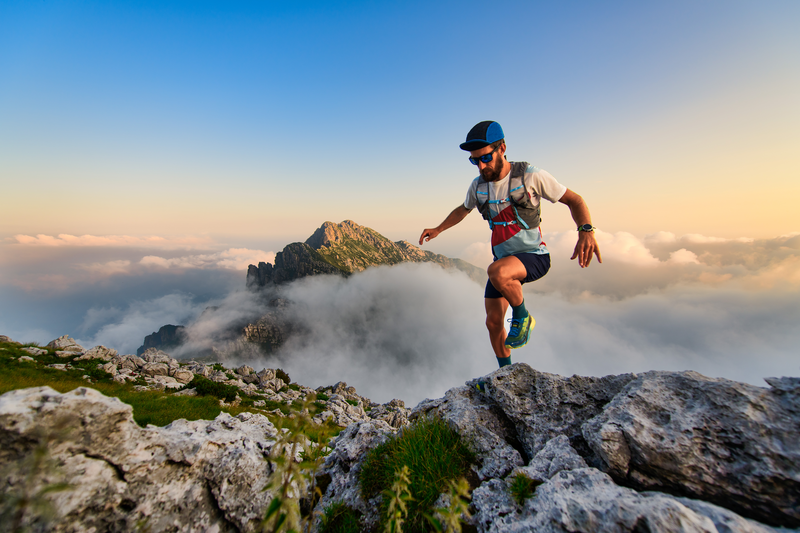
95% of researchers rate our articles as excellent or good
Learn more about the work of our research integrity team to safeguard the quality of each article we publish.
Find out more
REVIEW article
Front. Pharmacol. , 19 March 2025
Sec. Ethnopharmacology
Volume 16 - 2025 | https://doi.org/10.3389/fphar.2025.1527210
This article is part of the Research Topic Reviews in Ethnopharmacology: 2025 View all 10 articles
Boesenbergia rotunda: (L.) Mansf. (family Zingiberaceae), also known as fingerroot, is a medicinal and food plant that is widely distributed in southern China, Southeast Asia, and South Asia. It is a traditional herb and spice that is also known for its beneficial effects on Qi, appetite, stagnation and pain relief. The objective of this study is to conduct a comprehensive and systematic review of the botanical characteristics, traditional applications, phytochemical metabolites, pharmacological properties, toxicology, quality control measures, pharmacokinetics, and clinical applications of B. rotunda. A bibliometric analysis of current studies on B. rotunda was also conducted to facilitate further exploration and utilization of B. rotunda in the functional food and pharmaceutical industries. These data were collected from PubMed, Web of Science, Google Scholar, China National Knowledge Infrastructure doctoral and master’s theses and other books and scientific databases by searching the keywords Boesenbergia rotunda. Phytochemical analysis has revealed the presence of flavonoids, monoterpenes, alkaloids, aromatic metabolites, phenols, and other metabolites in B. rotunda, exhibiting a wide range of biological activities such as anti-cancer, nephroprotective, anti-inflammatory, anti-bacterial, hepatoprotective, anti-obesity, and anti-oxidant effects, both in vivo and in vitro. In this paper, the research of B. rotunda is discussed in depth by combining traditional application and modern pharmacological research, aiming to provide valuable reference for the future research and practical application of B. rotunda.
Boesenbergia rotunda (L.) Mansf., belonging to the Zingiberaceae family and colloquially referred to as fingerroot, is a versatile plant with both medicinal and culinary applications. It is extensively integrated into regional gastronomy and traditional medicinal practices. This plant is renowned for its diverse health benefits and is indigenous to Southern China, Southeast Asia, and South Asia (Vergoten and Bailly, 2022; Kaewjai et al., 2023). It has high ornamental value, medicinal value and edible value (Figures 1A–C) (Apiratikul et al., 2023; Zhang et al., 2023). B. rotunda is predominantly distributed across India, Myanmar, Thailand, China, Malaysia, and so on. In the pharmacopoeias of Vietnam, Cambodia, Laos and China, the rhizomes of this species have traditionally been recognized for their therapeutic effects, including reducing flatulence, relieving fatigue, improving symptoms of menstrual cramps, and boosting bile production (Figure 1D) (Kongratanapasert et al., 2023). The leaves of B. rotunda are notably broad and bear a resemblance to those of the banana plant, complemented by the plant’s aesthetically pleasing flowers. These characteristics give it some ornamental value (Jamornwan et al., 2022). In the field of medicine, the rhizome of B. rotunda is recognized as a medicinal botanical with distinctive pungent characteristics and warm thermogenic properties in traditional Chinese medicine. It is particularly esteemed for its multifaceted therapeutic potential, which includes the facilitation of Qi circulation, enhancement of appetite, mitigation of stagnation, and alleviation of pain. These pharmacological actions render B. rotunda an effective agent in the management of gastrointestinal distension, diarrhea, and an array of associated gastrointestinal disorders (Nguyen et al., 2021). At present, because of its rich medicinal value, it has been widely used in clinical treatment (Kongwattanakul et al., 2022). In Southeast Asia and other places, B. rotunda is still a commonly used seasoning for fish, vegetables and curry seasoning, which can remove the smell of food, and has a variety of effects such as nourishing (Rungsa et al., 2023).
Figure 1. The ornamental value of B. rotunda (A), products (B, C), along with a global distribution map of B. rotunda (D), can be accessed at the global biodiversity Information Facility (GBIF) website (https://www.gbif.org/species/2758480).
In recent years, B. rotunda has received increasing attention for its profound medicinal and nutritional value. Many scholars have conducted extensive and far-reaching research on B. rotunda (Techapichetvanich et al., 2024). Currently, over 200 distinctive metabolites have been isolated and identified through phytochemical investigations of various parts of B. rotunda. These include flavonoids, monoterpenes, alkaloids, aromatics, phenols, amides, esters, organic acids, diterpenes, and other metabolites. Notably, the abundant flavonoids and monoterpenes impart a unique fragrance to B. rotunda, enhancing its clinical application potential. Contemporary pharmacological studies reveal that the extracts and isolated metabolites exhibit a diverse range of biological activities, including significant anti-cancer activity (Widyananda et al., 2022), nephroprotective activity (Sujana et al., 2024), anti-inflammatory activity (Liana et al., 2024), anti-bacterial activity (Thadtapong et al., 2024), anti-obesity activity (Rungsa et al., 2023), hepatoprotective activity (Salama et al., 2018), anti-oxidant activity (Saah et al., 2024), and other bioactivities (Kaewjai et al., 2023). Furthermore, the rich nutritional profile and salutary metabolites present in B. rotunda offer considerable promise for application within the sphere of natural animal nutrition supplements and feed additives (Yushinta et al., 2023).
Despite the large number of research results on B. rotunda, the existing literature still lacks a holistic and systematic overview of B. rotunda, and the understanding of B. rotunda lacks a certain degree of comprehensiveness. The aim of this review is to improve the existing research on B. rotunda and critically summarize the latest progress, including geographical distribution, botanical characteristics, ethnomedical applications, phytochemical metabolites, pharmacological activities, quality control, toxicity assessment, and clinical settings. The ultimate goal of this review is to establish a solid scientific foundation for continued research, development, and application of B. rotunda, as well as to indicate potential avenues for future exploration and innovation.
A systematic literature search was performed utilizing online databases including PubMed, Elsevier, Web of Science, and China National Knowledge Infrastructure (CNKI), as well as repositories of doctoral and master’s theses. The search employed a variety of keywords, such as “B. rotunda”, “fingerroot”, and “B. rotunda (L.) Mansf”, to compile an extensive corpus of relevant literature. The data, encompassing aspects of botany, ethnopharmacology, phytochemistry, pharmacology, and toxicology pertaining to B. rotunda, were meticulously synthesized. Chemical metabolites identified were delineated using ChemDraw software. And bibliometric analysis of B. rotunda using VOSviewer, Web of Science, etc. The literature review spanned an unrestricted time frame, with eligible publications predominantly dating from 2002 to 2024.
B. rotunda is a perennial herbaceous species, attaining a stature of approximately 50 cm, and is notable for the absence of a conspicuous aerial stem (Figure 2A). The subterranean rhizome of this plant is ovate, yellowish in color, possesses a distinctive aroma, and exhibits a substantial thickness (Figure 2B) (Taheri et al., 2022). The foliar blades of B. rotunda, situated at the plant’s base, display an ovate-elongated to elliptic-lanceolate morphology, with lengths spanning from 25 to 50 cm and widths ranging from 7 to 12 cm. These leaves are distinguished by an acutely pointed apex and a base that tapers to a broadly rounded form (Figure 2C) (Bahadur Gurung et al., 2022). The inflorescence of B. rotunda, which resembles a spike, emerges exclusively from the rhizome and comprises three to six flowers (Figure 2D). The bracts are ovate, measuring 2–4 cm in length, with an acuminate apex and exhibit a pubescent texture. The flowers exhibit an enchanting blue-purple coloration. The calyx, 1–2 cm in length, features a cleft on one of its sides. The corolla tube can extend up to a maximum length of 4 cm, adorned with lanceolate lobes that measure 2.2–2.5 cm in length. The lateral staminodes are congruent in length to the corolla lobes and are oval in shape. The lip flap, which is obovate and cuneate, measures approximately 3 cm in length. The filaments are notably short, while the anthers are broad, characterized by linear appendages and linear anther locules, which are approximately 1 cm in length and bear a thickened stigma (https://www.worldfloraonline.org/taxon/wfo-0000341663). B. rotunda exhibits a distinctive growth pattern, flourishing in warm, humid, and semi-shaded environments. It is optimally suited for cultivation within tropical monsoon forests at elevations ranging from 700 to 1,900 m. The distribution of B. rotunda spans across the tropical monsoon forests of Southeast Asia, including regions in Malaysia, Singapore, Thailand, and the Philippines, as well as extending to the South Asian subcontinent, notably India and Sri Lanka, with sporadic occurrences in other locales (Liew et al., 2022). Additionally, B. rotunda is also found in Yunnan and other regions within China, where it has become a traditional Chinese medicine (Zhang et al., 2023).
Figure 2. The whole plant (A), roots (B), stems and leaves (C), and flowers (D) of B. rotunda (https://www.gbif.org/species/2758480).
B. rotunda is native to the Indonesian archipelago, specifically the islands of Java or Sumatra (Vergoten and Bailly, 2022). The plant is known in Southeast Asia for its healing properties. It is employed in the treatment of a spectrum of conditions, encompassing fever, rheumatism, muscular discomfort, peptic ulcers, gastrointestinal disturbances, bacterial infections, and a variety of other health complaints (Yuliana et al., 2013; Wang et al., 2022; Taechowisa et al., 2024). The remarkable therapeutic efficacy of B. rotunda has catalyzed its extensive utilization throughout the Southeast Asian region. This is further substantiated by its recognition and inclusion in the monograph on Association of Southeast Asian Nations herbal standards, which serves as a testament to its established medicinal value in the region (Kanchanapiboon et al., 2020). In Indonesia, B. rotunda is highly regarded as a health-promoting medicinal resource, known for addressing male sexual dysfunction and female infertility (Potipiranun et al., 2018). Within the traditional Indonesian healthcare framework, B. rotunda is considered invaluable for postpartum recovery, earning it the colloquial title of “ginseng in ginger” (Md-Mustafa et al., 2014). In Thailand, the plant is known locally as Thai Ginseng, and its rhizome extract is used to enhance wound repair, create anti-aging skincare formulations, and also to relieve stomach discomfort, manage leukoplakia, treat abscesses, and address leucorrhea (Kanchanapiboon et al., 2020). Meanwhile, in the context of traditional Thai medicine, the rhizome of Zingiber officinale is frequently prescribed for gastrointestinal complaints, including bloating, dyspepsia, and peptic ulcers (Mohan et al., 2020). Furthermore, in South Korea, a daily intake of up to 700 mg of a standardized finger root extract has been deemed both safe and efficacious (Vergoten and Bailly, 2022). It is also significant to note that both the extract derived from B. rotunda and its principal active metabolite, panduratin A, have demonstrated anti-viral activities against SARS-CoV-2 (Kaewjai et al., 2023).
Furthermore, B. rotunda is a staple in Southeast Asian culinary practices, prized for its nuanced flavor profile and invigorating aroma (Kabir et al., 2020). In Cambodia, B. rotunda is traditionally thinly sliced and pickled with fish broth to prepare the iconic Khmer dish, ‘Khmer Rice Noodle Soup’ Vietnamese cuisine employs B. rotunda as both a metabolic enhancer and an aromatic agent in the renowned ‘Lemon Leaf Vegetable Stew’ Indonesian gastronomy incorporates the rhizomes to lend a unique flavor to various porridges (San et al., 2022). In Thailand, B. rotunda is a pivotal condiment in the cherished shrimp soup, a dish particularly favored by nursing mothers due to its reputed ability to significantly enhance lactation (Rungsa et al., 2023). Collectively, B. rotunda functions not only as a savory seasoning but also as a nutraceutical food, enriching culinary creations with its distinctive essence while concurrently providing potential health benefits through dietary intervention.
Future research should further explore the traditional applications of B. rotunda, explore the mechanism of action of B. rotunda, search for the best therapeutic program, establish comprehensive safety information, and comprehensively safeguard the traditional applications of B. rotunda. In addition, comprehensive research on its dual role of medicinal and edible uses will further explore its application in medicine, thus expanding its role in modern medicine. Explore its potential in the field of functional foods and develop effective and reliable health foods.
To date, a total of approximately 205 chemical metabolites have been effectively extracted and characterized from diverse anatomical segments of B. rotunda. These metabolites mainly included flavonoids (1–83), monoterpenes (84–126), alkaloids (127–156), other aromatic metabolites (157–169), phenols (170–177), amides (178–183), lipids (184–188), organic acids (189–191), diterpenes (192–194), and other metabolites (195–205). Here, the present paper comprehensively compiled the chemical metabolites of bioactive metabolites and their related literature and chemical structures, as shown in Table 1 and Figure 3. This also encompasses the identification of new metabolites in recent years, which provides a foundation for the development of clinical drugs.
Table 1. Chemical metabolites isolated and identified from the different parts of Boesenbergia rotunda (L.) Mansf.
Figure 3. The structural compositions of the metabolites derived from B. rotunda have been elucidated.
Flavonoid metabolites are widely present in plants, and their special structures confer significant activity (Dhiman et al., 2016; Murti et al., 2021). Within the context of B. rotunda, flavonoids and their derivatives are recognized as the predominant pharmacologically active metabolites, exhibiting a prolific presence. To date, a cumulative total of 83 flavonoids and their derivatives (1–83) have been successfully isolated from B. rotunda. In recent years, these flavonoids have attracted considerable research interest, with studies revealing an impressive spectrum of biological activities. In a recent study, Thadtapong et al. (2024) identified that metabolite 20 potently inhibits multidrug-resistant Acinetobacter baumannii, showcasing remarkable anti-bacterial properties. Furthermore, Liana et al. (2024) reported that the flavonoid metabolite Pinostrobin (1), isolated from B. rotunda, exerts a significant inhibitory effect on atopic dermatitis. This finding implies the potential of Pinostrobin (1) as a topical skincare product or therapeutic agent for the management of this skin condition. These findings underscore the therapeutic potential of B. rotunda’s flavonoids and highlight the importance of continued research into their medicinal applications.
Monoterpene metabolites are ubiquitous in the plant kingdom and are important metabolites of plant essential oils. Although the structure is simple, it has significant biological activity (Liu et al., 2021). To date, approximately 43 distinct monoterpenes (84–126), have been identified in B. rotunda, serving as the principal determinants of its characteristic fragrance. Presently, research on monoterpene metabolites is predominantly concentrated on essential oil mixtures, which has resulted in a significant knowledge gap regarding the study of individual monoterpene metabolites.
In recent years, it has been found that alkaloid metabolites have good biological activity and can effectively treat various diseases (Kittakoop et al., 2022). To date, approximately 30 alkaloids (127–156), have been isolated and purified from B. rotunda, predominantly from its rhizomes. Nonetheless, the investigation of alkaloids in B. rotunda is still in its infancy, with a notable dearth of comprehensive studies exploring their pharmacological potential.
Additionally, B. rotunda is noted for its aromatic metabolites that incorporate a benzene ring structure. Thus far, approximately 13 distinct aromatic metabolites (157–169), have been identified within the rhizomes of B. rotunda. However, research pertaining to these specific metabolites is sparse, indicating a need for further investigation. Broadening the research horizon of B. rotunda necessitates an intensified investigation into the active aromatic metabolites present within this species.
Phenolic metabolites are widely used in the field of pharmaceutical cosmetics because of their wide range of pharmacological activities (Alu’datt et al., 2016). To date, 8 phenolic metabolites (170–177), have been successfully isolated from B. rotunda. However, the biological effects of these phenolic metabolites remain inadequately explored, and the specific biological activities of these metabolites are yet to be fully elucidated. This underscores an urgent requirement for additional research endeavors to uncover their potential therapeutic applications.
In recent years, amide metabolites have become an indispensable part of pharmacology due to their diverse biological activities (Narendar Reddy et al., 2019). In the context of B. rotunda, approximately six fatty alcohol metabolites (178–183), have been identified across various parts of the plant. Despite this, the current body of research regarding the biological activities of these specific metabolites is sparse, indicating a significant gap that warrants further exploration and investigation. This underscores the need for a more in-depth analysis of these metabolites to elucidate their potential roles and applications in pharmacology and medicine.
Naturally occurring lipid metabolites, which incorporate ester functionalities into their molecular structures, play a pivotal role in a multitude of biological processes. These metabolites are characterized by their unique physical and chemical properties, which are essential for various life functions. Within B. rotunda, five ester metabolites (184–188) have been identified, contributing to the plant’s metabolic profile. Despite their identification, the biological activities of these ester metabolites remain largely unexplored, indicating a significant gap in our understanding of their potential roles and applications.
Organic acid metabolites are widely present in plants. They play an important role in various industrial and biological applications (Yan et al., 2024). At present, three types of organic acid metabolites (189–191) have been found from B. rotunda. Despite their identification, there is a dearth of extensive research on the biological activities of these organic acid metabolites, suggesting a significant area for future investigative efforts.
Currently, B. rotunda has been identified to contain three diterpenoids, namely, metabolites 192–194. Notably, metabolites 193 and 194 have demonstrated significant anti-proliferative effects on human cancer cell lines, as reported by Win et al. (2019). The identification of these bioactive diterpenoids in B. rotunda suggests that they may play a crucial role in the plant’s chemotherapeutic applications, warranting in-depth investigation into their potential as novel cancer therapeutics.
In addition to the well-documented diterpenoids and other metabolites, B. rotunda has yielded a diverse array of organic metabolites, including those designated as 195–205, which have been identified across various parts of the plant. Despite the potential health-promoting effects suggested by their presence, the scientific literature on the specific health benefits of these metabolites is currently limited. This underscores an urgent research imperative to explore the bioactivity and potential applications of these organic metabolites within the context of B. rotunda.
At present, it has been found to have significant biological activity from the extracts and metabolites of B. rotunda. This suggests that B. rotunda can be widely used to prevent and improve a range of diseases. It has been found that B. rotunda has many other potential therapeutic effects such as anti-tumor, anti-cancer, anti-inflammatory, anti-bacterial, anti-oxidation and anti-obesity. The manifold biological activities of B. rotunda will be vividly delineated through a Sankey diagram, providing a visual synthesis of its multifaceted therapeutic potential (Figure 4) (Feng et al., 2024). Furthermore, Figures 5–7 elucidate the potential mechanisms that underpin the partial biological activities of B. rotunda extracts and their bioactive metabolites. A comprehensive overview of these metabolites and their associated bioactivities is presented in Table 2, with numerical references aligning with those in Table 1, which enumerates the names of each metabolite. These pharmacological findings of B. rotunda were further followed up with an exhaustive review and in-depth discussion to delve into its multiple biological activities.
Figure 5. A conceptual diagram illustrating the potential mechanism underlying the anti-cancer activity of B. rotunda is presented.
Figure 6. A diagrammatic depiction of the plausible mechanism underlying the nephroprotective activity of B. rotunda is provided.
Figure 7. A graphical illustration depicting the potential mechanism underlying the anti-inflammatory effects of B. rotunda is presented.
Table 2. Summary of biological activities of bioactive metabolites and extracts of Boesenbergia rotunda (L.). (Increase, ↑; Decrease, ↓).
In their comprehensive analysis of B. rotunda utilizing the KNApSAcK database, Widyananda et al. (2022) identified the presence of 20 metabolites, with chalcones and flavonoids being the most prevalent. Among these identified metabolites, seven metabolites have exhibited anti-cancer properties. These include Sakuranetin (19), Cardamonin (27), Alpinetin (3), Pinocembrin (2), 7,4′-Dihydroxy-5-methoxyflavanone (8), Demethoxyyangonin (157), and Pinostrobin chalcone (28). Utilizing predictive analysis, these metabolites have demonstrated stable interactions with key proteins, including matrix metallopeptidase 12 (MMP 12), cyclin-dependent kinase 4 (CDK 4), janus kinase 3 (JAK 3), vascular endothelial growth factor receptor 2 (VEGFR 2), matrix metallopeptidase 13 (MMP 13), vascular endothelial growth factor receptor 1 (VEGFR 1), and potassium voltage-gated channel subfamily A member 3 (KCNA 3). These interactions suggest a potential for these metabolites to inhibit proliferation and induce apoptosis in breast cancer cells. Additionally, in vitro assays have confirmed that B. rotunda extract exerts cytotoxic effects on T47D cells, initiating apoptotic pathways. On a global scale, colorectal cancer (CRC) remains one of the most prevalent forms of cancer, posing significant threats to human health and survival. Chuah et al. (2022) conducted a study examining the therapeutic efficacy of nanovesicles derived from fingerroot (FDNVs) in the context of CRC, uncovering significant anti-cancer activity against the CRC cell lines HT-29 and HCT 116. The treatment with 25 μg/mL of B. rotunda extract for a duration of 24 h led to pronounced cytotoxicity in all cell lines under investigation. Consistently, FDNVs elicited cytotoxic effects on both CRC cell lines in a dose- and time-dependent manner, with inhibitory concentration 50 (IC50) values of 47.8 and 34 μg/mL achieved after a 72 h exposure, respectively. Notably, FDNVs displayed no cytotoxicity towards normal human colonic epithelial cells. Moreover, FDNVs triggered early apoptosis in HT-29 and HCT116 cells in a dose-responsive fashion, upregulating the expression of cysteinyl asparaginase and the pro-apoptotic gene bcl-associated X protein (Bax), while downregulating the expression of the anti-apoptotic B-cell lymphoma-2 (Bcl-2) gene. These findings suggest a selective anti-cancer effect of FDNVs on CRC cells, highlighting their potential as a targeted therapeutic agent.
Additionally, Break et al. (2020) reported that Cardamonin (27), a chalcone metabolite derived from B. rotunda, exerted notable cytotoxic effects on Nasopharyngeal Carcinoma Cells (HK1), with an IC50 value of 26.7 mg/mL achieved after a 48 h treatment period. In stark contrast, the IC50 value against normal NP69 cells exceeded 200 mg/mL, highlighting a significantly higher selectivity for HK1 cells. This finding suggests a marked preference for cancer cells with minimal effects on normal cellular counterparts. Western blot analysis revealed that myricetin did not significantly modulate the expression levels of B-cell lymphoma-extra large (Bcl-XL), Bcl-2, or Bax, leading to the hypothesis that Cardamonin (27) induces apoptosis in HK1 cells via an extrinsic, rather than intrinsic, apoptotic pathway. Cheah et al. (2011) reported that Panduratin A (20), a bioactive metabolite isolated from B. rotunda, significantly inhibited the proliferation of A549 human non-small cell lung cancer cells. This metabolite exhibited cytotoxicity against these cells, with a half maximal IC50 of 4.4 μg/mL, which is equivalent to 10.8 μM. Further studies demonstrated Panduratin A’s (20) capacity to induce G2/M phase arrest in cancer cells, resulting in the accumulation of BrdU-labeled cancer cells and an increase in phosphorylated histone H3 levels. Simultaneously, Panduratin A (20) led to a decrease in cancer cell viability and an enhancement in plasma membrane permeability. These observations suggest a significant inhibitory effect on the activation of the nuclear factor-κB (NF-κB) signaling pathway, which may underlie its anti-cancer activity.
Melanoma, a highly aggressive cutaneous tumor originating from melanocytes, is characterized by its propensity for metastasis and its significant impact on patient prognosis. Lai et al. (2015) investigated the biological effects of Panduratin A (20), a cyclohexyl chalcone metabolite isolated from B. rotunda, on human malignant melanoma A375 cells. Preliminary findings revealed that Panduratin A (20) possessed cytotoxic activity against A375 cells, with an IC50 value of 11.66 μM. Furthermore, the primary target of Panduratin A (20) in A375 cancer cells was identified as the mitochondria, suggesting its role in modulating mitochondrial oxidative phosphorylation, secretion, and endoplasmic reticulum stress pathways. The metabolite was found to induce apoptosis through sustained endoplasmic reticulum stress via the PERK/eIF2α/ATF4/CHOP signaling cascade.
The flavonoid and polyphenol metabolites present in extracts of B. rotunda have demonstrated potent inhibitory effects on tumor and cancer cells. Experimental data have underscored the therapeutic potential of B. rotunda in addressing breast, skin, and digestive system malignancies. Nonetheless, the correlation between the chemical structures of these metabolites and their biological activities is an area that is not well-explored, and the precise mechanisms underlying their anti-cancer effects remain to be fully elucidated. Furthermore, the innovative role of these chemical metabolites in the specific targeting of tumors or cancer cells warrants additional investigation. Future research endeavors should concentrate on deciphering the structure-activity relationship of these metabolites, thoroughly examining the bioactivity of flavonoid and polyphenol metabolites, and dissecting their mechanisms of action to augment their therapeutic efficacy and specificity.
Bioactive flavonoids, isolated from B. rotunda, manifest a range of pharmacological properties, notably the ability to avert kidney injury, thereby positioning them as traditional nephroprotective agents. In the investigation conducted by Sujana et al. (2024), the nephroprotective efficacy of the ethanolic extract of B. rotunda rhizome (EEBR) was assessed across dosages of 125, 250, and 500 mg/kg BW. Comparative analysis with the negative control group revealed a significant reduction in serum creatinine, urea nitrogen, glutamic pyruvic transaminase, and malondialdehyde levels. Furthermore, the cisplatin-induced upregulation of renal cortex genes, including kidney injury molecule 1 (KIM-1), neutrophil gelatinase-associated lipocalin (NGAL), caspase 3, and caspase 7, in rats was substantially mitigated. In parallel, EEBR exerted no significant influence on body weight or the relative kidney weight percentage, thus bolstering its safety profile. Consistent with these findings, the natural flavonoid Pinostrobin (1), derived from B. rotunda, has been shown by Ijaz et al. (2023) to possess the ability to ameliorate renal injury in rats. Pinostrobin (1) was demonstrated to significantly mitigate cadmium-induced renal impairment by modulating cadmium-induced alterations in urea, creatinine, anti-oxidant enzyme activities, creatinine clearance, circulating enzymes of the tricarboxylic acid cycle, renal oxidative stress, apoptosis, and inflammation. These renoprotective effects are hypothesized to be mediated through Pinostrobin’s (1) anti-inflammatory, anti-apoptotic, and anti-oxidant properties. In a parallel study, Worakajit et al. (2022) explored the protective effects of Panduratin A (20), an active metabolite derived from B. rotunda, against mucin-induced nephrotoxicity in rats using both in vivo and in vitro methodologies. The experimental outcomes indicated that treatment with 2.5 and 25 mg/kg BW Panduratin A (20) effectively attenuated the increase in blood urea nitrogen (BUN) following visfatin administration, inhibited visfatin-induced apoptosis in renal tubular epithelial cells, and neutralized the mitochondrial damage provoked by visfatin in these cells. Additionally, Panduratin A (20) led to a downregulation in the expression of carbonyl proteins, cytochrome c, caspase 3, T cell immunoglobulin domain and mucin domain protein-1 (Tim-1), and NGAL, while concurrently upregulating the expression of Bcl-2 within renal tissues and renal proximal tubular cells. Thongnuanjan et al. (2021) employed the in vitro cultured human renal cell line RPTEC/TERT 1 to investigate the impact of Panduratin A (20) on cisplatin-induced toxicity. Their findings indicated that Panduratin A (20) ameliorates cisplatin-induced nephrotoxicity in both mice and RPTEC/TERT 1 cells by diminishing apoptotic cell death. The oral administration of Panduratin A (20) at a dosage of 50 mg/kg BW was found to enhance renal function and alleviate cisplatin-induced renal tubular damage. This salutary effect is believed to be mediated through the inhibition of the extracellular signal-regulated kinase (ERK) 1/2 and caspase-3 signaling pathways.
In summary, B. rotunda exhibits a significant capacity to safeguard renal health through the modulation of multiple renal function pathways. The plant has been shown to effectively lower blood levels of creatinine, urea nitrogen, glutamate pyruvate transaminase, and malondialdehyde, which suggests its considerable therapeutic potential in the treatment and prevention of kidney injury. Nonetheless, the precise mechanisms underlying the nephroprotective activity of B. rotunda extracts and metabolites remain partially elucidated, with several studies focusing primarily on pharmacodynamic assessments rather than delving into specific pathological mechanisms. Follow-up studies should further analyze how B. rotunda exerts its nephroprotective activity through in vivo and in vitro experiments, so as to explore the mechanism of the nephroprotective effect of B. rotunda. In addition, further toxicological and clinical studies are necessary to convert the remarkable activity of B. rotunda into clinical applications.
In their 2022 investigation, Jamornwan et al. (2022) evaluated the anti-inflammatory effects of Panduratin A (20), a metabolite isolated from B. rotunda, on lipopolysaccharide (LPS)-induced microglia activation in the spontaneous immune microglia (SIMA9) model. The study revealed that Panduratin A (20) displayed potent biological activity across a range of concentrations (0.1, 1, 5, 10, and 50 μM), reducing the levels of nitric oxide (NO) and p-NF-κB p65 in a concentration-dependent manner. Moreover, Panduratin A (20) significantly diminished the secretion of pro-inflammatory cytokines, including tumor necrosis factor-α (TNF-α), interleukin-1β (IL-1β), and interleukin-6 (IL-6), while markedly enhancing the release of anti-inflammatory cytokines, interleukin-4 (IL-4) and interleukin-10 (IL-10), from LPS-stimulated cells. Consequently, this resulted in an increased production and secretion of anti-inflammatory mediators, highlighting the immunomodulatory potential of Panduratin A (20) in the context of neuroinflammation. Mohan et al. (2020) studied the gastric protective effect of Boesenbergin A (35) isolated from the extract of B. rotunda by using ethanol-induced gastric ulcer model in rats. The tissue malondialdehyde (MDA) levels were significantly decreased by 14.01 and 7.13 μmol/g under the pretreatment of 10 and 20 mg/kg BW doses, respectively. In addition, Boesenbergin A (35) significantly restored elevated prostaglandin E2 (PGE2) levels while reducing NO and inducible NO synthase (iNOS) levels. Rosdianto et al. (2020) conducted a study to elucidate the anti-inflammatory mechanisms of the ethanol extract derived from B. rotunda. Their research demonstrated that the extract significantly reduced the infiltration of inflammatory cells in the gastric and intestinal tissues of rats subjected to acetic acid-induced inflammation. Additionally, the extract modulated the expression levels of key regulatory proteins, Akt and NF-κB p65. These findings provide a scientific basis for the potential application of B. rotunda extract in anti-inflammatory therapy and gastric protection, underscoring its therapeutic potential in mitigating inflammatory responses and safeguarding gastrointestinal health.
Pui Ping et al. (2020) explored the protective effects of Cardamonin (27), a metabolite derived from B. rotunda, against inflammation-mediated pain and injury. Their findings imply that the injury-preventive properties of Cardamonin (27) may be associated with its capacity to modulate protein kinase C (PKC) activity, N-methyl-D-aspartic acid (NMDA) and non-NMDA glutamate receptor function, the L-arginine/nitric oxide/cGMP signaling pathway, and ATP-sensitive potassium channels. Notably, Cardamonin (27) significantly suppressed acetic acid-induced pain responses in a dose-dependent manner at concentrations of 0.3, 1, 3, and 10 mg/kg BW, with corresponding inhibition rates of 45%, 56%, 80%, and 100%, respectively. These results suggest that the anti-inflammatory and analgesic effects of Cardamonin (27) may be attributed to its influence on PKC activity, glutamate receptor activity, and associated signaling pathways. In a comparable study, Ping et al. (2018) observed a significant dose-dependent inhibition of acetic acid-induced abdominal torsional pain following both intraperitoneal injection and oral administration of Cardamonin (27) at doses of 0.3, 1, 3, and 10 mg/kg BW. Concurrently, Cardamonin (27) demonstrated notable analgesic effects in models induced by formalin, capsaicin, and glutamate. However, the precise mechanisms underlying these anti-inflammatory and analgesic effects of Cardamonin (27) require further elucidation. Voon et al. (2017) conducted a study to investigate the pharmacological activity of Cardamonin (27) in a rheumatoid arthritis model, which was established by plantar injection of complete Freund’s adjuvant in Sprague-Dawley rats. Treatment with varying concentrations of Cardamonin (27), specifically 0.625, 1.25, and 2.5 mg/kg BW, resulted in a significant reduction in plasma concentrations of IL-6 and IL-1β. Notably, only the lowest dose of 0.625 mg/kg BW of Cardamonin (27) elicited a significant decrease in plasma TNF-α levels within the rheumatoid arthritis model. These results highlight the capacity of Cardamonin (27) to substantially suppress complete Freund’s adjuvant-induced inflammatory changes in the joints of rats with rheumatoid arthritis.
Furthermore, Boonyarattanasoonthorn et al. (2023) conducted a pharmacokinetic study of Panduratin A (20) in Beagle dogs, thereby establishing its oral safety and laying a scientific foundation for the potential use of botanical drugs based on B. rotunda extract in the context of COVID-19 pandemic management. In a related study, Kongratanapasert et al. (2023) reported that Panduratin A (20), extracted from B. rotunda, led to significant decreases in lung pathophysiology and inflammatory mediators, including PGE2 and IL-6, in hamsters infected with SARS-CoV-2. Notably, PA showed high bioavailability at dosages of 300 and 1,000 mg/kg BW. In a similar vein, Kanjanasirirat et al. (2020) demonstrated the potent anti-SARS-CoV-2 activity of Panduratin A (20) in Vero E6 cells, with an IC50 value of 3.62 μg/mL (CC50 = 28.06 μg/mL) and 0.81 μM (CC50 = 14.71 μM), indicating its potential as an anti-viral agent. These findings suggest that Panduratin A (20) possesses not only oral safety and high bioavailability but also exhibits potential dual efficacy as an anti-inflammatory and anti-viral agent. This dual functionality renders Panduratin A (20) a promising candidate for the therapeutic management of COVID-19 and associated inflammatory conditions.
In aggregate, a plethora of experimental studies have demonstrated a strong correlation between the anti-inflammatory activity of B. rotunda and its abundant flavonoid content. Notably, flavonoids such as panduratin A (20) have been proven to exert significant anti-inflammatory effects by inhibiting pivotal inflammatory mediators, including NO, PGE2, and TNF-α. Despite these findings, the extant research on the anti-inflammatory activities of B. rotunda is not sufficiently comprehensive to elucidate the underlying mechanisms fully. Consequently, there is a clear imperative for subsequent, more profound investigations into the anti-inflammatory mechanisms of B. rotunda. Moreover, flavonoids have garnered considerable interest due to their potential in drug discovery. The precise modification of these flavonoids through chemical synthesis or targeted molecular approaches could enhance their therapeutic precision and efficacy. Such advancements could pave the way for the development of novel pharmaceuticals with augmented potency and selectivity. Therefore, it is essential to delve deeper into the study of flavonoids in B. rotunda to harness their full therapeutic potential and contribute significantly to medical advancement.
The anti-bacterial and anti-viral properties of B. rotunda have been well-documented in literature. In a particular study, Thadtapong et al. (2024) delineated the potency of a novel flavonoid, extracted from B. rotunda, in impeding biofilm formation of two notable strains, Aci46 and Aci44. Additionally, they illustrated the capacity of panduratin A (20), at concentrations of 2.5 and 5 μM, to potentiate the activity of colistin. This revelation underscores the potential of panduratin A (20) to function as an adjuvant for mucilage, augmenting the efficacy of existing anti-microbial agents. More recently, Apinundecha et al. (2023) explored the combined anti-microbial effects of B. rotunda essential oil (BREO) and cloxacillin. Their findings indicated that BREO possessed notable anti-bacterial properties, capable of decreasing the activity of Staphylococcus aureus, with a minimum inhibitory concentration (MIC) of 2 mg/mL.
Biofilm formation is a key virulence factor associated with the opportunistic fungal pathogen Candida albicans. The principal metabolites of B. rotunda extract, Pinostrobin (1) and Pinocembrin (2), have been shown to substantially inhibit biofilm formation. Kanchanapiboon et al. (2020) reported that both the extract and Pinocembrin (2) significantly reduced cell surface hydrophobicity and downregulated ALS3 mRNA expression levels. The extract exerted a significant inhibitory effect on biofilm development, with an IC50 value of 17.7 μg/mL, indicating its potency. In parallel, B. rotunda extract displayed considerable inhibitory activity against beta-lactam-resistant strains of S. aureus and Staphylococcus epidermidis. Teethaisong et al. (2018) elucidated the mechanism of action, which involves interaction with the bacterial cytoplasmic membrane, leading to membrane disruption and subsequent leakage of intracellular contents. Blastocystis hominis, a protozoan parasite, can cause a spectrum of gastrointestinal symptoms in humans and animals. Kaewjai et al. (2023) determined that at a MIC of 62.5 μg/mL, B. rotunda extract significantly suppressed the growth of Blastocystis hominis, inducing cellular crumpling and exhibiting significant anti-protozoal activity.
To date, the majority of research has concentrated on the potent in vitro anti-bacterial effects of B. rotunda, with a relative scarcity of in vivo studies. The adverse reactions and potential for drug resistance associated with B. rotunda remain undetermined, and an accurate assessment of drug interactions, their subsequent reactions, and effects within biological systems is yet to be fully realized. It is essential to further investigate the in vivo responses, anti-bacterial mechanisms, and physiological effects of B. rotunda to gain a comprehensive understanding of its therapeutic potential and biological interactions. This includes examining how B. rotunda not only inhibits or eradicates certain bacteria but also modulates immune responses, thereby enhancing anti-bacterial efficacy through various mechanisms, the precise role of which remains to be elucidated. Consequently, an in-depth study of the in vivo responses, anti-microbial mechanisms and physiological effects of B. rotunda is essential for a comprehensive understanding of its therapeutic potential and biological interactions.
Obesity is a critical factor in the development of chronic metabolic syndromes. The metabolites Panduratin A (20), Cardamonin (27), and Isopanduratin A (21), all of which are derived from B. rotunda, have been identified as potent anti-obesity agents. These metabolites demonstrate both anti-adipogenic and lipolytic activities, highlighting their potential in the management of obesity-related metabolic disorders. Rungsa et al. (2023) conducted a study revealing that isopanduratin A (21), a metabolite of B. rotunda, potently inhibited adipogenesis by specifically targeting the mitogen-activated protein kinase (MAPK) pathway and modulating the AKT/GSK3β and AKT/AMPK-ACC signaling cascades. Notably, isopanduratin A (21) impeded ERK phosphorylation during the initial phase of adipogenesis, thereby suppressing mitotic clonal expansion. This distinctive attribute has not been observed for Pinostrobin (1) and, to date, has not been reported for Panduratin A (20) or Cardamonin (27), underscoring the unique potential of isopanduratin A (21) in the context of anti-obesity therapeutics. San et al. (2022) conducted a study demonstrating that Panduratin A (20), at concentrations above 5 μM, significantly reduced both cell viability and triglyceride levels. Notably, Panduratin A (20) did not affect mitotic clonal expansion during adipogenesis. At concentrations between 5 and 20 μM, Panduratin A (20) markedly decreased the mRNA expression levels of key adipogenic factors CCAAT/enhancer-binding protein α (C/EBPα), peroxisome proliferator-activated receptor γ (PPARγ), and sterol regulatory element binding protein-1c (SREBP-1c), along with the protein expression of phosphorylated protein kinase B (p-Akt), phosphorylated c-jun N-terminal kinase (p-JNK), and phosphorylated p38 mitogen-activated protein kinase (p-p38). Compared to the control group, a significant reduction in the p-JNK/JNK ratio was observed at the lower concentration of 5 µM of Panduratin A (20), while the suppression of the p-p38/p38 ratio was evident at higher concentrations. The accumulation of cellular lipid droplets, as assessed by the oil red O staining assay, showed reductions to 92.3%, 85.4%, and 51.2% following treatment with 5, 10, and 20 µM of Panduratin A (20), respectively. This indicates a substantial decrease in lipid droplet accumulation in cells treated with Panduratin A (20). In a related study by Jitsaeng et al. (2023), Panduratin A (20), isolated from fraction 3 (BP-3) of the ethanolic extract of B. rotunda, was found to exhibit anti-adipogenic and lipolytic activities. In their study, the BP-3 extract, containing 0.29 g/g extract of Panduratin A (20), was non-toxic to cells and demonstrated more potent anti-adipogenic and lipolytic effects on 3T3-L1 preadipocyte cells at a concentration of 10 μg/mL. Furthermore, Chatsumpun et al. (2017) characterized a range of metabolites in B. rotunda extract that exhibit inhibitory activities against α-glucosidase and pancreatic lipase, with biflavones and chalcones being the predominant metabolites. Potipiranun et al. (2018) observed the inhibition of glycosylation and α-glucosidase by metabolites derived from B. rotunda. From the dichloromethane extract, three flavanones, two chalcones, two dihydrochalcones, and one narcotic piperolactone were isolated. These metabolites demonstrated more potent inhibition of advanced glycation end products compared to aminoguanidine. It was also observed that activity varied among different structural types. Metabolites featuring hydroxyl and α-β unsaturated ketone structures showed greater activity, whereas metabolites with methoxy and geranyl groups exhibited reduced activity. Notably, the presence of a methoxy group at the C-4 position of dihydrochalcone was more effective than that at the C-6 position. Additionally, Pinocembrin (2) showed moderate inhibitory effects against maltase and sucrose, with IC50 values of 0.35 mM and 0.39 mM, respectively. These findings underscore the structure-activity relationships among the metabolites of B. rotunda and their potential implications in the management of diabetes and obesity.
B. rotunda has been shown to have a significant ability to inhibit lipogenesis, suggesting that it could be used in foods and drugs for the prevention and control of obesity. However, current studies are mainly limited to a few metabolites and further exploration of other metabolites or extracts is scarce. Meanwhile, the identified lipogenesis inhibitory activities have not been thoroughly investigated and detailed mechanistic studies are lacking, thus preventing a precise assessment of the anti-obesity mechanism of Verticillium rolfsii. Therefore, it is important to thoroughly grasp the mechanism of action of B. rotunda and comprehensively assess the therapeutic potential of B. rotunda, thereby laying a solid foundation for safe and effective translation into clinical practice.
The hepatoprotective effects of the chalcone Panduratin A (20) were assessed in a rat model of liver injury induced by intraperitoneal administration of thioacetamide (TAA). In a study conducted by Salama et al. (2018), the protective effects of B. rotunda extracts on liver cirrhosis were investigated. The findings revealed that Panduratin A (20) substantially decreased the severity of liver injury, activation of hepatic stellate cells (HSCs), and collagen deposition. Furthermore, Panduratin A (20) significantly elevated the levels of superoxide dismutase (SOD), catalase (CAT), and glutathione peroxidase (GPx), thereby establishing its hepatoprotective mechanism through the inhibition of HSC activity. In a distinct study, Salama et al. (2013) established a liver injury model in SD rats by intraperitoneal injection of 200 mg/kg BW TAA. The research demonstrated that the oral administration of B. rotunda extract exerts a significant protective effect against liver injury in rats. Histopathological examination of the rat livers revealed marked amelioration following B. rotunda extract intervention. Furthermore, B. rotunda extract was found to reduce the levels of liver nitrotyrosine and urine 8-OH-dG, enhance the activities of CAT and GPx, and promote hepatocyte survival. The primary mechanisms by which B. rotunda extract ameliorates liver injury in rats encompass the inhibition of fibrotic factors such as transforming growth factor beta 1 (TGF-β1), NF-κB, and IL-6, upregulation of caspase-1 expression, elevation of serum levels of Bax and caspase-3, and suppression of extracellular matrix proteins and hepatocyte proliferation.
The hepatoprotective significance of B. rotunda is increasingly acknowledged, particularly for its ability to curb the proliferation of fibrotic cells and to trigger apoptosis. Despite this, research dedicated to harnessing its potential in the prevention and treatment of liver injury remains sparse, with a notable absence of mechanistic studies. Considering the multifaceted origins of hepatocellular injury, the mechanisms and pathologies are highly heterogeneous, and thus further refinement of its therapeutic mechanism of action is needed. A comprehensive understanding of the therapeutic mechanisms of B. rotunda is essential to effectively translate the potential of B. rotunda into clinical practice and to ensure the safety and efficacy of liver disease treatment.
The anti-oxidant potency of chalcone Panduratin A (20) was assessed by Salama et al. (2013) using 2,2-Diphenyl-1-picrylhydrazyl (DPPH) free radicals, ferric reducing power, and a normal embryonic cell line WRL-68. The experimental results demonstrated that Panduratin A (20) harbors anti-oxidant properties, with a dose-dependent decrease in DPPH radical content and an increase in iron-reducing power activity. Additionally, it was observed that Panduratin A (20) significantly reduced MDA levels and bolstered the activities of SOD, CAT, and GPx, thereby further enhancing cellular anti-oxidant defenses. Abdelwahab et al. (2011) conducted a study on the methanol extract of B rotunda and its principal metabolite, Pinostrobin (1), to evaluate their anti-ulcer and anti-oxidant activities against ethanol-induced gastric ulcers. The experimental results indicated that the extract offered protection to the gastric mucosa, as evidenced by a reduction in ulcer area and mucosal damage, along with the mitigation or resolution of submucosal edema and leukocyte infiltration. Additionally, in vitro assessments revealed that the extract significantly suppressed the production of NO by LPS/IFN-γ-activated rodent cells, while showing no inhibitory effect on cyclooxygenase-1 (COX-1) and cyclooxygenase-2 (COX-2). In a subsequent study, Isa et al. (2012) assessed the anti-oxidant activity of B. rotunda using the oxygen radical absorbance capacity assay, with Quercetin (6) serving as a standard anti-oxidant reference. Both B. rotunda at a concentration of 20 μg/mL and Quercetin (6) at 5 μg/mL, equivalent to 11.91 and 160.32 µM Trolox concentrations, respectively, exhibited robust anti-oxidant activity by inhibiting the COX-2 enzyme.
At present, research on B. rotunda has demonstrated promising anti-oxidant activity, garnering significant interest within the scientific community due to its implications for therapeutic applications. Nonetheless, the current body of research is limited, particularly with regard to in vivo studies, and there is a pressing need for more comprehensive investigations to elucidate the mechanisms underlying the anti-oxidant effects of its metabolites. These studies are crucial for advancing our comprehension of B. rotunda’s pharmacological activity and for optimizing its utilization in the development of pharmaceuticals.
Pinostrobin (1), a naturally occurring bioflavonoid metabolite derived from the rhizomes of B. rotunda, has been the subject of scientific scrutiny. In a study conducted by Kongsui et al. (2023), Parkinson’s disease rat models were administered a dosage of 40 mg/kg BW Pinostrobin (1), which resulted in a marked reduction of free radicals within the substantia nigra, a significant decrease in MDA levels, and a substantial increase in anti-oxidant enzyme activities, namely, SOD and GSH. These biochemical changes corresponded with an improvement in the locomotor function of the rats, indicative of a therapeutic effect. In a parallel investigation, Thongrong et al. (2023) determined that Pinostrobin (1) mitigated chronic stress-induced cognitive deficits and averted brain dysfunction by exhibiting anti-oxidant properties that diminished neuronal cell damage and bolstered the functionality of astrocyte markers, glial fibrillary acidic protein (GFAP), and excitatory amino acid transporter 2 (EAAT2). Pinostrobin (1), a bioflavonoid metabolite isolated from B. rotunda, has demonstrated anti-oxidant properties and the capacity to facilitate the repair of peripheral nerve damage. In a study by Kongsui et al. (2023), male Wistar rats with sciatic nerve crush injuries were subjected to treatment with Pinostrobin (1) at dosages of 20 and 40 mg/kg BW to assess its reparative effects. The findings indicated that Pinostrobin (1) treatment at both concentrations mitigated oxidative stress by increasing endogenous glutathione levels. This upregulation led to an enlargement of axon diameter, an increase in the count of dorsal root ganglion (DRG) neurons, and an enhanced expression of phosphorylated extracellular signal-regulated kinase 1/2, thereby promoting the recovery of sciatic nerve functionality. Collectively, the study suggests that Pinostrobin (1) could serve as an effective therapeutic strategy for the treatment of peripheral nerve injuries. Furthermore, Wang et al. (2022) reported in their study that the B. rotunda extract possesses anti-inflammatory and analgesic effects. Diabetic peripheral neuropathy was induced in SD rats by administering a combination of 30% fructose solution and streptozotocin. Throughout the experiment, the administration of B. rotunda extract at dosages of 100 and 400 mg/kg BW significantly reduced blood glucose levels and attenuated weight loss in diabetic rats, demonstrating a hypoglycemic effect. Additionally, the analysis of serum pro-inflammatory cytokines revealed that the concentrations of TNF-α and IL-1β in the serum of diabetic rats treated with B. rotunda extract were significantly lower compared to the normal control group, with the reduction exhibiting a dose-dependent pattern.
Targeting angiogenesis represents a compelling and promising therapeutic strategy in oncology. Adhikari et al. (2020) revealed that B. rotunda extract elicited relaxation in coronary artery rings via both endothelium-dependent mechanisms, such as the nitric oxide-cGMP pathway, and endothelium-independent mechanisms, including the blockade of Ca2+ channels. Furthermore, flavonoids with vasorelaxant activity were isolated and characterized from the active fractions, comprising Naringenin 5-methyl ether (5), Alpinetin (3), pinocembrin (2), Pinostrobin (1), and 4-hydroxypanduratin A (22). These metabolites all demonstrated significant vasodilatory effects, although the precise mechanisms responsible for these actions remain to be fully elucidated. Additionally, Bereswill et al. (2012) investigated the anti-angiogenic properties of the natural chalcone Panduratin A (20), originating from B. rotunda. This was substantiated through both in vitro and in vivo studies. In vitro evaluations indicated that Panduratin A (20) displayed concentration-dependent cytotoxicity and growth inhibition in human umbilical vein endothelial cells, distinct from its effects on normal human fibroblasts and liver epithelial cells, with an IC50 of 6.91 mM. In vivo, the inhibitory effect of Panduratin A (20) on neointimal formation was confirmed using the mouse Matrigel plug assay and the zebrafish angiogenesis model, thereby highlighting its potential as an anti-angiogenic agent. Ruttanapattanakul et al. (2022) characterized Pinocembrin (2) as an active metabolite exerting potent stimulatory effects on human keratinocytes. Following a 15 min exposure to Pinocembrin (2) at concentrations of 15.6, 31.3, and 62.5 μM, the activation of two key kinases, ERK1/2 and Akt, was rapidly triggered in the individual cells. This finding underscores the potential utility of plants and natural products rich in Pinocembrin (2) as viable alternatives for skin regeneration and wound healing therapies.
In summary, B. rotunda exhibits potential in mitigating heat-induced pain, cold-induced pain, and mechanical allodynia in diabetic rats, while concurrently enhancing motor coordination through its anti-oxidant properties, reducing neuronal damage, and upregulating astrocyte marker functions such as GFAP and EAAT2. Furthermore, it demonstrates anti-cancer activity by suppressing cancerous lesions and promoting overall bodily function, with a particular impact on angiogenesis. As a result, B. rotunda presents itself as a promising candidate for use as an adjunct therapy or a source of pharmaceutical leads in the therapeutic management of a spectrum of conditions, including Alzheimer’s disease, Parkinson’s disease, neurotraumatic injuries, and neoplastic diseases. Subsequently, a thorough investigation of these activities is warranted to elucidate their underlying mechanisms of action, thereby contributing to advancements in the field of medicine.
The quality standardization of medicinal products is designed to ensure the consistency of quality and composition in traditional Chinese medicine, thereby ensuring the reliability of its clinical efficacy (Wu et al., 2018; Wang et al., 2024). Currently, the analysis of active metabolites in B. rotunda primarily focuses on flavonoids. B. rotunda is rich in a variety of flavonoids. Ruttanapattanakul et al. (2021) employed high-performance liquid chromatography to analyze the metabolite profile of various B. rotunda extracts, identifying kaempferol (4) as a predominant bioactive metabolite. Their methodology demonstrated precision and stability in quantifying kaempferol content. Furthermore, complementary chromatographic analyses of the ethyl acetate fraction of B. rotunda by other researchers revealed eight flavonoids, which are considered the signature chemical metabolites characteristic of B. rotunda (Adhikari et al., 2020; Kanchanapiboon et al., 2020) determined the chemical metabolites of the extract by UPLC. Two flavonoids, Pinostrobin (1) and Pinocembrin (2), were determined from the extract. The contents of the two metabolites were 25.2% and 11.6%, respectively. Another study utilized LC-MS to quantify Quercetin (6) in the ethanol extract of B. rotunda rhizomes, establishing a method for its determination and confirming the presence of Quercetin (6) (Rosdianto et al., 2020). Additionally, Yuliana et al. (2013) utilized a comprehensive extraction-nuclear magnetic resonance metabolomics approach to identify adenosine A1 receptor-binding metabolites in the rhizome extract of B. rotunda. Their study led to the discovery of two flavonoids, pinocembrin (2) and hydroxy-panduratin (49). This methodology facilitates the expedited identification of bioactive metabolites from plant extracts, circumventing the requirement for prior purification.
From a biological perspective, the identification of B. rotunda has emerged as a focal point of new research, offering a fresh direction in its characterization. Researchers applied PCR technology to conduct an exhaustive examination of the entire chloroplast genome of B. rotunda. Notably, within the large single-copy (LSC) and small single-copy (SSC) regions of the chloroplast genome, they discovered multiple highly polymorphic regions, such as psbK-psbI, trnT-GGU-psbD, rbcL-accD, ndhF-rpl32, and ycf1. Due to their high polymorphism, these regions can serve as molecular markers for DNA barcoding and species delineation, thereby providing a crucial reference for the identification of B. rotunda (Liew et al., 2022).
The establishment and enforcement of quality standards for B. rotunda are instrumental in advancing the standardization of production and distribution processes, thereby fostering the robust growth of the traditional Chinese medicine sector. Given its dual role as both a medicinal and edible resource, the formulation of stringent quality benchmarks is of paramount importance. At present, the quality standards for B. rotunda predominantly concentrate on flavonoid content, with research into quality criteria for other metabolites remaining comparatively scarce. Consequently, there is a critical need to augment and refine the quality assessment parameters to ensure comprehensive quality assurance for B. rotunda.
Pharmacokinetics is dedicated to elucidating the dynamic processes of drug disposition within the human body, providing a crucial scientific foundation for the realization of personalized therapeutic regimens, as well as for ensuring the efficacy and safety of drug treatments (Li et al., 2020; Feng et al., 2024). Boonyarattanasoonthorn et al. (2023) investigated the pharmacokinetic profile of Panduratin A (20) in beagles. They found no statistically significant differences between two pharmacokinetic parameters following intravenous administration of 1 mg/kg BW Panduratin A (20) and multiple oral doses of 5 and 10 mg/kg BW of B. rotunda extract formulations. Whereas, Cmax and area under the curve (AUC) of the B. rotunda extract preparations were positively correlated with the dose of the oral drug. The conversion of Panduratin A (20) to other metabolites primarily occurs through oxidative and glucuronidation pathways, while its elimination from the body mainly occurs via the fecal route. This indicates the safety of both intravenous administration of the pure metabolite and oral administration of B. rotunda extracts. The findings of the study indicate that the pharmacokinetic profile of Panduratin A (20) closely resembles that of orally administered B. rotunda extracts. Certain researchers utilized LC-MS to quantify the levels of Panduratin A (20) in the plasma and lungs of hamsters infected with SARS-CoV-2 after treatment with B. rotunda extracts. They observed a dose-dependent increase in its levels. Specifically, at a dosage of 1,000 mg/kg BW, the concentration of Panduratin A (20) attained values ranging from 7,000 to 9,000 μg/L in plasma and 1,500–2,500 μg/L in lung tissues, leading to a significant reduction in lung inflammation and cytokine levels. However, pharmacokinetic studies of B. rotunda remain quite limited.
In conclusion, the pharmacokinetic profile of B. rotunda is crucial for the development and optimization of its pharmaceutical applications. From the results of the current study, it was found that there are still inherent limitations, fewer pharmacokinetic studies and lack of systematic pharmacokinetic studies. Future studies should focus on elucidating the absorption kinetics of the active metabolites in B. rotunda, including the rate and extent of absorption. It is also important to explore the distribution characteristics of these metabolites in vivo, to assess their concentrations in different tissues and organs, and to determine whether there is a specific targeting tendency. As well as studies on the metabolic and excretory pathways of the active metabolites and their derivatives. These will greatly contribute to the clinical application of B. rotunda and provide some help to the drug research of B. rotunda.
As previously noted, B. rotunda possesses a venerable history of utilization in China, serving as a valued and reputedly safe traditional Chinese medicine as well as a ubiquitous dietary staple. While the literature on the toxicity and safety assessment of B. rotunda is somewhat scarce, ongoing surveillance of its usage is warranted. Notably, Panduratin A (20) and Pinostrobin (1), metabolites isolated from B. rotunda, have been documented to exhibit no clinical signs of toxicity, morbidity, or mortality following continuous administration over a 90-day period at dosages of 25, 50, or 100 mg/kg BW (Techapichetvanich et al., 2024). Saah et al. (2024) employed freeze-drying technology to encapsulate B. rotunda extract and investigated the impact of maltodextrin (MD), arabic gum (GA), and their blends (MDGA) with various wall materials on the biological activity of the encapsulated product. The study demonstrated that the incorporation of MDGA as a carrier material mitigated the cytotoxicity associated with MDGA-5 and MDGA-7 formulations, concurrently enhancing the viability of MC3T3-E1 cells and the activity of alkaline phosphatase. These findings suggest that the MDGA wall material not only augments the biological efficacy of the B. rotunda extract but also contributes to the preservation of the stability of its bioactive metabolites. Consequently, this encapsulation technique is projected to be instrumental in the development of therapeutic and nutritional products designed for the management or prevention of osteoporosis. Ruttanapattanakul et al. (2022) performed cytotoxicity assays of Pinocembrin (2) in immortalised human keratinogenic HaCaT cells and showed that Pinocembrin (2) between 15.6 and 125 µM presented a significant increase in cell viability/cell proliferation. However, 500 µM was the only Pinocembrin (2) concentration that significantly reduced HaCaT cell viability. In addition, Panduratin A (20), isolated from the ethanol extract fraction 3 (BP-3) of B. rotunda, was further studied. The findings revealed that the BP-3 extract contained a notably high amount of Panduratin A (20). At concentrations below 10 μg/mL, it exhibited no cytotoxicity towards 3T3-L1 cells, yet demonstrated potent anti-lipogenic and lipolytic activities at this same concentration range (Jitsaeng et al., 2023).
Most current studies primarily focus on short-term effects, with limited investigation into long-term chronic toxicity. Additionally, there is a notable absence of comprehensive assessments of organ-specific toxic effects, which are essential for understanding potential side effects in clinical use. Furthermore, more detailed dose-response studies are necessary to precisely determine the threshold between therapeutic and toxic doses. Future research should include acute, subacute, and chronic toxicity studies to fully understand the safety profile of B. rotunda. Establishing a standardized toxicity test protocol will ensure consistency and comparability across various studies. Additionally, exploring the potential synergistic toxic effects of B. rotunda when combined with other drugs or supplements is crucial. Moreover, long-term clinical trials should be initiated to evaluate the safety and efficacy of B. rotunda in real-world settings, providing valuable data for its clinical application (Wang et al., 2024). To explore the effects of long-term exposure to different dose levels, and to develop better predictive models to evaluate the safety factor. Such a systematic investigation will provide a solid scientific basis for the safe and effective utilization of B. rotunda in clinical settings.
At present, although it is found that B. rotunda has good biological activity in vitro and in vivo, there is a lack of certain clinical data, and there are relatively few related clinical studies. This situation limits our comprehensive understanding of the efficacy and safety of B. rotunda. Jitsaeng et al. (2023) selected 13 healthy volunteers to investigate the anti-lipogenesis and lipolysis effects of Panduratin A (20) microneedle serum. The volunteers applied the microneedle serum containing Panduratin A (20) to their thigh skin and gently massaged the area for 14 days. The results indicated a decrease in thigh circumference, along with increased skin hydration and firmness after treatment, suggesting its potential as a lipolysis agent. In addition, a single-blind, randomized, cross-over study evaluated the preventive and alleviating effects of a traditional Chinese medicine mouthwash containing B. rotunda extract on radiation-induced oral mucositis. A total of 120 patients undergoing radiotherapy for head and neck cancer were randomly divided into three treatment groups receiving normal saline, sodium bicarbonate, or the traditional Chinese medicine extract gargle. The therapeutic effects were assessed using mucositis score and body mass index. The results showed that the traditional Chinese medicine mouthwash could slightly delay the onset of symptoms, with an average mucositis score of less than two across all groups and no significant difference in body mass index. These findings suggest that the traditional Chinese medicine gargle may serve as an effective alternative to bicarbonate and normal saline for the preventive treatment of radiation-induced oral mucositis (Kongwattanakul et al., 2022). Chitapanarux et al. (2021) conducted a 4-week randomized, double-blind, placebo-controlled study to assess the effectiveness and safety of B. rotunda extract in individuals with functional dyspepsia. The outcomes indicated that patients receiving B. rotunda extract experienced significant alleviation in symptoms such as dyspepsia, heartburn, and reflux. Furthermore, the treatment group demonstrated superior overall symptom improvement compared to the placebo group. In addition, the level of blood inflammatory markers in the treatment group was reduced, showing an inhibitory effect on gastric body inflammation. These findings suggest that B. rotunda extract may be an effective method for the treatment of functional dyspepsia.
The above studies have provided valuable insights into the pharmacological effects of B. rotunda in humans, thereby expanding the range of clinical therapeutic options. Although modern pharmacological studies have demonstrated that B. rotunda possesses a wide range of biological activities, they have failed to translate into clinical applications, and the scarcity of clinical trials has limited the full realization of B. rotunda’s potential. Therefore, there is an urgent need to further investigate the multiple pharmacological activities of B. rotunda in clinical practice.
Bibliometric studies are instrumental in elucidating research trends, identifying hotspots, and charting developmental pathways within a delineated academic sphere, thereby providing valuable insights for strategic disciplinary planning. In this regard, we have executed a bibliometric analysis of the existing scholarly literature concerning B. rotunda. A comprehensive bibliometric analysis of the scientific literature on B. rotunda has uncovered that this species possesses a rich historical utilization in traditional medicinal practices and exhibits significant promise in modern scientific inquiry. Notably, this potential has been markedly exemplified during the preceding decade (Figure 8A), with a notable escalation in the volume of research on B. rotunda. This trend underscores a burgeoning surge in scholarly attention and interest towards B. rotunda. To enhance the comprehension of research hotspots and trends concerning B. rotunda, we have delineated a network visualization map encompassing all co-occurring keywords. This network diagram elucidates the pivotal themes and interconnections within the research landscape of B. rotunda. Flavonoids, which are the predominant bioactive metabolites in B. rotunda, have emerged as a focal point of investigation. These metabolites are hypothesized to possess therapeutic potential across a spectrum of diseases (Figure 8B).
Figure 8. B. rotunda in the number of publications from 2002 to 2024 (A), network visualization map of all keywords co-occurrence for B. rotunda (B).
In summary, there has been a marked escalation in scholarly interest towards B. rotunda in recent years, underscoring its potential as a focal point for contemporary scientific inquiry. It is imperative to conduct subsequent in-depth pharmacological studies to substantiate the therapeutic efficacy of flavonoid metabolites present in B. rotunda. Concurrently, the exploration and development of other metabolites derived from B. rotunda are anti-cipated to pave the way for novel drug discovery and development paradigms.
The present manuscript provides an exhaustive overview of the results of scholarly research on the traditional applications, phytochemical metabolites, pharmacological properties and clinical applications of B. rotunda. Screening of existing studies on B. rotunda through various databases, screening and analysis of the literature revealed the identification and isolation of more than 200 chemical metabolites from different parts of B. rotunda, of which flavonoids are the most prominent bioactive metabolites. These flavonoids have significant biological activities and have been incorporated into clinical studies. In addition, the study of the multiple bioactivities of B. rotunda provides a solid foundation for its further development and lays the groundwork for its potential clinical applications.
While the existing body of research on B. rotunda has made significant strides, there are several areas that require further exploration and deepening: (1) The majority of activity studies have concentrated on specific extracts and flavonoids, with the bioactivity of other metabolites being relatively uncharted. A more exhaustive investigation into the bioactivity of B. rotunda is essential to maximize its clinical potential. Compile a comprehensive list of all known metabolites in B. rotunda, focusing on those that have not been extensively studied. Further study of the mechanism of action of key metabolites, exploration of synergistic effects with other therapeutic agents, and development of new drug delivery systems. (2) Although over 200 metabolites have been identified in B. rotunda, research on these metabolites remains limited. The bioactivity of monomeric metabolites has primarily focused on a select few, particularly flavonoids. Future research should aim to uncover the potential value of other metabolites and fully exploit the therapeutic potential of B. rotunda. (3) Quality control studies on B. rotunda have predominantly focused on flavonoids, with less emphasis on other metabolites. Enhanced quality control measures are needed to ensure comprehensive surveillance of all B. rotunda metabolites. Additionally, biological identification techniques can augment quality control efforts, and there is a need to strengthen research in this domain. Incorporate advanced analytical techniques such as LC-MS/MS and NMR to detect and quantify a broader range of metabolites in B. rotunda extracts. (4) There is a dearth of pharmacokinetic studies on B. rotunda, and further research into the pharmacokinetics of its principal active substances and extracts is imperative. Such studies are vital for informing clinical drug therapy. Start with animal models to establish pharmacokinetic parameters, followed by human studies to validate these findings and assess the pharmacokinetics in a clinical setting. Use advanced pharmacokinetic modeling and simulation tools to analyze the data and predict the behavior of B. rotunda metabolites in the body. (5) A comprehensive toxicological evaluation of B. rotunda is necessary to assess its safety. While most research has focused on in vitro toxicology of various extracts and metabolites, in vivo studies are notably scarce. Future research must expand the assessment of both in vitro and in vivo systemic toxicity and safety profiles. Conduct in vivo toxicological studies, including acute, subacute, and chronic toxicity tests, to evaluate the safety profile of B. rotunda in animal models. Expand the assessment to include organ-specific toxic effects and systemic toxicity to provide a comprehensive understanding of the safety risks associated with B. rotunda. (6) Ultimately, the development of B. rotunda-based drugs must be anchored in clinical practice. Despite its myriad biological activities, clinical research on B. rotunda is still limited. Therefore, prioritizing follow-up clinical studies on B. rotunda is crucial to ensure its effective translation into clinical applications. Identify and recruit eligible participants for the clinical trials, ensuring a diverse and representative sample population. Implement rigorous data monitoring and analysis protocols to ensure the reliability and validity of the clinical trial results, and use the findings to inform the development of effective clinical applications for B. rotunda. These can further improve the research on B. rotunda and thus promote the development of B. rotunda in the field of medicine and food.
Overall, B. rotunda occupies an important position in the field of medicine and food. Nevertheless, research on Luo Han Guo is still fragmented and incomplete, and some of its metabolites and activities have yet to be fully explored and utilized. Future research should focus on understanding the areas of pharmacology, clinical studies and pharmacokinetics. The results of this study comprehensively summarize the relevant literature and discuss the current state of research on B. rotunda, providing critical insights and inspiration for subsequent research efforts. These are essential to promote the comprehensive development and utilization of B. rotunda.
YW: Writing–original draft. JW: Data curation, Writing–original draft. FL: Investigation, Writing–original draft. XP: Methodology, Writing–original draft. GX: Software, Writing–original draft. MZ: Conceptualization, Writing–review and editing. ZH: Writing–review and editing.
The author(s) declare that financial support was received for the research, authorship, and/or publication of this article. This work was finically supported by the National Natural Science Foundation of China (No. 82274105, 82274095) and the Key Industry Innovation Chain Project of Key Research and Development Program of Shaanxi Province (No. 2022ZDLSF05-09).
Authors ZH and FL are researchers at Shaanxi Buchang Pharmaceutical Co. Ltd.
The remaining authors declare that the research was conducted in the absence of any commercial or financial relationships that could be construed as a potential conflict of interest.
The author(s) declare that no Generative AI was used in the creation of this manuscript.
All claims expressed in this article are solely those of the authors and do not necessarily represent those of their affiliated organizations, or those of the publisher, the editors and the reviewers. Any product that may be evaluated in this article, or claim that may be made by its manufacturer, is not guaranteed or endorsed by the publisher.
Abdelwahab, S. I., Mohan, S., Abdulla, M. A., Sukari, M. A., Abdul, A. B., Taha, M. M. E., et al. (2011). The methanolic extract of Boesenbergia rotunda (L.) Mansf. and its major compound pinostrobin induces anti-ulcerogenic property in vivo: possible involvement of indirect antioxidant action. J. Ethnopharmacol. 137, 963–970. doi:10.1016/j.jep.2011.07.010
Acharoen, O., Picker, K., eutrakul, V., Taylor, W. C., and untiwachwuttikul, P. (1987). Constituents of the Zingiberaceae. X. Diastereomers of [7-Hydroxy-5-Methoxy-2-Methyl-2-(4′-Methylpent-3′-Enyl)-2H-Chromen-8-yl] [3″-Methyl-2′-(3′″-Methylbut-2″′-Enyl)]-[6″-Phenylcyclohex-3″-Enyl]M ethanone (panduratin B), a constituent of the red rhizomes of a variety of boesenbergia pandurata. Aust. J. Chem. 40, 455–459. doi:10.1071/ch9870455
Adhikari, D., Gong, D.-S., Oh, S. H., Sung, E. H., Lee, S. O., Kim, D.-W., et al. (2020). Vasorelaxant effect of Boesenbergia rotunda and its active ingredients on an isolated coronary artery. Plants 9, 1688. doi:10.3390/plants9121688
Alu’datt, M. H., Rababah, T., Alhamad, M. N., Al-Mahasneh, M. A., Almajwal, A., Gammoh, S., et al. (2016). A review of phenolic compounds in oil-bearing plants: distribution, identification and occurrence of phenolic compounds. Food Chem. 218, 99–106. doi:10.1016/j.foodchem.2016.09.057
Apinundecha, C., Teethaisong, Y., Suknasang, S., Ayamuang, I.-o., Eumkeb, G., and Vijayakumar, S. (2023). Synergistic interaction between Boesenbergia rotunda (L.) Mansf. Essential oil and cloxacillin on methicillin-resistant Staphylococcus aureus (MRSA) inhibition. Evidence-Based Complementary Altern. Med. 2023, 3453273–3453311. doi:10.1155/2023/3453273
Apinundecha, C., Teethaisong, Y., Suknasang, S., Ayamuang, I.-o., Eumkeb, G., and Vijayakumar, S. (2023). Synergistic interaction between Boesenbergia rotunda (L.) Mansf. Essential oil and cloxacillin on methicillin-resistant Staphylococcus aureus (MRSA) inhibition. Evidence-Based Complementary Altern. Med. 2023, 3453273. doi:10.1155/2023/3453273
Apiratikul, N., Tongraung, P., Dolsophon, K., Boonsri, P., and Songsrirote, K. (2023). Phytochemical from Zingiberaceae as a sustainable optical probe for heavy metal determination. Arabian J. Chem. 16, 105321. doi:10.1016/j.arabjc.2023.105321
Bahadur Gurung, A., Ajmal Ali, M., Al-Hemaid, F., El-Zaidy, M., and Lee, J. (2022). In silico analyses of major active constituents of fingerroot (Boesenbergia rotunda) unveils inhibitory activities against SARS-CoV-2 main protease enzyme. Saudi J. Biol. Sci. 29, 65–74. doi:10.1016/j.sjbs.2021.11.053
Bereswill, S., Lai, S.-L., Cheah, S.-C., Wong, P.-F., Noor, S. M., and Mustafa, M. R. (2012). In vitro and in vivo anti-angiogenic activities of panduratin A. PLoS One 7, e38103. doi:10.1371/journal.pone.0038103
Boonyarattanasoonthorn, T., Kongratanapasert, T., Jiso, A., Techapichetvanich, P., Nuengchamnong, N., Supannapan, K., et al. (2023). Absolute oral bioavailability and possible metabolic pathway of panduratin A from Boesenbergia rotunda extract in beagle dogs. Pharm. Biol. 61, 590–597. doi:10.1080/13880209.2023.2190777
Break, M. K. B., Chiang, M., Wiart, C., Chin, C.-F., Khoo, A. S. B., and Khoo, T.-J. (2020). Cytotoxic activity of Boesenbergia rotunda extracts against nasopharyngeal carcinoma cells (HK1). Cardamonin, a Boesenbergia rotunda constituent, inhibits growth and migration of HK1 cells by inducing caspase-dependent apoptosis and G2/M–phase arrest. Nutr. Cancer 73, 473–483. doi:10.1080/01635581.2020.1751217
Chatsumpun, N., Sritularak, B., and Likhitwitayawuid, K. (2017). New biflavonoids with α-glucosidase and pancreatic lipase inhibitory activities from Boesenbergia rotunda. Molecules 22, 1862. doi:10.3390/molecules22111862
Cheah, S.-C., Appleton, D. R., Lee, S.-T., Lam, M.-L., Hadi, A. H. A., and Mustafa, M. R. (2011). Panduratin A inhibits the growth of A549 cells through induction of apoptosis and inhibition of NF-KappaB translocation. Molecules 16, 2583–2598. doi:10.3390/molecules16032583
Cheah, S.-C., Lai, S.-L., Lee, S.-T., Hadi, A., and Mustafa, M. (2013). Panduratin A, a possible inhibitor in metastasized A549 cells through inhibition of NF-kappa B translocation and chemoinvasion. Molecules 18, 8764–8778. doi:10.3390/molecules18088764
Cheenpracha, S., Karalai, C., Ponglimanont, C., Subhadhirasakul, S., and Tewtrakul, S. (2006). Anti-HIV-1 protease activity of compounds from Boesenbergia pandurata. Bioorg. Med. Chem. 14, 1710–1714. doi:10.1016/j.bmc.2005.10.019
Chitapanarux, T., Lertprasertsuke, N., and Toworakul, C. (2021). Efficacy and safety of fingerroot (Boesenbergia rotunda) extract in patients with functional dyspepsia: a randomized, placebo-controlled trial. Digestion 102, 599–606. doi:10.1159/000510220
Chuah, L.-H., Wongkaewkhiaw, S., Wongrakpanich, A., Krobthong, S., Saengsawang, W., Chairoungdua, A., et al. (2022). Induction of apoptosis in human colorectal cancer cells by nanovesicles from fingerroot (Boesenbergia rotunda (L.) Mansf). Plos One 17, e0266044. doi:10.1371/journal.pone.0266044
Dhiman, A., Nanda, A., and Ahmad, S. (2016). A quest for staunch effects of flavonoids: utopian protection against hepatic ailments. Arabian J. Chem. 9, S1813–S1823. doi:10.1016/j.arabjc.2012.05.001
Feng, L., Wang, Z., Lei, Z., Zhang, X., Zhai, B., Sun, J., et al. (2024). Amomum villosum Lour.: an insight into ethnopharmacological, phytochemical, and pharmacological overview. J. Ethnopharmacol. 335, 118615. doi:10.1016/j.jep.2024.118615
Herunsalee, A., Pancharoen, O., and Tuntiwachwuttikul, P. (1987). Further studies of flavonoids of the black rhizomes Boesenbergia pandurata. J. Sci. Soc. Thail. 13, 119–122. doi:10.2306/scienceasia1513-1874.1987.13.119
Ijaz, M. U., Shahzadi, S., Hamza, A., Azmat, R., Anwar, H., Afsar, T., et al. (2023). Alleviative effects of pinostrobin against cadmium-induced renal toxicity in rats by reducing oxidative stress, apoptosis, inflammation, and mitochondrial dysfunction. Front. Nutr. 10, 1175008. doi:10.3389/fnut.2023.1175008
Isa, N. M., Abdelwahab, S. I., Mohan, S., Abdul, A. B., Sukari, M. A., Taha, M. M. E., et al. (2012). In vitro anti-inflammatory, cytotoxic and antioxidant activities of boesenbergin A, a chalcone isolated from Boesenbergia rotunda (L.) (fingerroot). Braz. J. Med. Biol. Res. 45, 524–530. doi:10.1590/s0100-879x2012007500022
Jaipetch, T., Reutrakul, V., Tuntiwachwuttikul, P., and Santisuk, T. (1982). Flavonoids in the black rhizomes of Boesenbergia panduta. Phytochemistry 22, 625–626. doi:10.1016/0031-9422(83)83075-1
Jamornwan, S., Chokpanuwat, T., Uppakara, K., Soodvilai, S., and Saengsawang, W. (2022). Anti-inflammatory activity of panduratin A against LPS-induced microglial activation. Biomedicines 10, 2587. doi:10.3390/biomedicines10102587
Jantan, I. b., Basni, I., Ahmad, A. S., Ali, N. A. M., Ahmad, A. R., and Ibrahim, H. (2001). Constituents of the rhizome oils of boesenbergia pandurata (roxb.) schlecht from Malaysia, Indonesia and Thailand. Flavour Fragr. J. 16, 110–112. doi:10.1002/ffj.956
Jitsaeng, K., Duangjit, S., Sritananuwat, P., Tansathien, K., Opanasopit, P., and Rangsimawon, W. (2023). Potential lipolytic effect of panduratin A loaded microspicule serum as a transdermal delivery approach for subcutaneous fat reduction. Biol. Pharm. Bull. 46, 1761–1768. doi:10.1248/bpb.b23-00491
Jitsaeng, K., Duangjit, S., Sritananuwat, P., Tansathien, K., Opanasopit, P., and Rangsimawong, W. (2023). Potential lipolytic effect of panduratin A loaded microspicule serum as a transdermal delivery approach for subcutaneous fat reduction. Biol. Pharm. Bull. 46, 1761–1768. doi:10.1248/bpb.b23-00491
Kabir, S. R., Dai, Z., Nurujjaman, M., Cui, X., Asaduzzaman, A. K. M., Sun, B., et al. (2020). Biogenic silver/silver chloride nanoparticles inhibit human glioblastoma stem cells growth in vitro and Ehrlich ascites carcinoma cell growth in vivo. J. Cell. Mol. Med. 24, 13223–13234. doi:10.1111/jcmm.15934
Kaewjai, C., Tonsomboon, A., Pawiwongchai, J., and Prommano, O. (2023). Antiprotozoal activity of Boesenbergia rotunda (L.) Mansf and ganoderma lucidum (Fr.) kart extracts against Blastocystis hominis. Veterinary World 16, 187–193. doi:10.14202/vetworld.2023.187-193
Kanchanapiboon, J., Kongsa, U., Pattamadilok, D., Kamponchaidet, S., Wachisunthon, D., Poonsatha, S., et al. (2020). Boesenbergia rotunda extract inhibits Candida albicans biofilm formation by pinostrobin and pinocembrin. J. Ethnopharmacol. 261, 113193. doi:10.1016/j.jep.2020.113193
Kanjanasirirat, P., Suksatu, A., Manopwisedjaroen, S., Munyoo, B., Tuchinda, P., Jearawuttanakul, K., et al. (2020). High-content screening of Thai medicinal plants reveals Boesenbergia rotunda extract and its component Panduratin A as anti-SARS-CoV-2 agents. Sci. Rep. 10, 19963. doi:10.1038/s41598-020-77003-3
Kittakoop, P., Darshana, D., Sangsuwan, R., and Mahidol, C. (2022). Alkaloids and alkaloid-like compounds are potential scaffolds of antiviral agents against SARS-CoV-2 (COVID-19) virus. Heterocycles 105, 115. doi:10.3987/rev-22-sr(r)3
Kongratanapasert, T., Kongsomros, S., Arya, N., Sutummaporn, K., Wiriyarat, W., Akkhawattanangkul, Y., et al. (2023). Pharmacological activities of fingerroot extract and its phytoconstituents against SARS-CoV-2 infection in golden Syrian hamsters. J. Exp. Pharmacol. 15, 13–26. doi:10.2147/jep.S382895
Kongsui, R., Promsrisuk, T., Klimaschewski, L., Sriraksa, N., Jittiwat, J., and Thongrong, S. (2023). Pinostrobin mitigates neurodegeneration through an up-regulation of antioxidants and GDNF in a rat model of Parkinson’s disease. F1000Research 12, 846. doi:10.12688/f1000research.134891.2
Kongsui, R., Surapinit, S., Promsrisuk, T., and Thongrong, S. (2023). Pinostrobin from Boesenbergia rotunda attenuates oxidative stress and promotes functional recovery in rat model of sciatic nerve crush injury. Braz. J. Med. Biol. Res. 56, e12578. doi:10.1590/1414-431x2023e12578
Kongwattanakul, S., Petchann, N., Petroch, P., Thanthong, S., Tungfung, S., Chamchod, S., et al. (2022). Prophylactic management of radiation-induced mucositis using herbal mouthwash in patients with head and neck cancer: an assessor-blinded randomized controlled trial. J. Complementary Integr. Med. 19, 771–780. doi:10.1515/jcim-2021-0457
Lai, S.-L., Wong, P.-F., Lim, T.-K., Lin, Q., and Mustafa, M. R. (2015). Cytotoxic mechanisms of panduratin A on A375 melanoma cells: a quantitative and temporal proteomics analysis. Proteomics 15, 1608–1621. doi:10.1002/pmic.201400039
Li, C., Chen, S.-C., Chen, Y., Girish, S., Kaagedal, M., Lu, D., et al. (2020). Impact of physiologically based pharmacokinetics, population pharmacokinetics and pharmacokinetics/pharmacodynamics in the development of antibody-drug conjugates. J. Clin. Pharmacol. 60 Suppl 1, S105-S119. doi:10.1002/jcph.1720
Liana, D., Eurtivong, C., and Phanumartwiwath, A. (2024). Boesenbergia rotunda and its pinostrobin for atopic dermatitis: dual 5-lipoxygenase and cyclooxygenase-2 inhibitor and its mechanistic study through steady-state kinetics and molecular modeling. Antioxidants 13, 74. doi:10.3390/antiox13010074
Liew, Y. J. M., Chua, K.-O., Yong, H.-S., Song, S.-L., and Chan, K.-G. (2022). Complete chloroplast genome of Boesenbergia rotunda and a comparative analysis with members of the family Zingiberaceae. Braz. J. Bot. 45, 1209–1222. doi:10.1007/s40415-022-00845-w
Ling, J., Mohamed, M., Rahmat, A., and Bakar, M. F. A. (2010). Phytochemicals, antioxidant properties and anticancer investigations of the different parts of several gingers species (Boesenbergia rotunda, Boesenbergia pulchella var attenuata and Boesenbergia armeniaca). J. Med. Plants Res. 4, 27–32. doi:10.5897/JMPR09.308
Liu, Y., Ma, X., Liang, H., Stephanopoulos, G., and Zhou, K. (2021). Monoterpenoid biosynthesis by engineered microbes. J. Industrial Microbiol. Biotechnol. 48, kuab065. doi:10.1093/jimb/kuab065
Md-Mustafa, N. D., Khalid, N., Gao, H., Peng, Z., Alimin, M. F., Bujang, N., et al. (2014). Transcriptome profiling shows gene regulation patterns in a flavonoid pathway in response to exogenous phenylalanine in Boesenbergia rotunda cell culture. BMC Genomics 15, 984–1025. doi:10.1186/1471-2164-15-984
Mohan, S., Hobani, Y. H., Shaheen, E., Abou-Elhamd, A. S., abdelhaleem, A., Alhazmi, H. A., et al. (2020). Ameliorative effect of Boesenbergin A, a chalcone isolated from Boesenbergia rotunda (Fingerroot) on oxidative stress and inflammation in ethanol-induced gastric ulcer in vivo. J. Ethnopharmacol. 261, 113104. doi:10.1016/j.jep.2020.113104
Morikawa, T., Funakoshi, K., Ninomiya, K., Yasuda, D., Miyagawa, K., Matsuda, H., et al. (2008). Medicinal foodstuffs. XXXIV. Structures of new prenylchalcones and prenylflavanones with TNF-alpha and aminopeptidase N inhibitory activities from Boesenbergia rotunda. Chem. Pharm. Bull. 56, 956–962. doi:10.1248/cpb.56.956
Murti, Y., Pathak, D., and Pathak, K. (2021). Green chemistry approaches to the synthesis of flavonoids. Curr. Org. Chem. 25, 2005–2027. doi:10.2174/1385272825666210728095624
Narendar Reddy, T., Beatriz, A., Jayathirtha Rao, V., and de Lima, D. P. (2019). Carbonyl compounds′ journey to amide bond formation. Chem. - Asian J. 14, 344–388. doi:10.1002/asia.201801560
Ng, K.-B., Bustamam, A., Sukari, M. A., Abdelwahab, S. I., Mohan, S., Buckle, M. J. C., et al. (2013). Induction of selective cytotoxicity and apoptosis in human T4-lymphoblastoid cell line (CEMss) by boesenbergin a isolated from Boesenbergia rotunda rhizomes involves mitochondrial pathway, activation of caspase 3 and G2/M phase cell cycle arrest. BMC Complementary Altern. Med. 13, 41. doi:10.1186/1472-6882-13-41
Nguyen, M. T. T., Nguyen, H. X., Dang, P. H., Le, T. H., Do, T. N. V., Omar, A. M., et al. (2021). Panduratins Q–Y, dimeric metabolites from Boesenbergia rotunda and their antiausterity activities against the PANC-1 human pancreatic cancer cell line. Phytochemistry 183, 112646. doi:10.1016/j.phytochem.2020.112646
Nishidono, Y., Fujita, T., Kawanami, A., Nishizawa, M., and Tanaka, K. (2017). Identification of PGC-1α activating constituents in Zingiberaceous crude drugs. Fitoterapia 122, 40–44. doi:10.1016/j.fitote.2017.08.007
Olatunji, O. J., Zhang, Q., Shu, S., Liu, C., and Wang, T. (2022). Therapeutic efficacy of polyphenol-rich fraction of boesenbergia rotunda in diabetic rats: a focus on hypoglycemic, antihyperlipidemic, carbohydrate metabolism, antioxidant, anti-inflammatory and pancreato-protective activities. Front. Bioscience-Landmark 27, 206. doi:10.31083/j.fbl2707206
Ping, C. P., Tengku Mohamad, T. A. S., Akhtar, M. N., Perimal, E. K., Akira, A., Israf Ali, D. A., et al. (2018). Antinociceptive effects of Cardamonin in mice: possible involvement of TRPV₁, glutamate, and opioid receptors. Molecules 23, 2237. doi:10.3390/molecules23092237
Potipiranun, T., Adisakwattana, S., Worawalai, W., Ramadhan, R., and Phuwapraisirisan, P. (2018). Identification of pinocembrin as an anti-glycation agent and α-glucosidase inhibitor from fingerroot (Boesenbergia rotunda): the tentative structure–activity relationship towards MG-trapping activity. Molecules 23, 3365. doi:10.3390/molecules23123365
Pui Ping, C., Akhtar, M. N., Israf, D. A., Perimal, E. K., and Sulaiman, M. R. (2020). Possible participation of ionotropic glutamate receptors and l-arginine-nitric oxide-cyclic guanosine monophosphate-ATP-sensitive K+ channel pathway in the antinociceptive activity of Cardamonin in acute pain animal models. Molecules 25, 5385. doi:10.3390/molecules25225385
Rosdianto, A. M., Puspitasar, I. M., Lesmana, R., and Levita, J. (2020). Determination of Quercetin and flavonol synthase in Boesenbergia rotunda rhizome. Pak. J. Biol. Sci. 23, 264–270. doi:10.3923/pjbs.2020.264.270
Rosdianto, A. M., Puspitasari, I. M., Lesmana, R., Levita, J., and Tan, G. T. (2020). Inhibitory activity of Boesenbergia rotunda (L.) Mansf. Rhizome towards the expression of Akt and NF-KappaB p65 in acetic acid-induced wistar rats. Evidence-Based Complementary Altern. Med. 2020, 6940313. doi:10.1155/2020/6940313
Rungsa, P., San, H. T., Sritularak, B., Böttcher, C., Prompetchara, E., Chaotham, C., et al. (2023). Inhibitory effect of isopanduratin A on adipogenesis: a study of possible mechanisms. Foods 12, 1014. doi:10.3390/foods12051014
Ruttanapattanakul, J., Wikan, N., Okonogi, S., Na Takuathung, M., Buacheen, P., Pitchakarn, P., et al. (2021). Boesenbergia rotunda extract accelerates human keratinocyte proliferation through activating ERK1/2 and PI3K/Akt kinases. Biomed. and Pharmacother. 133, 111002. doi:10.1016/j.biopha.2020.111002
Ruttanapattanakul, J., Wikan, N., Potikanond, S., and Nimlamool, W. (2022). Molecular targets of pinocembrin underlying its regenerative activities in human keratinocytes. Pharmaceuticals 15, 954. doi:10.3390/ph15080954
Saah, S., Siriwan, D., Trisonthi, P., and Dueramae, S. (2024). Physicochemical and biological properties of encapsulated Boesenbergia rotunda extract with different wall materials in enhancing antioxidant, mineralogenic and osteogenic activities of MC3T3-E1 cells. Saudi Pharm. J. 32, 101998. doi:10.1016/j.jsps.2024.101998
Salama, S. M., Abdulla, M. A., AlRashdi, A. S., and Hadi, A. H. A. (2013). Mechanism of hepatoprotective effect of Boesenbergia rotunda in thioacetamide-induced liver damage in rats. Evidence-Based Complementary Altern. Med. 2013, 157456–157513. doi:10.1155/2013/157456
Salama, S. M., AlRashdi, A. S., Abdulla, M. A., Hassandarvish, P., and Bilgen, M. (2013). Protective activity of Panduratin A against thioacetamide-induced oxidative damage demonstration with in vitro experiments using WRL-68 liver cell line. BMC Complement. Altern. Med. 24, 279. doi:10.1186/1472-6882-13-279
Salama, S. M., Bilgen, M., Al Rashdi, A. S., and Abdulla, M. A. (2012). Efficacy of Boesenbergia rotunda treatment against thioacetamide-induced liver cirrhosis in a rat model. Evidence-Based Complementary Altern. Med. 2012, 137083–137112. doi:10.1155/2012/137083
Salama, S. M., Ibrahim, I. A. A., Shahzad, N., Al-Ghamdi, S., Ayoub, N., AlRashdi, A. S., et al. (2018). Hepatoprotectivity of Panduratin A against liver damage: in vivo demonstration with a rat model of cirrhosis induced by thioacetamide. Apmis 126, 710–721. doi:10.1111/apm.12878
San, H. T., Khine, H. E. E., Sritularak, B., Prompetchara, E., Chaotham, C., Che, C.-T., et al. (2022). Pinostrobin: an adipogenic suppressor from fingerroot (Boesenbergia rotunda) and its possible mechanisms. Foods 11, 3024. doi:10.3390/foods11193024
Sujana, D., Sumiwi, S., Saptarini, N., and Levita, J. (2024). The nephroprotective activity of Boesenbergia rotunda rhizome by reducing creatinine, urea nitrogen, glutamic pyruvic transaminase, and malondialdehyde levels in the blood and attenuating the expression of Havcr1 (KIM-1), Lcn2 (NGAL), Casp3, and Casp7 genes in the kidney cortex of cisplatin-induced sprague-dawley rats. J. Exp. Pharmacol. 16, 189–200. doi:10.2147/jep.S459483
Sukari, M. A., Sharif, N. W. M., Yap, A. L. C., Tang, S. W., Neoh, B. K., Rahmani, M., et al. (2008). Chemical constituents variations of essential oils from rhizomes of four Zingberaceae species. Malays. J. Anal. Sci. 12, 638–644.
Suwannayod, S., Sukontason, K. L., Pitasawat, B., Junkum, A., Limsopatham, K., Jones, M. K., et al. (2019). Synergistic toxicity of plant essential oils combined with pyrethroid insecticides against blow flies and the house fly. Insects 10, 178. doi:10.3390/insects10060178
Taechowisa, T., Chuen-Im, T., and Phutdhawon, W. S. (2024). Antibacterial and anti-inflammatory properties of flavonoids from streptomyces chartreusis RH3.5. Pak. J. Biol. Sci. 27, 244–255. doi:10.3923/pjbs.2024.244.255
Taheri, S., Teo, C. H., Heslop-Harrison, J. S., Schwarzacher, T., Tan, Y. S., Wee, W. Y., et al. (2022). Genome assembly and analysis of the flavonoid and phenylpropanoid biosynthetic pathways in fingerroot ginger (Boesenbergia rotunda). Int. J. Mol. Sci. 23, 7269. doi:10.3390/ijms23137269
Tan, B. C., Tan, S. K., Wong, S. M., Ata, N., Rahman, N. A., and Khalid, N. (2015). Distribution of flavonoids and cyclohexenyl chalcone derivatives in conventional propagated andin vitro-derived field-Grown Boesenbergia rotunda (L.) Mansf. Evidence-Based Complementary Altern. Med. 2015, 451870–451877. doi:10.1155/2015/451870
Techapichetvanich, P., Tangpanithandee, S., Supannapan, K., Wongwiwatthananukit, S., Chang, L. C., and Khemawoot, P. (2024). Oral sub-chronic toxicity of fingerroot (Boesenbergia rotunda) rhizome extract formulation in Wistar rats. Toxicol. Rep. 12, 224–233. doi:10.1016/j.toxrep.2024.01.013
Teethaisong, Y., Pimchan, T., Srisawat, R., Hobbs, G., and Eumkeb, G. (2018). Boesenbergia rotunda (L.) Mansf. extract potentiates the antibacterial activity of some β-lactams against β-lactam-resistant staphylococci. J. Glob. Antimicrob. Resist. 12, 207–213. doi:10.1016/j.jgar.2017.10.019
Thadtapong, N., Chaturongakul, S., Napaswad, C., Dubbs, P., and Soodvilai, S. (2024). Enhancing effect of natural adjuvant, panduratin A, on antibacterial activity of colistin against multidrug-resistant Acinetobacter baumannii. Sci. Rep. 14, 9863. doi:10.1038/s41598-024-60627-0
Thongnuanjan, P., Soodvilai, S., Fongsupa, S., Chabang, N., Vivithanaporn, P., Tuchinda, P., et al. (2021). Protective effect of panduratin A on cisplatin-induced apoptosis of human renal proximal tubular cells and acute kidney injury in mice. Biol. Pharm. Bull. 44 (6), 830–837. doi:10.1248/bpb.b21-00036
Thongrong, S., Surapinit, S., Promsrisuk, T., Jittiwat, J., and Kongsui, R. (2023). Pinostrobin alleviates chronic restraint stress-induced cognitive impairment by modulating oxidative stress and the function of astrocytes in the hippocampus of rats. Biomed. Rep. 18, 20. doi:10.3892/br.2023.1602
Tuchindaa, P., Reutrakula, V., Claesona, P., Pongprayoonb, U., Sematongb, T., Santisukc, T., et al. (2002). Phytochem-Anti-inflammatory cyclohexenyl chalcone derivatives in Boesenbergia pandurata. Phytochemistry 59, 169–173. doi:10.1016/s0031-9422(01)00451-4
Tunnisa, F., Nur Faridah, D., Afriyanti, A., Rosalina, D., Ana Syabana, M., Darmawan, N., et al. (2022). Antioxidant and antidiabetic compounds identification in several Indonesian underutilized Zingiberaceae spices using SPME-GC/MS-based volatilomics and in silico methods. Food Chem. X 14, 100285. doi:10.1016/j.fochx.2022.100285
Tuntiwachwuttik, P., ancharoen, O., Reutrakul, V., and Byrne, L. T. (1984). (1'RS,2'SR,6'RS)-(2,6-Dihydroxy-4-methoxyphenyl)-[3'-methyl-2'-(3''-methylbut-2''-enyl)-6'-phenyl-cyclohex-3'-enyl]methanone (panduratin A)-a Constituent of the Red Rhizomers of a variety of Boesenbergia pandurata. Aust. J. Chem. 37, 449–453. doi:10.1071/ch9840449
Vergoten, G., and Bailly, C. (2022). Interaction of panduratin A and derivatives with the SARS-CoV-2 main protease (mpro): a molecular docking study. J. Biomol. Struct. Dyn. 41, 6834–6844. doi:10.1080/07391102.2022.2112618
Voon, F.-L., Sulaiman, M. R., Akhtar, M. N., Idris, M. F., Akira, A., Perimal, E. K., et al. (2017). Cardamonin (2′,4′-dihydroxy-6′-methoxychalcone) isolated from Boesenbergia rotunda (L.) Mansf. inhibits CFA-induced rheumatoid arthritis in rats. Eur. J. Pharmacol. 794, 127–134. doi:10.1016/j.ejphar.2016.11.009
Wang, P., Wen, C., and Olatunji, O. J. (2022). Anti-inflammatory and antinociceptive effects of Boesenbergia rotunda polyphenol extract in diabetic peripheral neuropathic rats. J. Pain Res. 15, 779–788. doi:10.2147/jpr.S359766
Wang, Z., Shi, Y., Zhang, X., Sun, J., Guo, D., Luan, F., et al. (2024). Research progress in the ethnopharmacology, phytochemistry, pharmacology, toxicology, and quality control of Valeriana jatamansi Jones. J. Ethnopharmacol. 332, 118403. doi:10.1016/j.jep.2024.118403
Widyananda, M. H., Wicaksono, S. T., Rahmawati, K., Puspitarini, S., Ulfa, S. M., Jatmiko, Y. D., et al. (2022). A potential anticancer mechanism of finger root (Boesenbergia rotunda) extracts against a breast cancer cell line. Scientifica 2022, 9130252–9130317. doi:10.1155/2022/9130252
Win, N. N., Awale, S., Esumi, H., Tezuka, Y., and Kadota, S. (2007). Bioactive secondary metabolites from boesenbergia pandurata of Myanmar and their preferential cytotoxicity against human pancreatic cancer PANC-1 cell line in nutrient-deprived medium. J. Nat. Prod. 70, 1582–1587. doi:10.1021/np070286m
Win, N. N., Awale, S., Esumi, H., Tezuka, Y., and Kadota, S. (2008). Panduratins D—I, novel secondary metabolites from rhizomes of boesenbergia pandurata. Chem. and Pharm. Bull. 56, 491–496. doi:10.1248/cpb.56.491
Win, N. N., Kyaw, M. M., Prema, , Ngwe, H., Ito, T., Asakawa, Y., et al. (2019). Dinorcassane diterpenoid from Boesenbergia rotunda rhizomes collected in lower Myanmar. Chem. and Biodivers. 16, e1800657. doi:10.1002/cbdv.201800657
Worakajit, N., Thipboonchoo, N., Chaturongakul, S., Jutabha, P., Soontornniyomkij, V., Tuchinda, P., et al. (2022). Nephroprotective potential of Panduratin A against colistin-induced renal injury via attenuating mitochondrial dysfunction and cell apoptosis. Biomed. Pharmacother. 148, 112732. doi:10.1016/j.biopha.2022.112732
Wu, X., Zhang, H., Fan, S., Zhang, Y., Yang, Z., Fan, S., et al. (2018). Quality markers based on biological activity: a new strategy for the quality control of traditional Chinese medicine. Phytomedicine 44, 103–108. doi:10.1016/j.phymed.2018.01.016
Yan, Y., Zou, M., Tang, C., Ao, H., He, L., Qiu, S., et al. (2024). The insights into sour flavor and organic acids in alcoholic beverages. Food Chem. 460, 140676. doi:10.1016/j.foodchem.2024.140676
Yuliana, N. D., Budijanto, S., Verpoorte, R., and Choi, Y. H. (2013). NMR metabolomics for identification of adenosine A1 receptor binding compounds from Boesenbergia rotunda rhizomes extract. J. Ethnopharmacol. 150, 95–99. doi:10.1016/j.jep.2013.08.012
Yushinta, F., Andi Aliah, H., Dwi Kesuma, S., Siti, A., Nita, R., Abdul, M., et al. (2023). The optimal dosage of fermented herbal extract on growth and feed efficiency of nile Tilapia (Oreochromis niloticus). Trop. Life Sci. Res. 34, 39–56. doi:10.21315/tlsr2023.34.2.3
Zhang, L., Jiang, Q., Wang, X., Jaisi, A., and Olatunji, O. J. (2023). Boesenbergia rotunda displayed anti-inflammatory, antioxidant and anti-apoptotic efficacy in doxorubicin-induced cardiotoxicity in rats. Sci. Rep. 13, 11398. doi:10.1038/s41598-023-38560-5
ALP alkaline phosphatase
ALT alanine aminotransferase
AMPK AMP-activated protein kinase
AST aspartate aminotransferase
AUC area under the curve
Bax bcl-associated X protein
Bcl-2 B-cell lymphoma-2
Bcl-XL B-cell lymphoma-extra large
BNP brain natriuretic peptide
Caspase cysteinyl aspartate specific proteinase
CAT catalase
C/EBPα CCAAT/enhancer-binding protein α
CDK 4 cyclin-dependent kinase 4
CNKI China National Knowledge Infrastructure
COX-1 cyclooxygenase-1
COX-2 cyclooxygenase-2
CK-MB creatine kinase MB
CPT Carnitine palmitoyl transferase
CRC colorectal cancer
cTnI cardiac troponin I
DPPH 2,2-Diphenyl-1-picrylhydrazyl
DRG dorsal root ganglion
EAAT2 excitatory amino acid transporter 2
FAS fatty acid synthase
FBG fasting blood glucose
FFA free fatty acids
GFAP glial fibrillary acidic protein
GPx glutathione peroxidase
GSH reduced glutathione
HDL high-density lipoprotein
HO-1 heme oxygenase-1
HSC hepatic stellate cells
IL-1β interleukin-1β
IL-4 interleukin-4
IL-10 interleukin-10
IL-6 interleukin-6
IL-8 interleukin-8
iNOS inducible NO synthase
JAK 3 janus kinase 3
KCNA 3 potassium voltage-gated channel subfamily A member 3
KIM-1 kidney injury molecule 1
LDH lactate dehydrogenase
LDL low-density lipoprotein
LDLC low-density lipoprotein cholesterol
LDLR low density lipoprotein receptor
MAPK mitogen-activated protein kinase
MDA malondialdehyde
MMP 12 matrix metallopeptidase 12
MMP 13 matrix metallopeptidase 13
MPO myeloperoxidase
MRSA methicillin resistant Staphylococcus aureus
NGAL neutrophil gelatinase-associated lipocalin
NF-κB nuclear factor-κB
NO nitric oxide
nNOS neuronal nitric oxide synthase
Nrf2 nuclear factor erythroid 2-related factor
PARP poly ADP-ribose polymerase
PBG Post-prandia blood glucose
PGE2 prostaglandin E2
PPAR-α peroxisome proliferator activated receptors-α
PPAR-γ peroxisome proliferator-activated receptor-γ
PKC protein kinase C
p-Akt phosphorylated protein kinase B
p-JNK phosphorylated c-jun N-terminal kinase
p-p38 phosphorylated p38 mitogen-activated protein kinase
RANKL Receptor activator of nuclear factor kappa-β ligand
RCT reverse cholesterol transport
SOD superoxide dismutase
SREBP-1c sterol regulatory element binding protein-1c
STZ streptozotocin
TAA thioacetamide
T-AOC total anti-oxidant capacity
TC total cholesterol
TG triglycerides
TGF-β1 transforming growth factor beta 1
Tim-1 T cell immunoglobulin domain and mucin domain protein-1
TNF-α tumor necrosis factor-α
TRAP tartrate-resistant acid phosphatase
VEGFR 2 vascular endothelial growth factor receptor 2
VEGFR 1 vascular endothelial growth factor receptor 1
VLDLC very low-density lipoprotein cholesterol
Keywords: Boesenbergia rotunda (L.) Mansf, traditional usages, flavonoids, anti-cancer activity, quality control, toxicology, clinical settings
Citation: Wang Y, Wen J, Liu F, Peng X, Xu G, Zhang M and Huang Z (2025) Traditional usages, chemical metabolites, pharmacological activities, and pharmacokinetics of Boesenbergia rotunda (L.) Mansf.: a comprehensive review. Front. Pharmacol. 16:1527210. doi: 10.3389/fphar.2025.1527210
Received: 13 November 2024; Accepted: 21 February 2025;
Published: 19 March 2025.
Edited by:
Irina Ielciu, University of Medicine and Pharmacy Iuliu Hatieganu, RomaniaReviewed by:
Laiba Arshad, Forman Christian College, PakistanCopyright © 2025 Wang, Wen, Liu, Peng, Xu, Zhang and Huang. This is an open-access article distributed under the terms of the Creative Commons Attribution License (CC BY). The use, distribution or reproduction in other forums is permitted, provided the original author(s) and the copyright owner(s) are credited and that the original publication in this journal is cited, in accordance with accepted academic practice. No use, distribution or reproduction is permitted which does not comply with these terms.
*Correspondence: Zhuangzhuang Huang, aHVhbmdzdHJvbmdAMTYzLmNvbQ==; Mingliang Zhang, bWx6aGFuZ2VkdUAxMjYuY29t
Disclaimer: All claims expressed in this article are solely those of the authors and do not necessarily represent those of their affiliated organizations, or those of the publisher, the editors and the reviewers. Any product that may be evaluated in this article or claim that may be made by its manufacturer is not guaranteed or endorsed by the publisher.
Research integrity at Frontiers
Learn more about the work of our research integrity team to safeguard the quality of each article we publish.