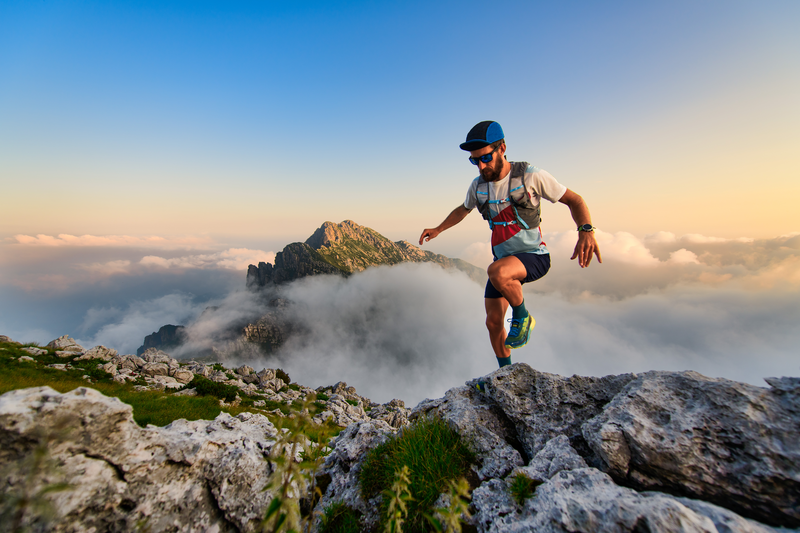
94% of researchers rate our articles as excellent or good
Learn more about the work of our research integrity team to safeguard the quality of each article we publish.
Find out more
REVIEW article
Front. Pharmacol.
Sec. Ethnopharmacology
Volume 16 - 2025 | doi: 10.3389/fphar.2025.1524505
The final, formatted version of the article will be published soon.
You have multiple emails registered with Frontiers:
Please enter your email address:
If you already have an account, please login
You don't have a Frontiers account ? You can register here
This systematic review and meta-analysis aims to assess the efficacy of GBE in the treatment of IPF by evaluating its impact on total effective rate, blood gas analysis, pulmonary function tests, and markers of inflammation and fibrosis. Methods: We conducted a comprehensive search across seven databases, including PubMed, EMBASE, Web of Science, CNKI, Wanfang DATA, VIP, and CBM, without restrictions on publication date. Randomized controlled trials (RCTs) that investigated the effects of GBE on IPF patients were eligible for inclusion. Relevant literature was screened, and the data in the included studies were extracted for quality assessment according to the Risk of bias tool. Results: A total of 14 RCTs involving 1043 patients were included in the analysis. GBE significantly improved the total effective rate, arterial oxygen partial pressure, arterial oxygen saturation, forced vital capacity, forced expiratory volume in one second, maximum voluntary ventilation, and sixminute walk test compared to the control group. Additionally, there was a significant reduction in arterial carbon dioxide partial pressure, interleukin-4, hyaluronan, and laminin levels. Conclusion: GBE may offer therapeutic benefits in IPF by improving respiratory function, modulating inflammation, and affecting fibrosis markers. These findings support the potential use of GBE as an adjunct therapy in IPF and suggest that further large-scale, multicenter trials are warranted to confirm its efficacy and safety. 1 Introduction Idiopathic Pulmonary Fibrosis (IPF) is a devastating interstitial lung disease characterized by the progressive replacement of functional lung tissue with fibrotic scar tissue, leading to impaired gas exchange and ultimately respiratory failure (1). The etiology of IPF remains obscure, and despite recent advances in understanding its pathogenesis, the disease continues to carry a poor prognosis, with a median survival time of only three to five years following diagnosis (2). The complexity of IPF's pathogenesis, which encompasses chronic inflammation, dysregulated wound healing, and microenvironmental changes that drive fibroblast proliferation and extracellular matrix deposition, poses significant challenges for disease management(3).
Keywords: Ginkgo biloba extract, Idiopathic Pulmonary Fibrosis, Meta-analysis, Systematic review, RCT - randomized controlled trial
Received: 07 Nov 2024; Accepted: 17 Feb 2025.
Copyright: © 2025 Sun, Peng, Xiao, Li and Chen. This is an open-access article distributed under the terms of the Creative Commons Attribution License (CC BY). The use, distribution or reproduction in other forums is permitted, provided the original author(s) or licensor are credited and that the original publication in this journal is cited, in accordance with accepted academic practice. No use, distribution or reproduction is permitted which does not comply with these terms.
* Correspondence:
Sheng Chen, Shenzhen Traditional Chinese Medicine Hospital, Shenzhen ,Guangdong, China
Disclaimer: All claims expressed in this article are solely those of the authors and do not necessarily represent those of their affiliated organizations, or those of the publisher, the editors and the reviewers. Any product that may be evaluated in this article or claim that may be made by its manufacturer is not guaranteed or endorsed by the publisher.
Research integrity at Frontiers
Learn more about the work of our research integrity team to safeguard the quality of each article we publish.