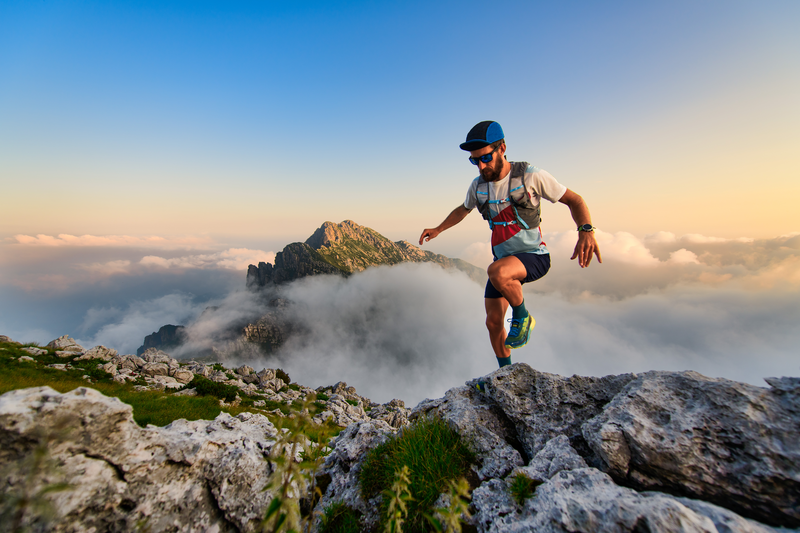
94% of researchers rate our articles as excellent or good
Learn more about the work of our research integrity team to safeguard the quality of each article we publish.
Find out more
ORIGINAL RESEARCH article
Front. Pharmacol.
Sec. Ethnopharmacology
Volume 16 - 2025 | doi: 10.3389/fphar.2025.1526274
This article is part of the Research Topic Exploring Herbal Innovations: New Frontiers in Functional Foods and Nutraceuticals Development View all articles
The final, formatted version of the article will be published soon.
You have multiple emails registered with Frontiers:
Please enter your email address:
If you already have an account, please login
You don't have a Frontiers account ? You can register here
Bletilla striata polysaccharide was extracted from natural medicinal plant Bletilla Striata. Bletilla Oligosaccharides (BO) were obtained by acid hydrolysis of polysaccharide. The structure of BO were characterised by HPGPC, GC-MS and NMR. The effect of BO on intestinal mucositis in mice was detected by assessing the levels of intestinal flora, ZO-1, occludin, and MUC-2, and inflammatory cytokines (IL-1β, IL-6, IL-10, and TNF-). Structural characterisation showed that BO were the neutral polysaccharide composed mainly of glucose and mannose. The backbone of BO consisted of →4)--Manp-(1→, →4)--Glcp-(1→ and small →3,4)--Manp-(1→. In vivo, BO reduced the concentrations of pro-inflammatory factors such as IL-1, IL-6 and TNF-, prevented the disruption of tight junctions ZO-1, occludin and improved the gut microbial community. Therefore, BO can relieve the progress of intestinal mucositis by relieving inflammation, protecting the intestinal epithelial barrier and regulating the intestinal microbiota. These data provide experimental evidence for the application of BO in chemotherapeutic intestinal mucositis.
Keywords: Bletilla oligosaccharides, Chemotherapeutic intestinal mucositis, Inflammation, intestinal flora, NF-kB
Received: 11 Nov 2024; Accepted: 12 Feb 2025.
Copyright: © 2025 Yin, li, xiong, jiang, ma and Qian. This is an open-access article distributed under the terms of the Creative Commons Attribution License (CC BY). The use, distribution or reproduction in other forums is permitted, provided the original author(s) or licensor are credited and that the original publication in this journal is cited, in accordance with accepted academic practice. No use, distribution or reproduction is permitted which does not comply with these terms.
* Correspondence:
Guoqiang Qian, Guangdong Pharmaceutical University, Guangzhou, China
Disclaimer: All claims expressed in this article are solely those of the authors and do not necessarily represent those of their affiliated organizations, or those of the publisher, the editors and the reviewers. Any product that may be evaluated in this article or claim that may be made by its manufacturer is not guaranteed or endorsed by the publisher.
Research integrity at Frontiers
Learn more about the work of our research integrity team to safeguard the quality of each article we publish.